Abstract
Soil solarization is an ecologically friendly method of controlling various plant pathogens and pests, but also affects non-pathogenic members of the soil biota. Here, we studied the impact of soil solarization on the community structure of soil ciliates using a culture-independent molecular approach, namely denaturing gradient gel electrophoresis (DGGE) of targeted 18S rRNA gene fragments. Greenhouse soil with added organic fertilizers was solarized for 33 days at an average temperature of 47–48°C. Solarization caused a drastic change in the ciliate community. The variation between replicates was large, which suggested that the distribution of ciliates was spatially heterogeneous in the soil, probably due to their decreased numbers. In contrast, non-solarized soil had a stable and homogeneous ciliate community during the experimental period. In solarized soil, most of the original ciliate community recovered 76 days after solarization. Sequence analysis of DGGE fragments indicated that both r-selected and K-selected species of ciliates were affected by solarization but recovered with time after solarization. Our results demonstrated both the vulnerability and resilience of the ciliate community to soil solarization and also the utility of using molecular-based analysis of ciliate communities as bioindicators of soil stress caused by solarization.
INTRODUCTION
Soil solarization (solar heating) was developed as an alternative method of soil disinfection in place of steaming or fumigation (Katan et al. Citation1976). The technique involves covering moist soil with a transparent plastic sheet during the warmest time of the year to achieve a soil temperature above the tolerance of many mesophilic organisms, including pathogenic microbes and weeds. The pesticidal efficacy of solarization is often complemented by amendment with organic matter (Stapleton Citation2000; Schönfeld et al. Citation2003; Simmons et al. Citation2013). The effectiveness of soil solarization against many pathogens, weeds and soil arthropods in the cultivation of various crops has been well demonstrated in both open fields and greenhouses (Stapleton Citation2000). This environmentally friendly method of soil disinfection has become prevalent since the use of methyl bromide, the most effective soil fumigant, has been virtually phased out to protect the stratospheric ozone layer.
Soil solarization controls a broad spectrum of pathogenic fungi, nematodes, weeds and other pests, but it also affects non-pathogenic microorganisms (Schönfeld et al. Citation2003; Culman et al. Citation2006; Simmons et al. Citation2014; Yokoe et al. Citation2015), and the nutrient release from dead microbial cells after solarization may cause a long-term increased growth response of crops (Chen et al. Citation1991). During soil solarization, high temperature, limited oxygen (O2) and organic enrichment alter the bacterial community structure in soil, leading to a higher dominance of thermotolerant and anaerobic species (Schönfeld et al. Citation2003; Simmons et al. Citation2014). The stability of an altered bacterial community structure caused by solarization in an agricultural soil in Italy was enhanced by organic enrichment with farmyard manure (Gelsomino and Cacco Citation2006). In contrast to the long-term effect of soil solarization on the bacterial community, the period of the impact on the fungal community in soil with a rice–wheat cropping system is shorter (Culman et al. Citation2006).
Ciliates (or Ciliophora) are ubiquitous microeukaryotic organisms found in nearly all types of soil environments. The species richness of ciliates in agroecosystems is often comparable with that in natural biotopes (Foissner Citation1997). Ciliates play an important role in soil ecosystems as predators of bacteria, fungi and other protists. Their community composition summarizes the state of all other soil communities, as different species have different prey ranges. They are therefore excellent bioindicators for the whole system. Grazing activity of ciliates enhances nutrient flow in soil and thus contributes to increased nutrient uptake by plants (Griffiths Citation1986). In addition, the dynamics and community structure of ciliates have been used as bioindicators of changes in the soil environment, such as moisture and temperature, and of human impact on soil including organic pollution, pesticide treatment and soil fumigation (Foissner Citation1987; Yeates et al. Citation1991; Foissner Citation1999). The moisture content is a primary controlling factor of ciliate grazing activity (Vargas and Hattori Citation1986), and the size of water-filled pores in soil can shape the ciliate community according to their body size (Fenchel Citation1987). Ciliates are used as bioindicators of the saprobic system in aquatic environments (Foissner and Berger Citation1996). In a rice field soil, long-term application of compost causes an increased predominance of ciliates in the eukaryotic community (Murase et al. Citation2015). The species richness and abundance of ciliates in savannah soil, including ciliates that grow at high temperature (up to 40°C), is increased by fire and deforestation (Foissner Citation1987). On the other hand, soil steaming greatly reduces the number of ciliate species in greenhouse soil even after 100 days of steaming, but favors the growth of the surviving ciliate species (Stout Citation1955). Therefore, the changes to the soil environment caused by soil solarization, i.e., high temperature, increased moisture and organic enrichment, may greatly affect the ciliate community. To the best of our knowledge, however, no study on the effect of solarization on soil biota has focused on any protists.
