ABSTRACT
Azolla (Azolla filiculoides) is a common aquatic fern that has been used successfully as a dual crop with lowland rice. It grows rapidly and has the ability to fix N2 for rice paddy. However, its ecological significance especially on greenhouse gases emissions remains unclear. To investigate the effect of azolla cover on methane (CH4) and nitrous oxide (N2O) emissions from rice paddy, a pot experiment with two treatments, control (rice plant only) and azolla cover (rice plus azolla covering on the flooding water), was carried out in Tsuruoka, Yamagata, Japan, in 2016. The results showed that the rice growth parameters, like shoot height, maximum and productive tiller numbers, and plant biomass were not significantly different between the two treatments. Dual cropping of azolla with rice significantly suppressed CH4 emissions, likely due to an increase in dissolved oxygen concentration and redox potential at the soil-water interface between flooding water and soil surface. There were significant (P < 0.05) positive correlations between CH4 flux and night respiration (CO2 emissions) between the two treatments. The cumulated CH4 emissions during the growth period until 106 days after transplanting (DAT) was significantly lower at 36.2 g C m−2 from azolla cover treatment than that from control treatment pot at 55.4 g C m−2. A prolonged nonsignificant N2O emission under the azolla cover treatment after the initial highest peak at 15 DAT was recorded due to denitrification of the nitrate in initial soil. No further N2O emissions were recorded thereafter from both treatments. Azolla cover did not affect N2O emissions from both treatments.
1. Introduction
Atmospheric concentrations of greenhouse gases (GHGs), such as carbon dioxide (CO2), methane (CH4), and nitrous oxide (N2O) have significantly increased as a result of anthropogenic activities. CH4 accounts for 15–20% of the radiative forcing with a global warming potential (GWP) of 25 times greater than carbon dioxide (CO2) on a mass basis, while N2O is 298 times more potent in heat trapping as compared to CO2 and accounts for 6–8% of the current global warming (IPCC Citation2007). According to IPCC (Citation2007), agriculture is estimated to account for 10–12% of anthropogenic emissions of GHGs worldwide, including 50% global CH4 and 60% of global N2O emissions. Rice paddies are considered as one of the most important sources of anthropogenic CH4, but they also emit N2O and the intensity of emissions is related to the nitrogen (N) fertilizer application rate (Akiyama et al. Citation2006). CH4 and N2O gases are simultaneously emitted from rice paddy soils depending on rice cultivation system, soil organic matter, soil moisture level, microbial activity, redox status, exogenous application of nitrogenous fertilizer, and organic materials (Akiyama et al. Citation2006; Snyder et al. Citation2009; Nishimura et al. Citation2011). The trade-off relationship between CH4 and N2O emissions in rice paddy fields is a strong indication that possible techniques to reduce these emissions simultaneously should be developed (Li Citation2007).
Azolla (Azolla filiculoides) is a floating aquatic fern growing in tropical and temperate freshwater ecosystems. As a result of its symbiosis with N2 fixation cyanobacteria, Anabaena azollae, within its leaf cavities, it has been cultivated in rice paddy for improving rice N nutrient in southern China and northern Vietnam as green manure for many centuries (Watanabe and Liu Citation1992; Wagner Citation1997). Even though chemical N fertilizers have substituted the role of azolla as a green manure, azolla is still cultivated by organic farmers, especially in rice-fish-azolla or rice-duck-azolla multiple eco-production systems in China and Japan (Cheng et al. Citation2015a, Citationb). Additionally, azolla is recognized to modify the physical, chemical, and biological properties of soil and the soil-water interface between flooding water and soil surface in rice fields for mobilizing fixed phosphates, retarding NH3 volatilization which accompanies the application of chemical N fertilizer, suppressing aquatic weeds in flooding rice field, and reducing evapotranspiration losses for rice production (Mandal et al. Citation1999; Cissé and Vlek Citation2003; Cheng et al. Citation2015a; Kimani et al. Citation2016; Kollah et al. Citation2016). Recently, azolla has been highlighted again for biofetilizer and biodiesel production (Bocchi and Malgioglio Citation2010; Jumadi et al. Citation2014; Brouwer et al. Citation2016; Kollah et al. Citation2016).
Previous studies have shown that azolla application in the paddies could increase (Chen et al. Citation1997; Ying et al. Citation2000) or decrease (Bharati et al. Citation2000; Ali et al. Citation2015; Singh and Strong Citation2016) CH4 emissions from rice soils. The discrepancy between these studies may be partly due to different experimental conditions, including different rice cultivars and soil types. N2O is a byproduct or an intermediate product of microbial nitrification and denitrification on the N cycling in soil-plant ecosystems (Cheng et al. Citation2004a, Citationb). There are many studies showing N2O emissions from symbiotic N2 fixation plants, such as leguminous crops and acacia trees as being larger than nonsymbiotic N2 fixation plants (Arai et al. Citation2008; Mori et al. Citation2010; Uchida and Akiyama Citation2013; Zhang et al. Citation2014). The N2O emission from soybean ecosystems could be mitigated by inoculation of high N2O reductase N-fixing rhizobium (Akiyama et al. Citation2016). As a symbiotic N2 fixing plant, how azolla affects N2O emission from rice paddy ecosystems as dual crops (rice and azolla covering on flooding water) is not well understood.
