ABSTRACT
Previously we have shown that site-specific application of glutathione (GSH) to roots inhibits cadmium (Cd) translocation from roots to shoots. In this study, we investigated the effects of chemical compounds containing sulfur, such as dithiothreitol (DTT), potassium sulfate (K2SO4) and a GSH synthesis inhibitor, buthionine sulfoximine (BSO), on the behavior of Cd in oilseed rape plants (Brassica napus L.). We found that DTT, just like GSH, also inhibited Cd translocation from roots to shoots significantly. However, the inhibitory effects of Cd behaviors differed between GSH and DTT. Cd efflux from root cells was activated by GSH and inactivated by DTT. Positron imaging experiments revealed that there are minor differences in the effects of GSH and DTT on Cd adsorption to the surface of roots. These results provide insights into the function of GSH involved in the inhibitory effects of Cd translocation from roots to shoots.
1. Introduction
Cadmium (Cd) is a toxic heavy metal that leads to serious health problems when it enters the food chain (Obata and Umebayashi Citation1999). Increasing amounts of Cd are released into the environment, making agricultural products less safe for consumption. To establish novel cultivation methods for producing safe agricultural products, it is necessary to elucidate and control the molecular mechanisms of the behavior of Cd in plants.
Sasaki et al. (Citation2012) revealed that OsNramp5, which encodes a natural resistance-associated macrophage protein, is the major transporter responsible for Cd uptake by roots in rice plants (Oryza sativa L.). Mutations of this gene decrease the Cd contents in the shoots of rice plants significantly (Ishikawa et al. Citation2012). The molecular mechanisms of Cd transport and tolerance in rice plants have been reviewed by Uraguchi and Fujiwara (Citation2012). The behavior of Cd in other crop species such as leafy vegetables is not fully understood. The Codex Alimentarius Commission, which established the maximum acceptable Cd accumulation level in leafy vegetables (Codex Alimentarius Citation2015), determined the maximum level of Cd content in cruciferous vegetables as 0.05 mg kg−1 (Codex Alimentarius Citation2015). Given the Cd pollution worldwide, it is necessary to establish a novel cultivation method to reduce Cd accumulation in these vegetables.
Previously, Nakamura et al. (Citation2013) found that application of glutathione (GSH) to roots inhibits Cd transport from roots to shoots in oilseed rape (Brassica napus L.). This study demonstrated that one of the causes of this phenomenon is the activation of Cd efflux from root cells by GSH. In order to trigger the inhibitory effects of Cd translocation, the GSH concentration should exceed a certain threshold amount in the roots (Nakamura et al. Citation2015). GSH is involved in various physiological activities, such as removal of oxygen species (Noctor and Foyer Citation1998), heavy metal detoxification (Mendoza-Cózatl et al. Citation2005), pathogen resistance (Mou et al. Citation2003) and sugar metabolism (Ito et al. Citation2003). Elucidation of the molecular mechanisms of GSH involved in Cd behaviors in plants would enable the establishment of novel cultivation methods for producing leafy vegetables with low Cd. If we are able to obtain inhibitory effects of Cd from some cheap compounds with a simpler molecular structure than GSH, production cost could be reduced.
This study investigated the effects of a commonly available reducing agent, dithiothreitol (DTT), along with other compounds with an SH group, such as GSH, and buthionine sulfoximine (BSO), a GSH synthesis inhibitor, on Cd behaviors in oilseed rape plants. Our initial results showed that both GSH and DTT had significant inhibitory effects on Cd translocation from roots to shoots. We then examined the effects of GSH and DTT on Cd efflux from roots and Cd distribution in roots of oilseed rape plants. Additionally, we attempted to visualize the behavior of Cd in GSH- and DTT-treated plants using a positron-emitting tracer imaging system (PETIS). Simultaneously, we also examined the effects of other sulfur-containing substances with a simpler structure than GSH, such as potassium sulfate (K2SO4), and cysteine (Cys), a precursor of glutathione, on Cd behavior in oilseed rape plants.
