Abstract
Denitrification products nitrous oxide ((N2O) and nitrogen (N2)) were measured in three flooded soils (paddy soil from Vietnam, PV; mangrove soil from Vietnam, MV; paddy soil from Japan, PJ) with different nitrate (NO3–) concentrations. Closed incubation experiments were conducted in 100-mL bottles for 7 d at 25°C. Each bottle contained 2 g of air-dried soil and 25 mL solution with NO3– (concentration 0, 5 or 10 mg N L−1) with or without acetylene (C2H2). The N2O + N2 emissions were estimated by the C2H2 inhibition method. Results showed that N2O + N2 emissions for 7 d were positively correlated with those of NO3– removal from solution with C2H2 (R2 = 0.9872), indicating that most removed NO3– was transformed to N2O and N2 by denitrification. In PJ soil, N2O and N2 emissions were increased significantly (P < 0.05) by the addition of greater NO3– concentrations. However, N2O and N2 emissions from PV and MV soils were increased by the addition of 0 to 5 mg N L−1, but not by 5 to 10 mg N L−1. At 10 mg N L−1, N2 emissions for 7 d were greater in PJ soil (pH 7.0) than in PV (pH 5.8) or MV (pH 4.3) soils, while N2O emissions were higher in PV and MV soils than in PJ soil. In MV soil, N2O was the main product throughout the experiment. In conclusion, NO3– concentration and soil pH affected N2O and N2 emissions from three flooded soils.
INTRODUCTION
Denitrification is a microbial reaction in terrestrial and aquatic ecosystems in which nitrate (NO3–) is used as an alternative electron acceptor when oxygen is limited (Philippot et al. Citation2007). Denitrification is responsible for the emission of nitrous oxide (N2O) as an intermediate product. Nitrous oxide is a greenhouse gas and contributes to depletion of the stratospheric ozone layer (Conrad Citation1996). The Intergovernmental Panel on Climate Change (IPCC) reported that direct emission of N2O from agricultural soils has increased over the last few decades, in parallel with increasing use of fertilizers. In 2005, N2O emission from agriculture soils accounted for 60% of global anthropogenic fluxes (Smith et al. Citation2007).
Denitrification requires a substrate of NO3– as an electron acceptor (Buresh and De Datta Citation1990; Weier et al. Citation1993; Cheng et al. Citation2004); available organic carbon (C) as an electron donor (Weier et al. Citation1993; Chiu et al. Citation2004); the absence of oxygen, which is related to soil moisture contents at > 60% water-filled pore space (WFPS; Weier et al. Citation1993); suitable soil temperature, which is optimal at 25°C (Canion et al. Citation2014); and suitable soil pH, which ranges from 3.8 to 8.2 (optimum around pH 7; Šimek and Cooper Citation2002).
Denitrification is expected to be the main process of nitrogen (N) transformation in areas where soil and sediments contain sufficient organic C under anaerobic conditions and receive water with a high NO3– concentration. These areas include flooded rice fields (Reddy and Patrick Citation1986; Buresh and De Datta Citation1990), riparian wetlands (Hefting et al. Citation2004; Van den Heuvel Citation2010) and mangrove forests (Chiu et al. Citation2004; Fernandes and Loka Citation2011). In such flooded soils, the main products of denitrification (N2O or N2) were dependent on environmental conditions. In flooded rice soils, N2 was mainly produced by denitrification, because N2O was further reduced to N2 under anaerobic conditions (Huang et al. Citation2007; Iida et al. Citation2007). In contrast, in Tuvem mangrove sediments (Goa, India), incomplete denitrification was responsible for up to 43–93% of N2O production (Fernandes et al. Citation2010).
To our knowledge, the factors affecting denitrification end products (N2O and N2) in flooded soils remain uncertain. We supposed that different physical and chemical properties of soil under the same flooded condition affect denitrification products. Our study was conducted in laboratory microcosms to investigate the emissions of N2O and N2 from three soils (two paddy soils and a mangrove soil) under flooded conditions with different NO3– concentrations (0, 5 or 10 mg N L−1) in standing water. The objective of the study was to identify the controlling factors of N2O and N2 emissions from soils of different types under flooded conditions.