Ciliates are a monophyletic group of protists, which allows us to use a molecular approach based on the SSU rRNA gene to analyze the community structure of soil ciliates (Lara et al. Citation2007; Jousset et al. Citation2010; Shimano et al. Citation2012). One of the greatest advantages of studying ciliates is that some high-level clades (i.e., orders and classes) are characterized by common metabolic and life-history features (Lara and Acosta-Mercado Citation2012), which rationalizes the use of molecular-based information of the ciliate communities as soil bioindicators. Here, we studied the impact of soil solarization on the ciliate community in a greenhouse soil in which tomatoes (Solanum lycopersicum) were later cultivated. We used a molecular approach involving polymerase chain reaction (PCR) and denaturing gradient gel electrophoresis (DGGE). Our results show the vulnerability of the ciliate community to soil solarization and its resilience after treatment.
MATERIALS AND METHODS
Study site
The impact of soil solarization on the ciliate community was studied in a greenhouse (720 m2) at Miyazaki Agricultural Research Institute (32.004°N, 131.463°E). The soil has been subjected to intervals of solarization for more than 10 years. Soil (haplic gray lowland soil, Gleyic Fluvisols) in the greenhouse was thoroughly, mechanically mixed using a farm tractor before the experiment. Some of the soil chemical properties were: pH, 5.96; electrical conductivity, 1.01 mS cm−1; total carbon (C), 3.10%; total nitrogen (N), 0.32%.
Soil solarization and tomato culture
The area in the closed greenhouse was divided into plots (5.67 m2 each). On July 22, 2013, commercial organic fertilizer (Del Plus 6-8-3, Kyushu–Showa Industry, Kagoshima, Japan) was added to the soil of four randomized plots, and liquid fertilizer prepared from distillery waste treatment of barley shochu (Japanese liquor; Unkai Shuzo Co. Ltd., Miyazaki, Japan) was added to the soil of another four plots. The amount of commercial organic fertilizer used was 133 g per plot, accounting for 460 kg C ha−1, 86 kg N ha−1, 97 kg P2O5 ha−1 and 60 kg K2O ha−1; the amount of liquid fertilizer from shochu waste used was 60 g per plot, accounting for 1,500 kg C ha−1, 220 kg N ha−1, 90 kg P2O5 ha−1 and 45 kg K2O ha−1. After ridging and saturating the soil in all plots with water, two plots with commercial organic fertilizer and two plots with shochu waste fertilizer were solarized by covering with a clear polyethylene sheet; as non-solarized controls, two plots with commercial organic fertilizer and two plots with shochu waste fertilizer were covered with a laminated aluminum sheet. Soils were covered with the sheets from July 24 to August 26, 2013. Soil temperature at a depth of 15 cm was monitored every hour with a temperature data logger (Thermo Recorder TR-52i, T&D Corporation, Nagano, Japan). The plastic sheets and aluminum sheets were removed at the end of the soil solarization period, and tomato seedlings (Solanum lycopersicum ‘Reiyou’ grafted onto ‘Boranchi’) were planted on September 30, 2013. The plants were grown and harvested until June 20, 2014.