2. Materials and methods
2.1. Plant materials
Oilseed rape plants (Brassica napus L. cv. Nourin No. 16, Kaneko Seed, Gumma, Japan) were grown hydroponically in a growth chamber under controlled conditions as described in (Nakamura et al. Citation2013). Modified Hoagland solution (Nakamura et al. Citation2008) was used as nutrient solution.
2.2. Plant treatment
Plants were exposed to 10 µM cadmium chloride (CdCl2) for 2 d or 2 weeks depending on the experiment. Sulfur containing reagents and glutathione synthesis inhibitor were applied to roots at the same time with these Cd treatments. GSH, DTT, Cys, K2SO4 and BSO (final concentration; 1 mM) were added to the nutrient solution including Cd. Each plant was grown for 4 weeks before harvest.
2.3. Measurement of Cd, Fe, Mn and Zn accumulation in plants
Harvested plants were treated according to the methods of Nakamura et al. (Citation2013). Cd, iron (Fe), manganese (Mn) and zinc (Zn) concentrations were measured simultaneously in the prepared samples with an inductively coupled plasma optical emission spectrometer (ICP-OES, iCAP 6000 SERIES, Thermo Fisher Scientific Inc., USA). Heavy metal contents in shoots and roots were calculated from these experimental results. Translocation ratios of these heavy metals indicate ratios of heavy metals translocated to shoots compared to those absorbed by the roots.
2.4. Collection of symplast sap and apoplast sap from oilseed rape plants and measurements of Cd concentration in these saps
After Cd treatments, root cell saps (both symplast and apoplast saps) were collected by centrifugation following the methods of Yu et al. (Citation1999). After sap collection, remnants were dried at 105°C overnight, weighed and then digested in a microwave oven (ETHOS 1600, Milestone, Italy). Cd concentration in collected saps and digested solution were measured with an atomic absorption photometer (AA280Z, Varian, USA). From these experimental results, Cd distribution ratio in roots was calculated.
2.5. Effects of GSH and DTT on Cd efflux from root cells
Cd efflux from root cells of oilseed rape plants was investigated according to the methods of Nakamura et al. (Citation2013). Three-week-old plants were used in these experiments. Prior to monitoring Cd efflux from roots, plants were pre-treated with Cd alone for 24 h. In Cd pre-treatment, every plant used in the Cd efflux assay was treated with 10 µM Cd alone under the same conditions. Therefore, Cd contents in tested plants were almost the same before Cd efflux assay. After washing the roots with a washing solution, each plant was transferred to a light-shielded bottle with 0.6 L of each nutrient solution for 24 h. Cd efflux from roots were calculated from Cd concentration in solution and root fresh weight (FW).
2.6. Positron imaging of Cd behavior in plants by PETIS
107Cd (half-life of decay: 6.5 h), produced and purified following the methods of Fujimaki et al. (Citation2010), was used as a positron-emitting radiotracer. Approximately 16 MBq of 107Cd dissolved in an appropriate volume of 0.5 mM calcium chloride (CaCl2) and 10 μM non-radioactive CdCl2 with 1 mM GSH or 1 mM DTT was prepared to each test plant. Two-week-old plants were set in a syringe and PETIS experiments were started by adding this solution. Images of 107Cd distribution in the field of view were obtained every 4 min for 36 h.
2.7. Statistical analysis
Obtained data were analyzed by Student’s t-test at a significance level of 0.01 or 0.05.