MATERIALS AND METHODS
Soil sampling sites
Soil samples were collected in May 2012 from lowlands, where soils are usually flooded: a paddy field in the Thanh Trung hamlet, Vietnam (PV; 16°31ʹ02”N, 107°30ʹ10”E); a mangrove forest located in the Huong Phong commune, Vietnam (MV; 16°33ʹ28”N, 107°36ʹ35”E); and a paddy field in Ushimado, Japan (PJ; 34°37ʹ22”N, 134°07ʹ40”E). The sampling sites were surrounded by agricultural fields. The PV, MV and PJ soils were classified according to the Food and Agriculture Organization (FAO) as Dystric Fluvisols, Gleyi-Salic Fluvisols and Dystric Gleysols, respectively. These flooded soils are usually under anaerobic conditions and rich in organic matter, which are favorable for denitrification. These areas would be expected to reduce the NO3– concentration of water entering from the agriculture fields by denitrification.
Soil properties
Soil samples were collected from the 0–20 cm layer and air-dried at room temperature, then passed through a 2-mm mesh sieve. All soil samples were subjected to measurements of pH and electrical conductivity (EC) at a soil:water ratio of 1:5 after shaking for 1 h at 175 rpm, using a digital pH meter (F-23, Horiba, Japan) and EC meter (DS-14, Horiba, Japan), respectively. Total carbon (TC) and total nitrogen (TN) contents of soil were determined using a CN Corder (MT-700, Yanaco, Japan). Mineral N was extracted with 2 M potassium chloride (KCl) solution (soil:solution ratio of 1:10). The ammonium (NH4+-N) and NO3–-N contents in extracts were measured by spectrophotometry using a continuous flow analyzer (QuAAtro 2-HR, Bltec, Japan). Dissolved organic carbon (DOC) was measured with a total organic carbon (TOC) analyzer (TOC-5000A, Shimadzu, Japan) at a soil:water ratio of 1:10. Cation exchange capacity (CEC) was determined with neutral ammonium acetate (NH4OAc; Chapman Citation1965). Soil texture was determined by the pipette method (Sarkar and Haldar Citation2005). Denitrifying bacteria in soils were estimated by the most probable number (MPN) method with Giltay’s medium (Ishikuri Citation2011).
Soils properties were as follows: TN, TC, DOC and CEC in the two paddy soils (PV and PJ) were higher than in the MV soil (). PJ soil was neutral while PV soil was moderately acid and MV soil was extremely acid. The density of denitrifying bacteria was the highest in PJ soil (2.7 × 104 MPN g−1), approximately 18 and 23 times higher than in PV and MV soils, respectively. Nitrate was not detected in all soil samples. The NH4+-N content was highest in the PV soil at 30.1 mg N kg−1, followed by those in MV and PJ soils.
Table 1 Selected properties of soils used.
Incubation experiment
Denitrification is a series of processes that transforms NO3– and nitrite (NO2–) to gaseous forms of NO, N2O, or N2. The acetylene inhibition method was used for estimating denitrification (Zaman et al. Citation2012). Because C2H2 inhibits the reduction of N2O to N2, we determined the denitrification rates as N2O production.
To prepare the C2H2-saturated solutions, C2H2 gas was passed through three Pyrex gas-washing bottles which contained 0.5 M sulfuric acid (H2SO4), deionized water and a certain concentration of NO3– solution (0, 5 or 10 mg N L−1), with a flow rate of 100 mL min−1 for 30 min. For three soil samples, closed incubation experiments were conducted in a 100-mL bottle at 25°C for 7 d under flooded conditions. Each bottle contained 2 g of air-dried soil and 25 mL of NO3– solution (0, 5 and 10 mg N L−1 in a solution are equivalent to 0, 63.4 and 126.5 mg N kg−1 oven-dried soil, respectively) with or without C2H2. All treatments were conducted in triplicate. A 0.5-mL gas sample was collected after 3 h on day 0 and days 1, 3 and 7 and analyzed for N2O using a gas chromatograph (GC-8A, Shimadzu, Japan) equipped with a 63Ni electron capture detector. Differences in N2O emissions during 7 d incubation with and without C2H2 were considered to be the amount of N2 emission during denitrification. The N2O and N2 emissions were calculated by the equation below on the weight basis of air-dried soil (mg N kg−1; Sun et al. Citation2012):
where VN2O is the N2O emission (mg N kg−1), V1 and V2 are the N2O emissions with and without C2H2, respectively, ρ is the density of N2O (1.25 kg m−3), Cg is the concentration of N2O in the gas phase (ppmv), Vg is the volume of head space (m3), VL is the volume of the liquid phase (m3), α is the Bunsen absorption coefficient (0.544 at 25°C), W is the dry soil weight (kg) and T is the temperature at sampling (25°C).