Soil sampling
Soil samples (about 500 g) were collected from the top 10 cm of soil on solarized and non-solarized plots on July 24 (before solarization period), August 9 (mid-solarization period), August 26 (end of solarization period) and November 11 (tomato cultivation period). Soil samples were sieved (< 2 mm) and stored at −20°C until used.
DGGE analysis of ciliate community
DNA was extracted from 0.5 g of each of two soil samples from each plot using an ISOIL for Beads Beating (Nippon Gene, Toyama, Japan), according to the manufacturer’s instructions, and dissolved in 100 μL TE (pH 8.0).
We analyzed the ciliate community using DGGE modified after Jousset et al. (Citation2010). A fragment of the 18S rRNA gene was amplified from extracted DNA by semi-nested PCR. The reaction mixture (25 μL) of the first PCR contained 5 pmol each of CilF forward primer and three mixed CilR reverse primers (Lara et al. Citation2007), 250 μM of each deoxyribonucleoside triphosphate, 1.25 U Taq polymerase (TaKaRa, Tokyo, Japan), 2.5 μL of the 10× PCR buffer provided with the enzyme, and 0.5 μL of template DNA. The PCR program in a thermal cycler (TP-600, TaKaRa) included an initial denaturation step of 5 min at 94°C, followed by 30 cycles of denaturation (45 s, 94°C), primer annealing (1 min, 58°C) and primer extension (1 min, 72°C), and a final extension step of 10 min at 72°C.
The reaction mixture (50 μL) of the second PCR contained 10 pmol each of CilF-GC forward primer and CilDGGE-r reverse primer, 250 μM of each deoxyribonucleoside triphosphate, 2.5 U Taq polymerase (TaKaRa), 5 μL of the 10× PCR buffer provided with the enzyme and 1 μL of the amplicon of the first PCR. The PCR program included an initial denaturation step of 5 min at 94°C, followed by 30 cycles of denaturation (1 min, 94°C), primer annealing (1 min, 52°C) and primer extension (1 min, 72°C), and a final extension step of 10 min at 72°C.
The amplified 18S rRNA gene fragments (10 μL) were separated by DGGE with a DCode System (Bio-Rad, CA, USA) on 1-mm-thick 6% polyacrylamide gels with a gradient of 20–42% denaturant (100% denaturant contained 7 M urea and 40% vol/vol formamide). Electrophoresis was carried out in 1× TAE buffer at 100 V for 16 h at a constant temperature of 60°C. The gels were stained with 1:10,000 (vol/vol) SYBR Green I (BMA, Rockland, ME, USA) for 30 min and scanned with a Typhoon 9400 laser scanner (GE Healthcare, Little Chalfont, UK).
DGGE patterns were analyzed by non-metric multi-dimensional scaling (non-metric MDS) using the PRIMER-E software (Plymouth Marine Laboratory, Plymouth, UK). Binary data (presence or absence) of DGGE bands were used to calculate the Bray–Curtis similarity. ANOSIM (analysis of similarity) 2 analysis was used to test the null hypothesis that there was no effect of the treatments on the DGGE profiles. The average similarity among the duplicate plots was calculated and compared between the solarized and non-solarized plots to examine the impact of soil solarization on the homogeneity of ciliate community.
Sequencing amplicons in the DGGE bands
DGGE fragments were excised from the gels. After two rounds of re-amplification of excised fragments and confirming the mobility of the amplicons on a DGGE gel, the amplicons were sequenced as previously described (Murase et al. Citation2012).
Nucleotide sequence accession numbers
The sequences are available in the DDBJ/EMBL/GenBank nucleotide sequence databases under the accession numbers LC028289–LC028301.
RESULTS
Performance of soil solarization
The soil temperature was kept above 40°C for most of the solarization period, with the highest temperature at 71°C and an average temperature of 47–48°C, meeting the general requirement of soil solarization. The soil temperature in the non-solarized plots was much lower, with the highest temperature < 43°C (). The soil temperatures of the plots with the two different organic fertilizers did not markedly differ, although the plot with liquid fertilizer from shochu waste treatment had a slightly lower temperature at night.