3. Results
3.1. Effects of reagents applied to roots on Cd accumulation in oilseed rape plants
To examine the effects of DTT, BSO, K2SO4 and Cys on Cd accumulation, Cd contents in shoots and roots of oilseed rape plants were measured after 2 d and 2 weeks of Cd exposure. At the time of harvest after 2 d of treatment, total dry weight (DW; shoot dry weight plus root dry weight) in control plants was about 1.5 g plant−1 (). The treated plants did not show any significant changes in total dry weight compared to controls after 2 d of treatment (). After 2 d of treatment, Cd content in shoots was about 0.4 µmol (g DW−1) in control plants. Shoot Cd content decreased significantly in plants exposed to GSH, DTT, BSO and Cys (). However, K2SO4, applied to roots, had no effect on shoot Cd content (). DTT applied to roots decreased the Cd content in roots (). However, unlike the Cd content in shoots, the root Cd content was not affected by GSH, BSO and Cys (). Cd translocation ratios were calculated from these experimental results. Cd translocation ratio was about half of control values and was significantly different when GSH was applied to roots (). As opposed to the plants treated for 2 d, plants treated with Cd and DTT for 2 weeks had a significant decrease in total dry weight (). On the other hand, shoot Cd content, root Cd content and Cd translocation ratio in control plants and plants exposed to GSH, DTT, K2SO4 and Cys for 2 weeks showed similar trends to those seen in plants treated with reagents for 2 d ( and ). BSO-treated plants, however, exhibited differences in Cd translocation ratio depending on the length of treatment ( and ) in that the Cd translocation ratio increased significantly in plants treated with Cd and BSO for 2 weeks () compared to those treated for 2 d (). In these experiments, we also measured Fe, Mn and Zn contents in harvested plants and found that the absorption of these elements by roots was not inhibited by GSH and DTT treatment for 2 d (Supplementary Fig. S1).
Figure 1. (A) Total dry weight of oilseed rape plants (Brassica napus L.) harvested after 2 d of treatment. (B, C) Cadmium (Cd) content in the shoots (B) and roots (C) of oilseed rape plants harvested after each treatment. (D) Cd translocation ratio of oilseed rape plants. Ratios were calculated from experimental results of dry weights and Cd content in shoots and roots. Data are means ± standard error (SE; n > 4). Values labeled with * and ** are significantly different from those of control plants according to Student’s t-test (*: P < 0.05, **: P < 0.01).
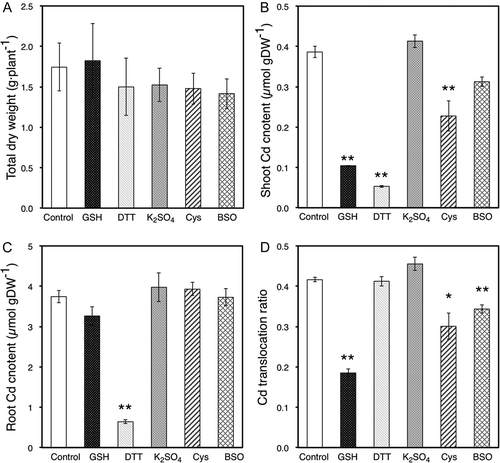
Figure 2. (A) Total dry weight of oilseed rape plants (Brassica napus L.) harvested after 2 weeks of treatment. (B, C): Cadmium (Cd) contents in the shoots (B) and roots (C) of oilseed rape plants harvested after each treatment. (D) Cd translocation ratio of oilseed rape plants, calculated from experimental results of dry weights and Cd content in shoots and roots. Data are means ± standard error (SE; n > 4). Values labeled with * and ** are significantly different from those of control plants according to Student’s t-test (*; P < 0.05, **; P < 0.01).
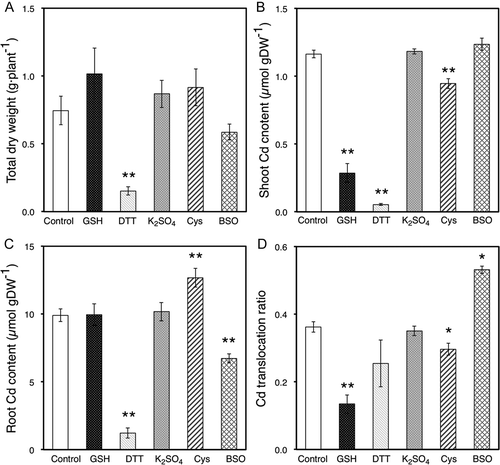
3.2. Effects of GSH and DTT in the root zone on Cd distribution in roots of oilseed rape plants
Application of DTT to roots inhibited Cd translocation from roots to shoots significantly, as well as with the application of GSH. To understand the effects of GSH and DTT in the root zone in detail, Cd distribution in roots was investigated after treatment with DTT or GSH. With the application of GSH or DTT to roots for 2 d, the Cd distributions in root symplast and apoplast decreased considerably compared to control plants (). An even higher reduction was observed when the treatment was extended to 2 weeks ().