On day 7 of incubation, the butyl septum on the bottle was opened. The solution was taken out to analyze the NO3– concentration. Nitrate removal was obtained from the difference between NO3– concentrations on days 0 and 7.
Statistical analysis
Data was expressed as a mean ± standard deviation (SD). An analysis of variance (ANOVA) was performed to examine the effects of NO3– concentrations and soil types on N2O and N2 emissions at a significance level of 0.05. Linear regressions were analyzed to identify the relationship between N2O + N2 emissions and NO3– removal.
RESULTS AND DISCUSSION
Effect of NO3– concentrations on N2O and N2 emissions
At 25°C, N2O and N2 emissions in 63.4 and 126.5 mg N kg−1 NO3– treatments were much greater than those in treatments without NO3– (). Addition of NO3– significantly enhanced denitrification and emissions of N2O and N2 (Weier et al. Citation1993; Cheng et al. Citation2004). The effect of NO3– concentrations (63.4 and 126.5 mg N kg−1) on N2O and N2 emissions were significantly observed only in PJ soil (pH 7), while this effect was not clear in PV and MV soils (P > 0.05, ). In PJ soil, N2O and N2 emissions increased with increasing concentration of NO3–. Denitrification rates depend more on the bacterial population (Philippot and Hallin Citation2005). The populations of denitrifying bacteria in PV and MV soils were smaller than in PJ soil (). Accordingly, PV and MV soils transformed less NO3– to N2O and N2 at higher NO3– concentrations than PJ soil (; ). Nitrate removal from solutions with C2H2 were positively correlated with those of denitrification in three flooded soils (R2 = 0.9872; ). Most removed NO3–-N was transformed to N2O and N2 by denitrification within 7 d because the slope of the regression line was nearly equal to 1 ().
Table 2 Change of NO3– (mg N kg−1)† after 7 d incubation with acetylene at 25°C.
Figure 1 Accumulated nitrous oxide (N2O) emissions (mg N kg–1) in (a) PV, (b) MV, and (c) PJ soils and nitrogen (N2) emissions (mg N kg–1) in (d) PV, (e) MV, and (f) PJ soils during 7 d incubation at 25°C. Bars indicate standard deviation, n = 3. Note: N2O emission (mg N kg–1) was measured in the incubation bottle without acetylene (C2H2). N2 emission (mg N kg–1) was calculated by the differences in N2O emissions during 7 d incubation with and without C2H2. PV, paddy soil from Vietnam; MV, mangrove soil from Vietnam; PJ, paddy soil from Japan.
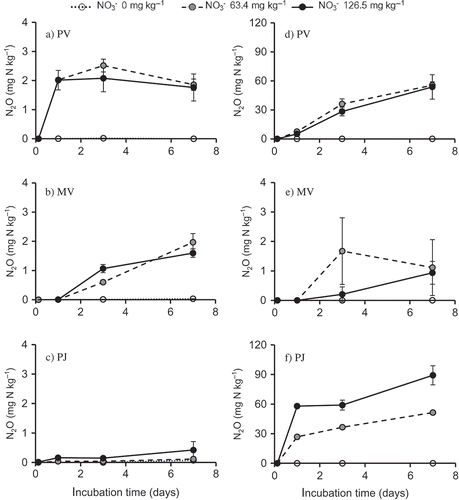
Effect of soil pH on N2O and N2 emissions
After 7 d incubation at 25°C with 126.5 mg N kg−1, N2O emissions in PV and MV soils (1.8 and 1.6 mg N kg−1, respectively) were significantly higher than in PJ soil (0.4 mg N kg−1; a–c). Nitrogen (N2) emission in the 126.5 mg N kg−1 treatment was highest in PJ soil (89.2 mg N kg−1), followed by PV soil (53.7 mg N kg−1). MV soil produced the lowest N2 emissions regardless of NO3– concentrations, with a range from 0 to 1.1 mg N kg−1 (d–f). Denitrification (N2 + N2O) was the highest in PJ soil (pH 7.0), followed by PV (pH 5.8) and MV (pH 4.3) soils.
In MV soil, N2O was the main product of denitrification. In contrast, N2 was the main product of denitrification in PJ and PV soils. The net N2O production will be strongly affected by the rate of further reduction to N2, and therefore the N2O:N2 ratio is an important parameter in determining N2O production originating from denitrification (Van den Heuvel Citation2010). Results on day 7 showed that the N2O:N2 molar ratio was the highest in acidic MV soil (1.7), followed by PV soil (0.03) and PJ soil (0.005). The N2O:N2 ratio increased with the decrease in soil pH.