Impact of solarization on DGGE banding patterns of the ciliate community
In the reaction mixture of the second PCR, we used the same high cycle number (30) and a high amount of template (1 μL of the amplicon of the first PCR) in order to achieve sufficient yields of PCR amplification to study the effect of soil solarization on the ciliate community structure. When the second PCR was conducted with a reduced cycle number (20) and less template (0.1 μL of amplicons of the first PCR), the PCR amplification yield from solarized soil was much lower than that from non-solarized soil during the solarization period (see Supporting Information, Fig. S1).
DGGE banding patterns before soil solarization (July 24) of replicates from the same plots were similar to each other and also to those of other treatment plots (). The DGGE banding patterns of samples from non-solarized soils remained stable throughout the experimental period. In contrast, the banding patterns of samples from solarized soils temporally changed; the number of bands decreased during solarization and the banding patterns between replicate plots and even between duplicate samples within the same plot differed at the end of the treatment (August 26). Solarization led to the disappearance of many prominent bands. In addition, one band was found at the end of the experiment only in solarized soil fertilized with commercial organic fertilizer (band 12, ). DGGE banding patterns of samples from solarized soil during the tomato cultivation period 76 days after solarization (November 11) were similar to those from non-solarized soils.
Figure 2 Impact of solarization on the denaturing gradient gel electrophoresis (DGGE) banding patterns of the ciliate community in soil fertilized with (A) commercial organic fertilizer and (B) liquid fertilizer from shochu waste. +, with solarization; –, without solarisation.
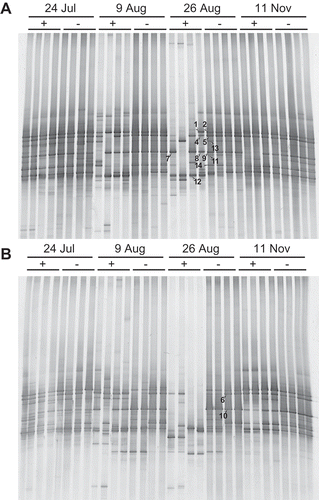
Non-metric MDS analysis represented the DGGE banding patterns well (, ). Soil solarization greatly altered the ciliate community, with a marked variation between replicates. An ANOSIM2 test indicated that the effect of soil solarization on DGGE profiles was significant for plots with either of the two different organic fertilizers (P < 0.001). The similarity of the banding patterns between replicate solarized plots was much lower than that between replicates of non-solarized plots (). The variation within the replicate solarized soils diminished, and the community structure became close to that of the non-solarized soils 76 days after the solarization. The results from the plots with the two different organic fertilizers were similar.
Figure 3 Non-metric multi-dimensional scaling using the Bray–Curtis similarity of the denaturing gradient gel electrophoresis (DGGE) banding patterns of polymerase chain reaction (PCR)-amplified ciliate 18S rRNA genes of soil fertilized with (A) commercial organic fertilizer and (B) liquid fertilizer from shochu waste. +, with solarization; –, without solarization.
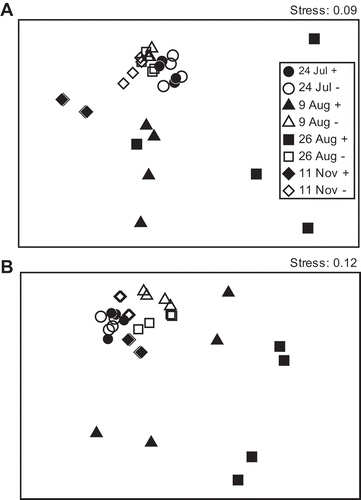
Figure 4 Impact of solarization on the Bray–Curtis similarity of the denaturing gradient gel electrophoresis (DGGE) patterns among duplicate plots. Soil fertilized with (A) commercial organic fertilizer and (B) liquid fertilizer from shochu waste. Bars represent the standard error of four combinations (duplicate plots × duplicate analyses).