Although numerous studies on the exchange of CH4 and N2O between the rice paddies and atmosphere have been conducted from the 1980s, most of those studies place their focus on either of the two gases. To understand how azolla cover on the flooding water affects CH4 and N2O emissions simultaneously from dual cropping (rice and azolla covering on flooding water) rice paddy systems, we conducted a pot experiment in 2016 summer season in Tsuruoka, Yamagata, Japan.
2. Material and methods
2.1. Experiment site, design, and management
This research was conducted in 2016 at the Experiment Farm, Faculty of Agriculture, Yamagata University, Tsuruoka, Yamagata Prefecture, located in northeastern Japan (38°44ʹN, 139°50ʹE, 16 m elevation). According to the Japan Meteorological Agency database for Tsuruoka Meteorological Observatory (http://www.data.jma.go.jp/obd/stats/etrn/index.php), which is located inside of the Experiment Farm, Faculty of Agriculture, Yamagata University, the climatic condition during rice growth season (31st May to 15th September) in 2016 was hotter and sunnier than the historic average of 1981–2010 (). The daily average air temperature and sunshine times were 23.71°C and 6.16 h than those of historic average of 1981–2010 at 22.60°C and 5.75 h, respectively.
Figure 1. Daily sunshine time (■) and average air temperature (●) during the experiment period from 31st May to 15 September 2016 in Tsuruoka, Japan. The bold line and dish line crossed Air Temp and Sunshine time are the average values for 1981–2010 (Av. 30 years). Data were from Japan Meteorological Agency.
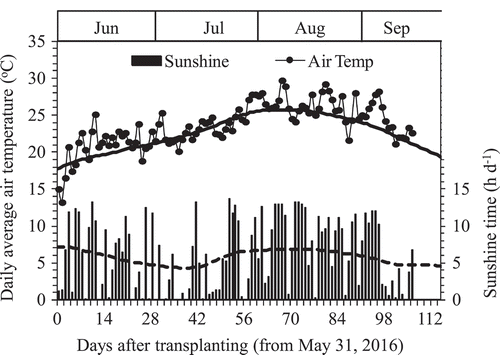
In situ pots were used with two treatments in four replications, (1) control (rice plant only, single cropping), and (2) azolla cover (rice plus azolla covering on the flooding water, dual cropping). Although azolla grows in this region during summer, rice growing season, no native azolla was available because of the harsh winter conditions. An introduced species (IRRI code FI 1001) was adopted and grown in glasshouse for this experiment (Cheng et al. Citation2010; Cheng et al. Citation2015a). Rice cultivar was Haenuki, which is a popular edible rice variety grown widely in the local area, Yamagata Prefecture.
The bulk soil used in this experiment was collected from a rice field at the University Farm and classified as an alluvial (). The soil was air-dried, sieved (5-mm mesh size), and mixed well before use. On 26 April 2016, geminated seeds were sown in a seedling tray (three seeds per cell). At three weeks after sowing, on 31 May 2016, the seedlings were transplanted (three seedlings per pot) to eight plastic pots (19.5 cm inside diameter, 27 cm height, and 0.2 cm thickness). Prior to transplanting, 6.0 kg (4.5 kg dry soil equivalent, water content was 25% in w/t) of the alluvial soil was mixed with 0.87 g of KH2PO4 and 0.87 g CO(NH2)2 and filled to each pot. The amounts of nitrogen, phosphorous, and potassium of the basal fertilizers were 0.60, 0.20, and 0.25 g pot−1, respectively. At 49 days after transplanting (DAT), we top-dressed with 0.20 g N, 0.10 g P, and 0.13 g K pot−1 by 0.43 g CO(NH2)2 and 0.43 g KH2PO4. At the end of the experiment, the total amounts of applied N, P, and K at field level were, 183.84, 89.73, and 112.88 kg per ha, respectively. The flooding water was maintained at about 5 cm depth throughout the experimental period by adding tap water. Five grams fresh weight of azolla was inoculated into the water of each of the azolla cover treatment pots after rice transplanting and its final dry weight determined prior to rice harvesting.
Table 1. Major properties of experimental paddy soil.