Figure 3. Cadmium (Cd) distribution ratio in the symplast sap, apoplast sap and other parts of roots from oilseed rape plants (Brassica napus L.). These ratios were calculated from experimental results of atomic absorption spectrometry analysis and the amount of symplast sap, apoplast sap and the residuals.
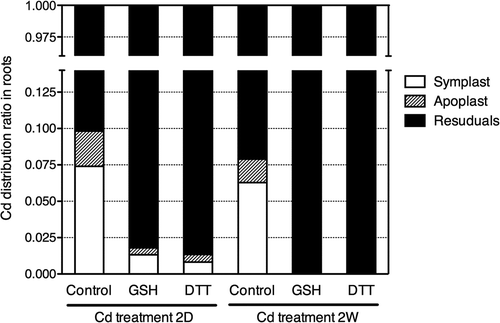
3.3. Effects of GSH and DTT on Cd efflux from root cells
In our previous study, we found that application of GSH to roots activated Cd efflux from root cells (Nakamura et al. Citation2013). In this study, we tested the effects of GSH and DTT applied to the nutrient solution on Cd efflux from root cells (). After Cd pre-treatment, tested plants had almost the same Cd level by Cd treatment under the same condition. The Cd amount excreted into nutrient solution was about 147 nmol FW−1 (). Cd amounts excreted into nutrient solution with GSH or DTT were about 172 nmol FW−1 and 69 nmol FW−1, respectively (). Cd efflux from root cells was activated in GSH-treated plants compared to control plants (). On the contrary, it was significantly inhibited by DTT in the root zone ().
3.4. Analysis of cd behaviors in oilseed rape plants by PETIS
Next we monitored the behavior of Cd in oilseed rape plants by PETIS. shows plants tested in PETIS experiments. A representative time series of PETIS images is presented in . It should be noted that all of the 107Cd intensities and values in the images and graphs from PETIS experiments have already been decay-corrected, which produces direct amounts of total Cd (non-radioactive Cd plus 107Cd). In GSH- and DTT-treated plants, 107Cd signals in the shoot remained weaker compared to those of control plants (). In control plants, strong 107Cd signals appeared at the shoot base and node where the petiole appeared (). shows the regions of interest (ROI) that were analyzed. Time courses of 107Cd intensities per unit area (time activity curve; TAC) were generated from the shoot base, node, upper root and lower root (). The upper root represents roots that existed outside of the hydroponic solution. The lower root represents roots immersed in the hydroponic solution. In the TAC from shoot base, 107Cd signals increased gradually to about 10 h in all plants. After that, 107Cd signals in the shoot base of control plants increased more sharply than those in GSH- and DTT-treated plants (). In the TAC from node, 107Cd signals in control plants also increased sharply after about 16 h from 107Cd feeding (). In the TAC from the upper root, there were major differences in accumulation of 107Cd signals between control plants and GSH- and DTT-treated plants with Cd accumulation reduced to almost one third of the control with both the treatments (). On the other hand, in the TAC from the lower root, there was little difference in the accumulation of 107Cd signals between control plants and GSH- and DTT-treated plants ().
Figure 4. Cadmium (Cd) efflux from root cells of oilseed rape plants (Brassica napus L.). The amount of Cd released from root cells to nutrient solution with GSH or DTT applied for 24 h was investigated. Experimental results are indicated as the amount of Cd in the nutrient solution normalized by root fresh weight. Data are means ± standard error (SE; n > 4). Value labeled with ** is significantly different from that of control plants according to Student’s t-test (P < 0.01).