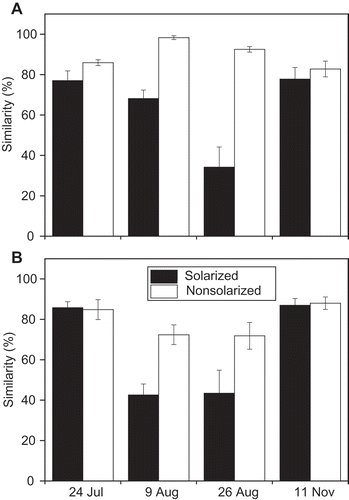
Phylogenetic affiliation of sequences of the amplicons in DGGE bands
Sequences of the major DGGE bands that were influenced by soil solarization showed close relationships to Sporadotrichida (Oxytricha sp.), Cyrtolophosida (Cyrtolophosis sp.) and Metopidae (Metopus sp.) (). The sequence of band 12, which appeared at the end of the experiment only in solarized soil fertilized with commercial organic fertilizer, was related to an environmental clone from soil but did not show a close relationship to any sequence of isolated ciliates in the database.
Table 1 Similarities of sequences from the excised bands to sequences in the NCBI database
DISCUSSION
In this study, we demonstrated that soil solarization greatly impacted the ciliate community structure of a greenhouse soil. Soil solarization also likely caused the number of ciliates to decrease, as demonstrated by the lower yield of PCR amplicons in the second PCR with a reduced cycle number and less template (Fig. S1). These results indicated the vulnerability of the soil ciliate community to solarization. The decrease in the number of ciliates in the soil may have caused the decreased spatial homogeneity of the ciliate community that was shown by the decreased similarity of DGGE banding patterns between replicate samples analyzed using 0.5 g of soil ().
Although non-solarized soil reached temperatures up to 43°C, the ciliate community did not markedly change, which suggested the resistance or adaptation of the ciliate community to this level of thermal stress. In keeping with the postulation of adaptation, a higher number of ciliates from non-solarized, burnt savannah soil able to be cultured at 40°C has been reported (Foissner Citation1987). The DGGE fragment that appeared at the end of the experiment only in solarized soil fertilized with commercial organic fertilizer (band 12 in ) might originate from thermophilic or thermotolerant ciliates.
DGGE patterns from samples 76 days after solarization (November 11) demonstrated that the original ciliate community reestablished in the soil following solarization. Many prominent bands did not completely disappear during solarization but remained at least in one of the four samples from the two replicate plots (two samples per plot). The recovery of the ciliate community suggests that the ciliates marginally survived in the solarized soil and proliferated after the treatment, which was supported by the increased amount of amplicons (Fig. S1). This is in contrast to steam sterilization of soil, which causes a large decrease in the number and species of culturable ciliates in soil even 100 days after treatment (Stout Citation1955). Chemical disinfection, including fumigation with methyl bromide (Yeates et al. Citation1991) and the use of pesticide and insecticide (Petz and Foissner Citation1989) also lead to a lag in the re-establishment of the soil ciliate community. Our results suggest that soil solarization may have a milder impact on ciliates than other methods of soil disinfection do. The greenhouse soil used in our study has been subjected to solarization for more than 10 years, and such a long-term treatment may shape the ciliate community to one that has resistance to moderately high temperature (up to 40°C), and resilience to the disturbance at higher temperatures. Further study is needed to understand the impact of different disinfection methods on soil ciliates.
The sequences of predominant DGGE fragments that disappeared by soil solarization were affiliated to Colpodea, Oxytrichidae and Armophorida (). Colpodea are regarded as r-selected species (Bamforth Citation1995) and are also known to have broad tolerance to harsh conditions (Lara and Acosta-Mercado Citation2012), while Oxytrichidae are regarded as K-selected species (Lüftenegger et al. Citation1985; Bamforth Citation1995). The results suggest that solarization has a great impact on both ecological types of ciliates but may not necessarily exterminate the K-selected species of ciliates in agricultural soils. The presence of Armophorida indicated the low-oxygen conditions of moistened soil as they are exclusively anaerobic (Lara and Acosta-Mercado Citation2012), and suggests that it is not anoxia but most likely high temperature that caused the marked change in the ciliate community in solarized soil.