2.2. Measurements of plants growth
Rice height and tiller number of each plant were measured once a week beginning at eight DAT. At that time, top rice leaf greenness (SPAD value, indicating the amount of chlorophyll present) was measured using a SPAD-502 Plus chlorophyll meter (Konica Minolta Inc., Tokyo, Japan). The data from four hills were averaged for each container during all rice growth periods. Above-ground parts of rice plants were harvested at 107 DAT and the roots were thoroughly washed with water. The samples were promptly oven dried at 70°C for 48 h for dry weight calculations. The dry weights of each part for all the plants in the experiment were also determined. Much of the ears were lost due to the field mouse invasion before harvest, so dry weight of ears was not shown in this paper. Before harvest, azolla in the flooding water was also collected and oven dried at 70°C for 48 h for dry weight calculations.
2.3. Measurements of CH4 and N2O fluxes, and plants dark respiration (CO2 flux)
Seasonal variations in CH4 and N2O fluxes, and plants dark respiration (CO2 flux) from the rice pots placed in outdoor water tanks (two pots per tank) (65 cm length × 46 cm width × 32 cm depth) filled with water, were collected with a cylindrical, transparent acrylic, closed-top chamber (20.5 cm inside diameter, 100 cm height, and 0.3 cm thickness). During the sampling time of 30 min, each pot was covered with the chamber to capture gas exchanged between the pots and the atmosphere. At 0, 15, and 30 min after the chamber was placed, a gas sample of about 30 mL was drawn with a 30-mL plastic syringe through a capillary tube at the top of the chamber and injected into a 19-mL vacuum bottle with a rubber stopper and screw cap. The bottles were sent to Institute for Agro-Environmental Sciences, NARO, where CH4, N2O, and CO2 concentrations in the bottles were analyzed using an automated analysis system for three gases of CO2, CH4, and N2O. This system consists of two gas chromatographs (GC-14B, Shimadzu, Kyoto, Japan), of which one has both a thermal conductivity detector (TCD) and a flame ionization detector (FID), and the other has an electron capture detector (ECD). This system can analyze 40 samples consecutively with a modified automated headspace sampler (HSS-2B, Shimadzu, Kyoto, Japan) (Sudo Citation2006). The fluxes were calculated from the increase in the gas concentration inside the chamber per square metre per hour (Cheng et al. Citation2006; Cheng et al. Citation2008). We measured CH4, N2O, and CO2 fluxes at night time about 20:00 ~ 22:00, because the average CH4 flux from rice paddy was similar to the flux at day time between 8:00 ~ 10:00 and night time 20:00 ~ 22:00 (Cheng et al. Citation2008; Minamikawa et al. Citation2015), and the increase in CO2 concentration in the chamber in the evening samples represented plants’ night respiration. The three gases fluxes were measured once every two weeks for the first 56 DATs. Later, gas measurements were carried out after every week and terminated after 106 DATs, a day before the final rice plant harvesting.
2.4. Measurements of dissolved CO2 and CH4, and NO3--N in soil solution
Soil solution in each pot was sampled with a Rhizon soil-solution sampler (10 Rhizon SMS-MOM; Eijkelk-amp Agrisearch Equipment, Giesbeek, the Netherlands). The sampler consists of a microporous polymer tube 10 cm long (2.5 mm outside diameter × 1.5 mm inside diameter) and a PVC tube (50 cm length × 2.7 mm outside diameter × 1.0 mm inside diameter). The microporous polymer tube was inserted vertically into the soil between the rice plant and pot edge at a depth of 10–15 cm in each pot one day after rice transplanting. About 8 mL of solution was sucked out of the polymer tube with a 10 mL vacutainer (Terumo Ltd, Tokyo, Japan) to remove impurities from the tube before the main sampling. Then a 19 mL semi-vacuum bottle (filled with pure N2 gas at 0.5 atm) fitted with a rubber stopper and a screw cap was connected to the sampler tube. About 9.5 mL of soil solution was sucked into the bottle by the time the pressure in the bottle reached 1 atm. The amount of solution collected and headspace volume were determined by weighing the bottle before and after sampling. CO2 and CH4 were measured in the laboratory with a gas chromatograph (Shimadzu GC-7A) with TCD and FID detectors, respectively. Concentrations of CO2 and CH4 dissolved in the soil solution were calculated by Henry’s law according to the concentrations of CO2 and CH4 in the headspace (Cheng et al. Citation2005; Cheng et al. Citation2006). The NO3--N concentration in soil solution was analyzed by colorimetric techniques at 450 nm by using a UV-1200V spectrophotometer (Shimadzu, Japan).
2.5. Statistical analysis
Two paired-sample t-test was applied for each parameter for which significant differences were found (P < 0.05) between control and azolla cover treatments. The analysis was done with SPSS 20 (SPSS Inc., Chicago, IL USA) statistical package.