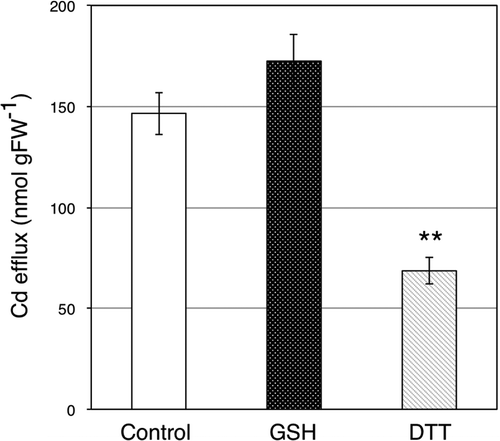
Figure 5. PETIS imaging of cadmium (Cd) behavior in oilseed rape plants (Brassica napus L.). (A) Field of view of a representative PETIS experiment. (B) Time series of PETIS images showing the 107Cd signal (0–36 h) after decay correction. Each image shown is a composite of 45 original images collected every 4 min. Each plant treatment is shown in the lower part of .
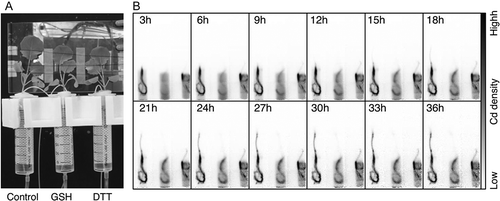
Figure 6. Time-course analyses of cadmium (Cd) behavior in the node and shoot base. (A) regions of interest (ROIs) for time-course analyses. Circles in panel A indicate ROI from shoot base and node (B, C); polygons in panel A also indicate ROI from upper root and lower root (D, E): time activity curves of 107Cd signal in ROI from shoot base (B) node (C), upper root (D) and lower root (E) are plotted. Each graph indicates the intensity of 107Cd signal after decay correction.
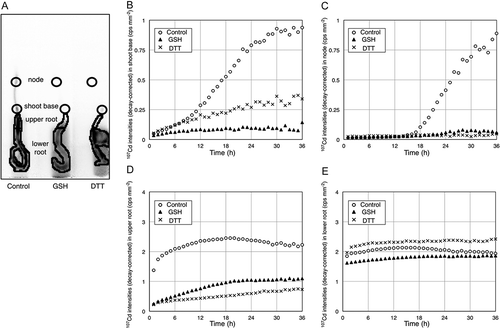
4. Discussion
4.1. Effects of reagents applied to roots on Cd accumulation in oilseed rape plants
Our results showed that short-term application of DTT did not have significant effects on the total DW of plants (). For any significant effect to show, at least 2 weeks of exposure to DTT was needed. DTT applied to roots for 2 weeks inhibited plant growth significantly (). Several cellular mechanisms that operate to control the redox state of elements in the cell have been attributed to GSH (Meyer and Hell Citation2005). DTT is a substance that is not naturally present in plants. Therefore, long-term DTT treatment might break the redox balance in plant bodies and, consequently, exhibit some effects on plant growth.
Cd accumulation in shoots decreased to less than 50% of the control by GSH and DTT in the root zone ( and ). Our results also indicated that shoot contents and root contents of other elements, such as Fe, Mn and Zn, did not decrease significantly with GSH and DTT treatment for 2 d. ZIP family proteins, which are involved in Fe, Mn and Zn absorption by roots, have also been shown to be related to Cd absorption in roots (Guerinot Citation2000). In these experimental results, the inhibitory effect of translocation from roots to shoots was specific to Cd. GSH (Rouhier et al. Citation2008) and DTT (Kim et al. Citation1985) are known to act by cleaving the disulfide bonds in proteins. The GSH synthesis inhibitor BSO increased the Cd translocation ratio when applied for 2 weeks, indicating that endogenous GSH also contributes to the inhibition of Cd translocation (). K2SO4 and Cys applied to roots had zero or minimal inhibitory effects on the behavior of Cd in plants compared to the effects of GSH (), indicating that it is difficult to inhibit Cd translocation from roots to shoots by using GSH precursors like K2SO4 and Cys.