DGGE analysis may underestimate the diversity of ciliates in soil as we observed 13–18 DGGE bands from samples from soil before solarization, and this number is lower than the number of ciliate species observed using the intensive non-flooded petri dish method (60–80 species; Foissner Citation1987). Nevertheless, our results demonstrate the utility of the ciliate community detected with molecular methods as a bioindicator of soil solarization to show the susceptibility and recovery of soil microbial community including the microbial food chain.
Following solarization, an increase in soluble minerals in soil is commonly observed (Stapleton Citation2000). Further research is needed to understand the ecological role of re-established ciliates as grazers in nutrient cycles and recolonization of pathogens in solarized soils.
SUPPLEMENTARY MATERIAL
The supplementary material for this article is available online from: http://dx.doi.org/10.1080/00380768.2015.1079799.
TSSP_A_1079799_supplementary_material.docx
Download MS Word (122 KB)ACKNOWLEDGMENTS
The authors are grateful to the staff of the Miyazaki Agricultural Research Institute for assistance. This study was financially supported by the Science and Technology Research Promotion Program for Agriculture, Forestry, Fisheries and Food Industry from the Ministry of Agriculture, Forestry and Fisheries of Japan.
REFERENCES
- Bamforth SS 1995: Interpreting soil ciliate biodiversity. Plant Soil, 170, 159–164. doi:10.1007/BF02183064
- Chen Y, Gamliel A, Stapleton JJ, Aviad T 1991: Chemical, physical, and microbial changes related to plant growth in disinfested soils. In Soil Solarization, Eds. Katan, J, DeVay, JE, pp. 103–129. CRC Press, Boca Raton.
- Culman SW, Duxbury JM, Lauren JG, Thies JE 2006: Microbial community response to soil solarization in Nepal’s rice-wheat cropping system. Soil Biol. Biochem., 38, 3359–3371. doi:10.1016/j.soilbio.2006.04.053
- Fenchel T 1987: Ecology of Protozoa: The Biology of Free-Living Phagotrophic Protists. Science Technology Publishers, Madison, Wisconsin.
- Foissner W 1987: Soil protozoa: fundamental problems, ecological significance, adaptations in ciliates and testaceans, bioindicators and guide to the literature. Prog. Protistol., 2, 69–212.
- Foissner W 1997: Protozoa as bioindicators in agroecosystems, with emphasis on farming practices, biocides, and biodiversity. Agric. Ecosyst. Environ., 62, 93–103. doi:10.1016/S0167-8809(96)01142-5
- Foissner W 1999: Soil protozoa as bioindicators: pros and cons, methods, diversity, representative examples. Agric. Ecosyst. Environ., 74, 95–112. doi:10.1016/S0167-8809(99)00032-8
- Foissner W, Berger H 1996: A user-friendly guide to the ciliates (Protozoa, Ciliophora) commonly used by hydrobiologists as bioindicators in rivers, lakes, and waste waters, with notes on their ecology. Freshw. Biol., 35, 375–482.
- Gelsomino A, Cacco G 2006: Compositional shifts of bacterial groups in a solarized and amended soil as determined by denaturing gradient gel electrophoresis. Soil Biol. Biochem., 38, 91–102. doi:10.1016/j.soilbio.2005.04.021
- Griffiths BS 1986: Mineralization of nitrogen and phosphorus by mixed cultures of the ciliate protozoan Colpoda steinii, the nematode Rhabditis sp. and the bacterium Pseudomonas fluorescens. Soil Biol. Biochem., 18, 637–641. doi:10.1016/0038-0717(86)90087-8
- Jousset A, Lara E, Nikolausz M, Harms H, Chatzinotas A 2010: Application of the denaturing gradient gel electrophoresis (DGGE) technique as an efficient diagnostic tool for ciliate communities in soil. Sci. Total Environ., 408, 1221–1225. doi:10.1016/j.scitotenv.2009.09.056
- Katan J, Greenberger A, Alon H, Grinstein A 1976: Solar heating by polyethylene mulching for control of diseases caused by soil-borne pathogens. Phytopathology, 66, 683–688. doi:10.1094/Phyto-66-683
- Lara E, Acosta-Mercado D 2012: A molecular perspective on ciliates as soil bioindicators. Eur. J. Soil Biol., 49, 107–111. doi:10.1016/j.ejsobi.2011.11.001
- Lara E, Berney C, Harms H, Chatzinotas A 2007: Cultivation-independent analysis reveals a shift in ciliate 18S rRNA gene diversity in a polycyclic aromatic hydrocarbon-polluted soil. FEMS Microbiol. Ecol., 62, 365–373.