3. Results
3.1. Changes in rice plant height, tiller number, and SPAD value during entire experimental period
Rice growth period from transplanting to harvesting was about 107 DAT for both treatments (control and azolla cover). Plant height increased steadily in both setups until the grain-filling stage about 85 DAT (). At harvest, the shoot height was 78.0 and 80.0 for control and azolla cover setups, respectively. There was no significant difference in rice plant height between the two treatments ().
Table 2. The main rice growth parameters, dry weight of rice plants and azolla at harvest, and the cumulated CH4 and N2O emissions during the experimental period between two treatments of absence (Control) and presence of A. filiculoides (Azolla).
Figure 2. Changes in plant heights (A), tiller numbers of rice plants (B), and leaf color in SPAD values (C) between the rice growth on the two treatments of absence (Control) and presence of A. filiculoides (Azolla) throughout the experiment. Bars indicate standard deviation (n = 4). Arrow indicates the day of fertilizer addition.
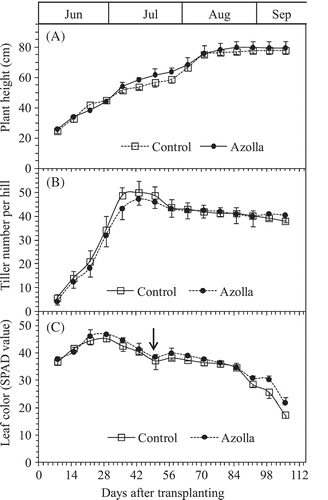
Rice tiller numbers reached a maximum at 43 DAT for both setups (). The maximum tiller numbers per hill were 50.8 for control treatment and 48.5 for the azolla cover treatment. At harvest, the productive tiller numbers per hill were 39.5 and 40.0 for control and azolla cover setups, respectively ().
Top rice leaf greenness (SPAD value) increased to maximum value until 22–29 DAT, 44.5 and 45.3 for control and azolla cover treatments, respectively, then declined slowly for both setups. Later, the leaf greenness was improved by first added fertilization on 49 DAT (). During the entire rice growth period, the SPAD value of azolla cover treatment was mostly darker than that of the control. There was a significant difference in SPAD value between the two treatments between 92–106 DAT (), with SPAD values between 28.6–17.4 for the control and 30.9–21.8 for azolla cover treatment, respectively.
3.2. Dry weight of rice plants and azolla at harvest
Dry weights of leaf, stem, and roots for the two treatments were not significant different. Leaf biomass per hill was 9.36 and 9.93 for control and azolla cover treatments, respectively. The stem biomass per hill was 57.11 for control and 56.97 for azolla cover treatments, while the roots biomass per hill was 9.75 and 10.11 for control and azolla cover treatments, respectively (). At harvest, the dry biomass of flooding azolla in the azolla cover pots was 13.60 g pot−1.
3.3. Changes in CH4 fluxes and accumulated emissions
The pattern/intensity of CH4 emissions from the pot experiment varied with the stage of rice growth (). The emission rates for the control and azolla cover treatments were relatively low and similar (0.00–7.60 mg C m−2 h−1) during the initial stage (15–57 DAT) and increased as the crop matured. The rates peaked on 71 DAT, (70.73 mg C m−2 h−1) and (52.51 mg C m−2 h−1) for control and azolla cover treatments, respectively, and decreased following ripening of the rice crop (46.38–27.71 mg C m−2 h−1) for the control and (24.69–17.25 mg C m−2 h−1) for the azolla cover treatments. While the azolla cover treatment recorded a continuous CH4 emission decrease, a second peak for the control treatment was recorded at 85 DAT. Total seasonal average CH4 emission rates were (55.4 and 36.2 g C m−2) for control and azolla cover treatments, respectively (). Variations of CH4 emissions from the two treatments in the pot experiment differed significantly between the heading (71 DAT) and maturity (106 DAT) stages.
Figure 3. Changes in the CH4 (A) and N2O (B) fluxes from the pots between the rice growth on the two treatments of absence (Control) and presence of A. filiculoides (Azolla) throughout the experiment. Bars indicate standard deviation (n = 4). Inset in (B) shows the concentration of NO3--N dissolved in soil solution at the first gas sampling day (15 DAT). Bold arrows in (A) indicate the heading days for each treatment.