4.2. Effects of GSH and DTT on Cd distribution in roots and Cd efflux from roots
Application of DTT to roots inhibited Cd translocation from roots to shoots significantly, as did the application of GSH (). However, there was a significant difference in Cd translocation ratio between GSH and DTT. To understand the effects of GSH and DTT in the root zone in more detail, we investigated the Cd distribution in roots and Cd efflux from roots. The Cd distribution ratio in the symplast and apoplast saps in roots of oilseed rape plants decreased when GSH and DTT were present in the root zone (). A similar trend was observed in the 2-d and 2-week treatment experiments (). GSH is known to be a precursor of phytochelatins (PCs; Rauser Citation1995). PCs play an important role in sequestering Cd into vacuoles. BSO inhibits GSH synthesis in roots. This leads to a decrease in sequestration of Cd into vacuoles and an increase in Cd translocation from roots to shoots. Our experimental results support that GSH and PCs are playing a role in the radial transport of Cd in roots. It has been reported that the cytosolic Cd concentration in root cells had large effects on Cd xylem loading (Uraguchi et al. Citation2009). A decrease in Cd concentration in root cells leads to a decrease in Cd accumulation in shoots. There were large differences in Cd content in roots of GSH- and DTT-treated plants ( and ). GSH and DTT had different effects on Cd efflux from root cells (). There are also reports suggesting that peptides with SH groups limited Cd accumulation inside the cells (Kuramata et al. Citation2009). In future studies, it is necessary to investigate substances induced by GSH that exist outside of the cells.
4.3. Analysis of the behavior of Cd in GSH- and DTT-treated plants by PETIS
PETIS enabled us to visualize the behavior of Cd in GSH- and DTT-treated plants (). We could observe the inhibitory effects of GSH and DTT on Cd translocation from roots to shoots ( and , ). GSH and DTT in the root zone decreased Cd accumulation in the shoot base, suggesting that xylem loading of Cd was restricted. In control plants, strong 107Cd signals appeared in the nodes of oilseed rape plants ( and ). Nodes have been reported to be involved in the Cd transport into phloem (Uraguchi et al. Citation2011). The involvement and importance of nodes in plants for distribution of mineral elements were reviewed by Yamaji and Ma (Citation2014). We observed minor differences in 107Cd signals in the lower root from control plants and GSH- and DTT-treated plants (). Yoshihara et al. (Citation2013) reported that Cd accumulating capacity in plant roots involves both a physical process and a physiological process. Our experimental results indicated that the application of GSH and DTT to roots had no effects on the physical process of Cd accumulation in root of oilseed rape plants. On the other hand, there was a major difference in 107Cd signals in the upper root from control plants and GSH- and DTT-treated plants (). 107Cd signals in the upper root were decreased by GSH and DTT treatment (). These results demonstrated that GSH and DTT inhibited Cd that entered the root cells. In this study, two ROIs (upper root and lower root) were set in the roots of tested plants (). There was a major difference in accumulation of 107Cd signals in ROIs from upper roots and those from lower roots when control plants and GSH- treated plants were compared (, ). In our previous study, there was no difference in accumulation of 107Cd signals in roots from control plants and GSH- treated plants (Nakamura et al. Citation2013). In our previous study, ROIs were set to the entire root of tested plants (Nakamura et al. Citation2013). The area of ROI from the lower root is much larger than that from the upper root (). There is no discrepancy between this study and our previous study when ROIs are set under the same condition. The difference in accumulation of 107Cd signals in upper root and lower root gave us insights into functions of GSH in roots.