- Lüftenegger G, Foissner W, Adam H 1985: r- and K-selection in soil ciliates - a field and experimental approach. Oecologia, 66, 574–579. doi:10.1007/BF00379352
- Murase J, Shibata M, Lee CG, Watanabe T, Asakawa S, Kimura M 2012: Incorporation of plant residue-derived carbon into the microeukaryotic community in a rice field soil revealed by DNA stable-isotope probing. FEMS Microbiol. Ecol., 79, 371–379. doi:10.1111/fem.2011.79.issue-2
- Murase J, Hida A, Ogawa K, Nonoyama T, Yoshikawa N, Imai K 2015: Impact of long-term fertilizer treatment on the microeukaryotic community structure of a rice field soil. Soil Biol. Biochem., 80, 237–243. doi:10.1016/j.soilbio.2014.10.015
- Petz W, Foissner W 1989: The effects of mancozeb and lindane on the soil microfauna of a spruce forest - a field-study using a completely randomized block design. Biol. Fertil. Soils, 7, 225–231. doi:10.1007/BF00709653
- Schönfeld J, Gelsomino A, van Overbeek LS, Gorissen A, Smalla K, van Elsas JD 2003: Effects of compost addition and simulated solarisation on the fate of Ralstonia solanacearum biovar 2 and indigenous bacteria in soil. FEMS Microbiol. Ecol., 43, 63–74. doi:10.1111/j.1574-6941.2003.tb01046.x
- Shimano S, Sambe M, Kasahara Y 2012: Application of nested PCR-DGGE (denaturing gradient gel electrophoresis) for the analysis of ciliate communities in soils. Microbes Environ., 27, 136–141. doi:10.1264/jsme2.ME11287
- Simmons CW, Claypool JT, Marshall MN et al. 2014: Characterization of bacterial communities in solarized soil amended with lignocellulosic organic matter. Appl. Soil Ecol., 73, 97–104. doi:10.1016/j.apsoil.2013.08.014
- Simmons CW, Guo HY, Claypool JT et al. 2013: Managing compost stability and amendment to soil to enhance soil heating during soil solarization. Waste Manage, 33, 1090–1096. doi:10.1016/j.wasman.2013.01.015
- Stapleton JJ 2000: Soil solarization in various agricultural production systems. Crop Protect., 19, 837–841. doi:10.1016/S0261-2194(00)00111-3
- Stout JD 1955: The effect of partial steam sterilization on the protozoan fauna of a greenhouse soil. J. Gen. Microbiol., 12, 237–240. doi:10.1099/00221287-12-2-237
- Vargas R, Hattori T 1986: Protozoan predation of bacterial-cells in soil aggregates. FEMS Microbiol. Ecol., 38, 233–242. doi:10.1111/j.1574-6968.1986.tb01733.x
- Yeates GW, Bamforth SS, Ross DJ, Tate KR, Sparling GP 1991: Recolonization of methyl-bromide sterilized soils under 4 different field conditions. Biol. Fertil. Soils, 11, 181–189. doi:10.1007/BF00335765
- Yokoe K, Maesaka M, Murase J, Asakawa S 2015: Solarization makes a great impact on the abundance and composition of microbial communities in soil. Soil Sci. Plant Nutr., 61, 641–652. doi:10.1080/00380768.2015.1048183