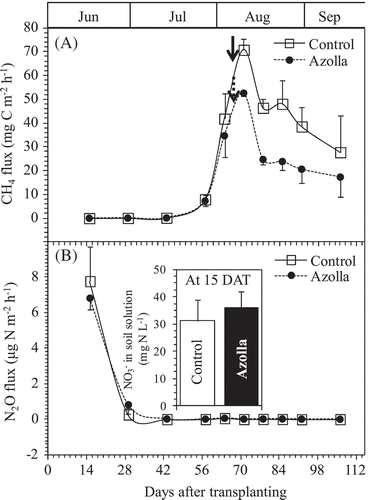
3.4. Changes in N2O fluxes and NO3--N concentrations in soil solution
The pattern/intensity of N2O emissions from the pot experiment recorded varied emission rates during the initial stage (15–29 DAT) and decreased rapidly following the development of anaerobic conditions in the soil. The emissions peaked on 15 DAT (7.77 µg N m−2 h−1) and (6.81 µg N m−2 h−1) for control and azolla cover treatments, respectively (). Variations of N2O emissions from the two treatments during 15–106 DAT were not significant. However, the azolla cover treatment showed slightly prolonged N2O emission during 15–29 DAT, 0.27 µg N m−2 h−1 for control and 0.81 µg N m−2 h−1 for the azolla cover treatment on 29 DAT. The NO3--N concentration in the solution was only detected on 15 DAT, and was 36.15 and 31.12 mg N L−1 for control and azolla cover treatments, respectively (inset .
3.5. Changes in CO2 night respiration
Night respiration (the CO2 mostly emitted from rice plant and floating azolla) ranged from 90.4 to 863.9 mg C m–2 h–1 during the entire experimental period, and this parameter varied with growth stage. The highest peak of night-time CO2 respiration was found at 10 weeks after rice transplanting for the two treatments (). The small peaks before and after the highest one were as a result of daily sunshine time (). Night-time CO2 was significantly higher at 15 DAT and from the 64 and 106 DAT (P < 0.05). The average values of CO2 respiration during the entire experimental period were 412.6 and 491.67 mg C m–2 h–1 from control and azolla cover treatments, respectively.
Figure 4. Changes in night respiration (CO2 emission) of rice plants grown in the pots between treatments of absence (Control and presence of A. filiculoides (Azolla) throughout the experiment period (A). Bars indicate standard deviation (n = 4). The daily maximum temperature and the temperature at sampling time (21:00 pm), and sunshine time on day of gases sampling were shown in (B).
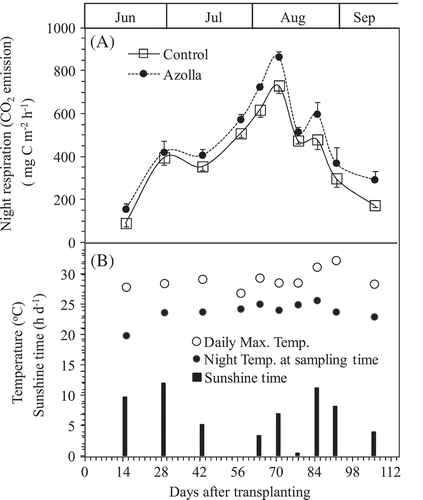
3.6. Changes in CO2 and CH4 concentrations in soil solution
Variations of soil solution CO2 concentrations between the two treatments during 15–57 DAT were not significantly different (). After the highest peak at 57 DAT for both treatments, at 64 DAT a significant soil concentration level (P < 0.05), was recorded with no further significant observations thereafter. Dissolved CH4 in the soil solution recorded nonsignificant variations between the two treatments throughout the growth period, 15–106 DAT (). However, azolla cover treatment showed lower CH4 soil solution concentrations in the first 15–43 DAT compared to control. The dissolved soil concentrations of CH4 for both treatments increased uniformly with the highest peak at 64 DAT and later stabilized. The average values of soil dissolved CO2 concentration during the experimental period were 146.0 and 153.0 µg C mL−1 for control and azolla cover treatments, respectively. While those of soil dissolved CH4 concentrations were 1.89 µg C mL−1 and 1.85 µg C mL−1 for control and azolla cover treatments, respectively.
4. Discussion
4.1. Effects of azolla cover on rice growth
Though the tiller number of control treatment during early rice growth stage and the maximum tiller number were higher than those of azolla cover treatment (), the productive tiller number at harvest was similar (). The SPAD values of control treatment were slightly higher than those of azolla cover treatment, but there were not significant in most cases, except the last two times (). The overall performance of the rice under control and azolla cover in respect to above and below ground dry weight biomasses was statistically similar (). Although we lost much of the aboveground biomass due to field mouse invasion, the results indicated that azolla cover during the single rice season did not affect rice growth. The reasons can be explained as follows. First, covering azolla was not plowed into soil during the rice growth season as biofertillzer; thus, the azolla cover treatment could not provide extra N for increasing rice growth. Second, covering azolla absorbed the nutrition elements from flooding water, but it did not compete against rice to absorb the nutrition elements from soil. Nonetheless, covering azolla as dual cropping did not compete for sunshine with rice and hence no decrease in rice growth. Related observations have been reported by Mujiyo et al. (Citation2016) for organic rice systems in Indonesia.