There was some discrepancy in cultivation experiments and positron imaging experiments. The Cd content in the roots of DTT-treated plants decreased significantly compared to that of GSH-treated plants in cultivation experiments ( and ). A noteworthy difference between cultivation experiments and positron imaging experiments is the washing step of roots in the cultivation experiment. It is likely that Cd absorbed nonspecifically to the surface of roots of DTT-treated plants and was washed out at the washing step. In the case of GSH treatment, a much lower amount of Cd was washed out than in DTT-treated plants, suggesting that GSH helps in activating tight accumulation of Cd in cell walls.
This study indicated that GSH applied to roots functions in activating Cd accumulation in cell walls of root cells in addition to activating Cd efflux from root cells. GSH operates at several points in plant roots to inhibit Cd translocation from roots to shoots. Further research is needed to elucidate the molecular mechanisms in which GSH is involved. Elucidation of the molecular mechanisms triggered by GSH will lead us to establish novel cultivation methods without gene manipulation.
TSSP_A_1188025_supplementary_material.pdf
Download PDF (217.5 KB)Acknowledgments
We express our thanks to Mr. H. Suto (Tokyo Nuclear Services Co., Ltd) for his technical assistance in producing 107Cd by irradiation. This study was supported in part by a Grant-in-Aid for Scientific Research (no. 26292183 to SN and no. 15H04578 to SF).
Supplemental data
Supplemental data for this article can be accessed here.
Additional information
Funding
References
- Codex Alimentarius 2015: Codex General Standard for Contaminants and Toxins in Food and Feed (Codex Stan 193-1995). http://www.fao.org/fileadmin/user_upload/livestockgov/documents/1_CXS_193e.pdf (May, 2015).
- Fujimaki S, Suzui N, Ishioka NS, Kawachi N, Ito S, Chino M, Nakamura S 2010: Tracing cadmium from culture to spikelet: non-invasive imaging and quantitative characterization of absorption, transport and accumulation of cadmium in an intact rice plant. Plant Physiol., 152, 1796–1806. doi:10.1104/pp.109.151035
- Guerinot ML 2000: The ZIP family of metal transporters. Biochim. Biophys. Acta, 1465, 190–198. doi:10.1016/S0005-2736(00)00138-3
- Ishikawa S, Ishimaru Y, Igura M, Kuramata M, Abe T, Senoura T, Hase Y, Arao T, Nishizawa NK, Nakanishi H 2012: Ion-beam irradiation, gene identification, and maker-assisted breeding in the development of low-cadmium rice. Proc. Natl. Acad. Sci. USA, 109, 19166–19171. doi:10.1073/pnas.1211132109
- Ito H, Iwabuchi M, Ogawa K 2003: The sugar-metabolic enzymes aldolase and triose-phosphate isomerase are targets of glutathionylation in Arabidopsis thaliana: detection using biotinylated glutathione. Plant Cell Physiol., 44, 655–660. doi:10.1093/pcp/pcg098
- Kim K, Rhee SG, Stadtman ER 1985: Nonenzymatic Cleavage of Proteins by reactive oxygen species generated by dithiothreitol and iron. J. Biol. Chem., 260, 15394–15397.