Previous studies have highlighted the effects of pH on denitrification, and on N2O and N2 emissions (Koskinen and Keeney Citation1982; Šimek and Cooper Citation2002; Čuhel and Šimek Citation2011; Van den Heuvel et al. Citation2011). Denitrification was low at pH 5 or below, and the optimum pH seemed to lie between 7 and 8 (reviewed in Šimek and Cooper Citation2002). In this study, soil pH was extremely acid, moderately acid and neutral for MV, PV and PJ soils, respectively. Probably due to the neutral pH, denitrification (N2O + N2) in PJ soil was higher than in PV and MV soils. Our study is consistent with previous studies in terms of the effect of pH on denitrification. Several laboratory experiments using pH-modified treatments and field experiments have confirmed that soil pH affects denitrification end products N2O and N2. Sun et al. (Citation2012) showed that N2O was the major component of gas products of denitrification in forest soils (acid soils), while N2 was the dominant product in grassland soil (close to neutral soil). Koskinen and Keeney (Citation1982) conducted a field experiment in which silt loam soil was maintained at different pH. They found that at the near-neutral pH of 6.9, N2 was the main product, and a more acidic condition (pH 4.6 and 5.4) favored the emission of N2O. In our study, higher N2O emission by denitrification occurred in MV soil, because a small amount of N2O was transformed to N2. The mechanism of pH controlling denitrification products is explained by the sensitivity of N2O reductase activity to pH. Under acidic pH, the activity and synthesis of N2O reductase is inhibited, resulting in increased N2O accumulation (Šimek and Cooper Citation2002). Soil pH can be used as a predictive parameter for denitrification and N2O emission (Čuhel and Šimek Citation2011).
CONCLUSIONS
We investigated controlling factors of N2O and N2 emissions from three flooded soils of different types and NO3– concentrations. Results showed that denitrification (N2O + N2) in PJ soil was NO3– limited. In PV and MV soils, denitrification was increased by NO3– addition from 0 to 63.4 mg N kg−1, but was not affected by that from 63.4 to 126.5 mg N kg−1. Denitrification products (N2O and N2) varied among the studied soils. Denitrification (N2O + N2) was higher in the neutral soil (PJ) than that in the moderately acidic soil (PV) and the extremely acidic soil (MV). Nitrous oxide was the main product in MV (acidic soil) while N2 was the dominant product in PJ and PV soils. We suggest that NO3– concentration and soil pH affected N2O and N2 emissions in three flooded soils.
ACKNOWLEDGMENTS
The authors wish to thank Mr. Tetsuo Idei in Ushimado, Japan, for permission to collect soil samples. This work was supported by the Japan Science and Technology Agency, Core Research of Evolutional Science and Technology (JST CREST). The first author gives thanks for the scholarships provided by the Okayama Gas Company and the Ministry of Education, Culture, Sports, Scientific and Technology, Japan (MEXT).
REFERENCES
- Buresh RJ, De Datta SK 1990: Denitrification losses from puddled rice soils in the tropics. Biol. Fertil. Soils, 9, 1–13.
- Canion A, Overholt WA, Kostka JE, Huettel M, Lavik G, Kuypers MMM 2014: Temperature response of denitrification and anaerobic ammonium oxidation rates and microbial community structure in Arctic fjord sediments. Environ. Microbiol., 16, 3331–3344.
- Chapman HD 1965: Cation exchange capacity. In Method of Soil Analysis- Chemical and Microbiological Properties, Eds. Black, CA, pp. 891–901. American Society of Agronomy, Madison.
- Cheng W, Tsuruta H, Chen G, Akiyama H, Yagi K 2004: N2O and N2 production potential in various Chinese agricultural soils by denitrification. Soil Sci. Plant Nutr., 50, 909–915. doi:10.1080/00380768.2004.10408553
- Chiu CY, Lee SC, Chen TH, Tian G 2004: Denitrification associated N loss in mangrove soil. Nutri. Cycl. Agroecosyst. 69, 185–189.
- Conrad R 1996: Soil microorganisms as controllers of atmospheric trace gases (H2, CO, CH4, OCS, N2O, and NO). Microbiol. Rev., 60, 609–640.