4.2. Effect of azolla cover on CH4 emission
The earliest research regarding the effect of azolla application on CH4 emission from rice soil ecosystems was started in 1990s. Chen et al. (Citation1997) and Ying et al. (Citation2000) reported that azolla application increased CH4 emission, likely due to the exudation of azolla root and decomposition of dead azolla, but not due to azolla transportation of CH4 in soil as rice plant did. However, the most recent reports showed that azolla cover decreased CH4 emission from rice soil ecosystems, likely due to the increase in redox potential in the root region and dissolved oxygen concentration at the soil-water interface (Ali et al. Citation2015; Singh and Strong Citation2016; Liu et al. Citation2017). In this study, we had not obviously observed the dead azolla until harvest, and even if the exudation of azolla roots contributed to the organic matter in soil surface, the contribution could have become an insignificant source of CH4 since the surface layer of submerged rice paddy always maintained oxic conditions. Hence, the possibility of the azolla covering in our experiment becoming a source for CH4 production and emission in the dual cropping azolla-rice soil ecosystem, was highly unlikely. Our results showed that CH4 fluxes occurred eight weeks after rice transplanting at 7.91 and 7.28 mg C m−2 h−1 for control and azolla cover treatments, respectively. After that, CH4 fluxes from the control treatment were always higher than those from azolla cover treatment (). The cumulated CH4 emissions during the growth period until 106 DAT (most during the periods after heading) were significantly lower at 36.2 g C m−2 from azolla cover treatment than that from control treatment pot at 55.4 g C m−2. Azolla cover decreased the seasonal CH4 emission by 34.7% compared to the control treatment without azolla cover (). This result was consistent with previous recent studies (Bharati et al. Citation2000; Ma et al. Citation2012; Ali et al. Citation2015; Liu et al. Citation2017).The cumulated CH4 emission from control treatment pot at 55.4 g C m−2 was similar to the value gathered in a field experiments in Yamagata (Itoh et al. Citation2011). Not only azolla, but also common duckweed (Lemna minor), the other floating macrophyte, significantly reduced the mean CH4 emission from flooded rice paddies in China by 20.4% (Wang et al. Citation2015). It is well known that CH4 emission from flooded rice paddy soils occurs through plant-mediated transport, ebullition, and diffusion, and that the plant-mediated transport is the dominant pathway (Nouchi et al. Citation1990). Net CH4 emission is determined by the balance between CH4 production and CH4 oxidation in rice plant-paddy soil ecosystems (Inubushi et al. Citation2003). Redox potential, which is an indirect indicator of the CH4 flux pattern from rice ecosystem (Wang et al. Citation1993), is directly related to dissolved oxygen concentration, and soils with higher redox potential could inhibit CH4 production and contribute to CH4 oxidation and hence lower CH4 emission rate (Malyan et al. Citation2016). Although we did not measure the dissolved oxygen content in the standing water and redox potential in this study, Xu et al. (Citation2017) reported 30.0–42.8 and 24.1–44.8% markedly enhanced dissolved oxygen at the soil-water interface and enhanced soil redox potential by 14.5–19.8% and 12.7–19.4% during the early and late rice growing seasons, respectively, of rice + azolla without N fertilizer treatment compared with the conventional rice without N fertilizer. Thus, for our study, the moderating effect of floating plants on CH4 emission from dual azolla-rice soil ecosystem could be reconsidered due to two main reasons. First, photosynthetically released oxygen by the floating plants into the flooding water could directly stimulate CH4 oxidation at the soil-water interface and rhizosphere of the surface layer, indirectly leading to a decrease in CH4 emission from plant-mediated transport (through the aerenchyma tissues). Second, the moderating effect on CH4 emission from dual azolla-rice soil could be attributed to the large masses of floating plants covering the flooding water surface of rice soil which could serve as a physical barrier obstructing the diffusion of CH4 from anaerobic soil to the atmosphere, which provides the other pathways for CH4 emitting to atmosphere as ebullition and diffusion (Van Der Steen et al. Citation2003). Our results showed no significant differences on rice growth parameters and dry biomass at harvest ( and ), and the soluble CH4 and CO2 concentrations in soil solutions () between control and azolla cover treatments, implying that azolla cover did not affect the CH4 production in the submerged dual cropping azolla-rice soil ecosystem. This confirmed that the decreased CH4 emission by azolla cover was due to stimulated CH4 oxidation (Bharati et al. Citation2000; Ali et al. Citation2015; Liu et al. Citation2017).