- Kuramata M, Masuya S, Takahashi Y, Kitagawa E, Inoue C, Ishikawa S, Youssefian S, Kusano T 2009: Novel cysteine-rich peptides from Digtaria ciliaris and Oryza sativa enhance tolerance to cadmium by limiting its cellular accumulation. Plant Cell Physiol., 50, 106–117. doi:10.1093/pcp/pcn175
- Mendoza-Cózatl D, Loza-Tavera H, Hernndez-Navarro A 2005: Sulfur assimilation and glutathione metabolism under cadmium stress in yeast, protists and plants. FEMS Microbiol. Rev., 29, 653–671. doi:10.1016/j.femsre.2004.09.004
- Meyer AJ, Hell R 2005: Glutathione homeostasis and redox-regulation by sulfhydryl groups. Photosynth. Res., 86, 435–457. doi:10.1007/s11120-005-8425-1
- Mou Z, Fan W, Dong X 2003: Inducers of plant systemic acquired resistance regulate NPR1 function through redox changes. Cell, 113, 935–944. doi:10.1016/S0092-8674(03)00429-X
- Nakamura S, Akiyama C, Sasaki T, Hattori H, Chino M 2008: Effect of cadmium on the chemical composition of xylem exudate from oilseed rape plants (Brassica napus L.). Soil Sci. Plant Nutr., 54, 118–127. doi:10.1111/j.1747-0765.2007.00214.x
- Nakamura S, Kondo H, Suzui N, Yin Y-G, Ishii S, Kawachi N, Rai H, Hattori H, Fujimaki S 2015: Effects of glutathione concentration in the root zone and glutathione treatment period on cadmium partitioning in oilseed rape plants. In Molecular Physiology and Ecophysiology of Sulfur, Eds. De Kok, LJ, Hawkesford, MJ, Rennenberg, H, Saito, K, Schnug, E, pp. 253–259. Springer International Publishing AG, Basel. doi:10.1007/978-3-319-20137-5_28
- Nakamura S, Suzui N, Nagasaka T et al. 2013: Application of glutathione to roots selectively inhibits cadmium transport from roots to shoots in oilseed rape. J. Exp. Bot., 64, 1073–1081. doi:10.1093/jxb/ers388
- Noctor G, Foyer CH 1998: ASCORBATE AND GLUTATHIONE: keeping active oxygen under control. Annu. Rev. Plant Physiol. Plant Mol. Biol., 49, 249–279. doi:10.1146/annurev.arplant.49.1.249
- Obata H, Umebayashi M 1999: Effects of cadmium on mineral nutrient concentrations in plants differing in tolerance for cadmium. J. Plant Nutr., 20, 97–105. doi:10.1080/01904169709365236
- Rauser WE 1995: Phytochelatins and related peptides (structure, biosynthesis, and function). Plant Physiol., 109, 1141–1149. doi:10.1104/pp.109.4.1141
- Rouhier N, Lemaire SD, Jacquot J-P 2008: The role of glutathione in photosynthetic organisms: emerging functions for glutaredoxins and glutathionylation. Annu. Rev. Plant Biol., 59, 143–166. doi:10.1146/annurev.arplant.59.032607.092811
- Sasaki A, Yamaji N, Yokosho K, Ma JF 2012: Nramp5 is a major transporter responsible for manganese and cadmium uptake in rice. Plant Cell, 24, 2155–2167. doi:10.1105/tpc.112.096925
- Uraguchi S, Fujiwara T 2012: Cadmium transport and tolerance in rice: perspectives for reducing grain cadmium accumulation. Rice, 5:5. doi:10.1186/1939-8433-5-5
- Uraguchi S, Kamiya T, Sakamoto T, Kasai K, Sato K, Nagamura Y, Yoshida A, Kyozuka J, Ishikawa S, Fujiwara T 2011: Low-affinity cation transporter (OsLCT1) regulates cadmium transport into rice grains. Proc. Natl. Acad. Sci. USA, 108, 20959–20964. doi:10.1073/pnas.1116531109
- Uraguchi S, Mori S, Kuramata M, Kawasaki A, Arao T, Ishikawa S 2009: Root-to-shoot Cd translocation via the xylem is the major process determining shoot and grain cadmium accumulation in rice. J. Exp. Bot., 60, 2677–2688. doi:10.1093/jxb/erp119
- Yamaji N, Ma JF 2014: The node, a hub for mineral nutrient distribution in graminaceous plants. Trends Plant Sci., 19, 556–563. doi:10.1016/j.tplants.2014.05.007
- Yoshihara T, Suzui N, Ishii Set al. 2013: A kinetic analysis of cadmium accumulation in a Cd hyper-accumulator fern, Athyrium yokoscense and tobacco plants. Plant Cell Environ., 37, 1086–1096. doi:10.1111/pce.12217
- Yu Q, Tang C, Chen Z, Kuo J 1999: Extraction of apoplastic sap from plant roots by centrifugation. New Phytol., 143, 299–304. doi:10.1046/j.1469-8137.1999.00454.x