- Čuhel J, Šimek M 2011: Effect of pH on the denitrifying enzyme activity in pasture soils in relation to the intrinsic differences in denitrifier communities. Folia Microbiol., 56, 230–235. doi:10.1007/s12223-011-0045-x
- Fernandes SO, Bharathi PAL, Bonin PC, Michotey VD 2010: Denitrification: an important pathway for nitrous oxide production in tropical mangrove sediments (Goa, India). J. Environ. Qual., 39, 1507–1516. doi:10.2134/jeq2009.0477
- Fernandes SO, Loka BPA 2011: Nitrate levels modulate denitrification activity in tropical mangrove sediments (Goa, India). Environ. Monit. Assess., 173, 117–125. doi:10.1007/s10661-010-1375-x
- Hefting M, Clément JC, Dowrick D, Cosandey AC, Bernal S, Cimpian C, Tatur A, Burt TP, Pinay G 2004: Water table elevation controls on soil nitrogen cycling in riparian wetlands along a European climatic gradient. Biogeochemistry. 67, 113–134. doi:10.1023/B:BIOG.0000015320.69868.33
- Huang S, Pant HK, Lu J 2007: Effects of water regimes on nitrous oxide emission from soils. Ecol. Eng., 31, 9–15.
- Iida T, Deb SK, Kharbuja RG 2007: Nitrous oxide emission measurement with acetylene inhibition method in paddy fields under flood conditions. Paddy Water Environ., 5, 83–91.
- Ishikuri S 2011: The MPN method. In Analytical Methods for Soil Microorganism, Eds. Japanese Scociety of Soil Microbiology, pp. 45–54. Yokendo, Tokyo, Japan. (in Japanese).
- Koskinen WC, Keeney DR 1982: Effect of pH on the rate of gaseous products of denitrification in a silt loam soil. Soil Sci. Soc. Am. J., 46, 1165–1167.
- Philippot L, Hallin S 2005: Finding the missing link between diversity and activity using denitrifying bacteria as a model functional community. Curr. Opin. Microbiol., 8, 234–239. doi:10.1016/j.mib.2005.04.003
- Philippot L, Hallin S, Schloter M 2007: Ecology of denitrifying prokaryotes in agricultural soil. Adv. Agron., 96, 249–305.
- Reddy KR, Patrick WH, Jr. 1986: Denitrification losses in flooded rice fields. Fertil. Res., 9, 99–116. doi:10.1007/BF01048697
- Sarkar D, Haldar A 2005: Soil physics. In Physical and Chemical Methods in Soil Analysis, Eds. Sarkar, D, Haldar, A, pp. 34–41. New Age International Publishers, India.
- Šimek M, Cooper JE 2002: The influence of soil pH on denitrification: progress towards the understanding of this interaction over the last 50 years. Eur. J. Soil Sci., 53, 345–354. doi:10.1046/j.1365-2389.2002.00461.x
- Smith P, Martino D, Cai Z et al. 2007: Agriculture. In Climate Change 2007: Mitigation, Eds. Metz, B, Davidson, OR, Bosch, PR, Dave, R, Meyer, LA, pp. 449–532. Cambridge University Press, Cambridge, United Kingdom and New York, USA.
- Sun P, Zhuge Y, Zhang J, Cai Z 2012: Soil pH was the main controlling factor of the denitrification rates and N2/N2O emission ratios in forest and grassland soils along the Northeast China Transect (NECT). Soil Sci. Plant Nutr., 58, 517–525. doi:10.1080/00380768.2012.703609
- Van den Heuvel RN 2010: Nitrous oxide emission hotspots and acidic soil denitrification in a riparian buffer zone. Faculty of Science, Ph.D. Thesis, pp. 128. Utrecht University, Netherlands.
- Van den Heuvel RN, Bakker SE, Jetten MSM, Hefting MM 2011: Decreased N2O reduction by low soil pH causes high N2O emissions in a riparian ecosystem. Geobiology. 9, 294–300. doi:10.1111/gbi.2011.9.issue-3
- Weier KL, Doran JW, Power JF, Walters DT 1993: Denitrification and the dinitrogen/nitrous oxide ratio as affected by soil water, available carbon, and nitrate. Soil Sci. Soc. Am. J., 57, 66–72. doi:10.2136/sssaj1993.03615995005700010013x
- Zaman M, Nguyen ML, Šimek M, Nawaz S, Khan MJ, Babar MN, Zaman S 2012: Emissions of nitrous oxide (N2O) and di-nitrogen (N2) from the agricultural landscapes, sources, sinks, and factors affecting N2O and N2 ratios. In Greenhouse Gases - Emission, Measurement and Management, Eds. Liu, G, pp. 1–31. Shanghai: InTech. http://cdn.intechopen.com.pdfs/32340.pdf