The sources of CH4 production are from both older matter (e.g., native soil organic matter, incorporated organic material such as straw and manure (Nakajima et al. Citation2016), and new matter from plant growth (e.g., root exudates and plant debris). In this pot experiment, we used light air-dried soil (at 25% w/t moisture) without any visible plant residues for cultivating single cropping rice and dual cropping azolla and rice. CH4 fluxes were not detected or were relatively low during early rice growth period until 43 DAT (), even though the amount of CH4 dissolved in the soil solution increased following the rice growth until rice heading stage (). It implied that the native soil organic matter was not the main source of CH4 production. The CH4 flux increased shapely after 43 DAT and reached to the highest peaks at heading stage for both treatments (). During the same period, the plant night respirations also recorded the highest peaks for both treatments (). Also significant positive correlations were found between CH4 flux and night respiration for both control (P < 0.05) and azolla cover (P < 0.01) treatments throughout after nine weeks of rice transplanting (). These results implied that the sources of CH4 productions from the both treatments were mostly from new matter through root exudates and plant debris, not from older matter, such as native soil organic matter and incorporated organic material, which is consistent with our previous results (Cheng et al. Citation2006; Cheng et al. Citation2008; Lou et al. Citation2008). In addition, the night respirations of azolla cover treatments were always higher than those of control treatments due to CO2 respired from both the azolla cover as dual cropping and rice (). It should be noted that the higher CO2 respiration from azolla cover treatment than control did not directly relate to different CH4 emissions between both treatments. Night CH4 emission and respired CO2 could be affected by many environmental factors, such as temperature, and sunshine time. In this experiment, the temperature at sampling time (around 21:00 pm) and daily maximum temperature at day time did not affect both night CH4 emission and respired CO2 during entire rice growth period ( and ). However, the daily sunshine time likely affected night CO2 respirations ().
4.3. Effect of azolla cover on N2O emission
To our knowledge, N2O emission from rice paddy affected by azolla cover was only reported by Chen et al. (Citation1997) and Ma et al. (Citation2012). Their results showed that azolla cover increased N2O emission from rice paddies due to N-fixation by azolla providing a source for N2O production through nitrification and denitrification, especially when the azolla died. The reason could be similar to that of N2O emissions from the other symbiotic N2 fixation plants ecosystems, such as leguminous crops and acacia trees ecosystems, which emitted more N2O to the atmosphere than nonsymbiotic N2 fixation plant ecosystems (Arai et al. Citation2008; Mori et al. Citation2010; Uchida and Akiyama Citation2013; Zhang et al. Citation2014). However, in this experiment, N2O flux was not detected or was relatively low (< 0.01 µg N m−2 h−1) at four weeks after rice transplanting from both control and azolla cover treatments (). It implied that azolla cover did not bring extra N2O flux from dual azolla and rice cropping ecosystems. The reason can be explained as discussed in the last section, as we had not obviously observed the dead azolla until harvest. Though relatively high N2O fluxes were detected at 15 DAT (first gas sampling) from both treatments at 7.77 and 6.81 µg N m−2 h−1 for control and azolla cover treatments, respectively (), the source for the high N2O can be attributed to the NO3--N in the initial soil before flooding. The NO3--N in the initial soil was 79.7 mg N kg−1 DW (). Even at 15 DAT, the NO3--N concentrations were 31.1 and 36.2 mg N L−1 in the soil solutions of control and azolla cover treatments, respectively (inset ). High NO3--N in the initial soil and high NO3--N dissolved in soil solution, and no statistical significance of NO3--N amounts and N2O fluxes between control and azolla cover treatments confirmed that the N2O flux from early rice growth period was from NO3--N denitrification in the initial soil. This result was consistent with our previous experiment (Cheng et al. Citation2006). Though there were no significant differences in N2O emissions between the control and azolla cover treatments in our study, later decomposition and/or incorporation of the covering azolla, if not removed from the pots, may increase N2O emission during the subsequent rice growth season.
5. Conclusions
To determine whether azolla cover affects CH4 and N2O emissions from dual azolla and rice cropping paddy soil, a pot experiment was carried out in Tsuruoka, Yamagata, Japan in 2016. The results showed that azolla cover did not affect rice growth, because azolla did not compete against rice to absorb the nutrition from soil and sunshine from rice canopy. Azolla cover did not affect CH4 production in the submerged rice soil, but dual cropping of azolla with rice significantly suppressed CH4 emissions, likely due to an increase in dissolved oxygen concentration and redox potential at the soil-water interface, thereby stimulating CH4 oxidation. The cumulated CH4 emissions during the growth period until 106 DAT (most during the periods after heading) were significantly lower at 36.2 g C m−2 from azolla cover treatments than that from control treatments pot at 55.4 g C m−2. Azolla cover decreased the seasonal CH4 emission at 34.7% compared to the control treatment without azolla cover. A prolonged nonsignificant N2O emission under the azolla cover treatment after the initial highest peak at 15 DAT was recorded due to denitrification of the nitrate in initial soil. No further N2O emissions were recorded thereafter from both treatments. Azolla cover did not affect N2O emissions from both treatments. It should be noted that our results are based on pot experiment, field studies should be carried out in future.
Acknowledgments
First author, Samuel Munyaka KIMANI, appreciates the African Business Education Initiative program for supporting his studies in Japan.
Additional information
Funding
References
- Akiyama H, Takada-Hoshino Y, Itakura M, Shimomura Y, Wang Y, Yamamoto A, Tago K, Nakajima Y, Minamisawa K, Hayatsu M 2016: Mitigation of soil N2O emission by inoculation with a mixed culture of indigenous Bradyrhizobium diazoefficiens. Sci. Rep., 6, Article number: 32869, doi:10.1038/srep32869.
- Akiyama H, Yan X, Yagi K 2006: Estimations of emission factors for fertilizer-induced direct N2O emissions from agricultural soils in Japan: summary of available data. Soil Sci. Plant Nutr., 52, 774–787. doi:10.1111/j.1747-0765.2006.00097.x.
- Ali MA, Kim PJ, Inubushi K 2015: Mitigating yield-scaled greenhouse gas emissions through combined application of soil amendments: a comparative study between temperate and subtropical rice paddy soils. Sci. Total Environ., 529, 140–148. doi:10.1016/j.scitotenv.2015.04.090.
- Arai S, Ishizuka S, Ohta S, Ansori S, Tokuchi N, Tanaka N, Hardjono A 2008: Potential N2O emissions from leguminous tree plantation soils in the humid tropics. Global Biogeochem. Cycl., 22, GB2028. doi:10.1029/2007GB002965.
- Bharati K, Mohanty SR, Singh DP, Rao VR, Adhya TK 2000: Influence of incorporation or dual cropping of Azolla on methane emission from a flooded alluvial soil planted to rice in eastern India. Agric. Ecosyst. Environ., 79, 73–83. doi:10.1016/S0167-8809(99)00148-6.
- Bocchi S, Malgioglio A 2010: Azolla-Anabaena as a biofertilizer for rice paddy fields in the Po Valley, a temperate rice area in northern Italy. Int. J. Agron., 2010, Article ID 152158, doi:10.1155/2010/152158.
- Brouwer P, Van Der Werf A, Schluepmann H, Reichart G-J, Nierop KGJ 2016: Lipid yield and composition of Azolla filiculoides and the implications for biodiesel production. BioEnergy Res., 9, 369–377. doi:10.1007/s12155-015-9665-3.
- Chen GX, Huang GH, Huang B, Yu KW, Wu J, Xu H 1997: Nitrous oxide and methane emissions from soil-plant systems. Nutr. Cycl. Agroecosyst., 49, 41–45. doi:10.1023/A:1009758900629.
- Cheng W, Okamoto Y, Takei M, Tawaraya K, Yasuda H 2015a: Combined use of Azolla and loach suppressed weed Monochoria vaginalis and increased rice yield without agrochemicals. Org. Agric., 5, 1–10. doi:10.1007/s13165-015-0097-3.
- Cheng W, Sakai H, Hartley AE, Yagi K, Hasegawa T 2008: Increased night temperature reduces the stimulatory effect of elevated carbon dioxide concentration on methane emission from rice paddy soil. Glob. Change Biol., 14, 644–656. doi:10.1111/j.1365-2486.2007.01532.x.
- Cheng W, Sakai H, Matsushima M, Yagi K, Hasegawa T 2010: Response of Azolla filiculoides, a floating aquatic fern, to elevated CO2, temperature, and phosphorus levels. Hydrobiologia., 656, 5–14. doi:10.1007/s10750-010-0441-2.
- Cheng W, Takei M, Sato C, Kautsar K, Sasaki Y, Sato S, Tawaraya K, Yasuda H 2015b: Combined use of Azolla and loach suppressed paddy weeds and increased organic rice yield: second season results. J. Wetlands Environ. Manag., 3, 1–13.
- Cheng W, Tsuruta H, Chen GX, Akiyama H, Yagi K 2004a: N2O and N2 production potential in various Chinese agricultural soils by denitrification. Soil Sci. Plant Nutr., 50, 909–915. doi:10.1080/00380768.2004.10408553.
- Cheng W, Tsuruta H, Chen GX, Yagi K 2004b: N2O and NO production in various Chinese agricultural soils by nitrification. Soil Biol. Biochem., 36, 953–963. doi:10.1016/j.soilbio.2004.02.012.
- Cheng W, Yagi K, Sakai H, Kobayashi K 2006: Effects of elevated atmospheric CO2 concentrations on CH4 and N2O emission from rice soil: an experiment in controlled-environment chambers. Biogeochemistry., 77, 351–373. doi:10.1007/s10533-005-1534-2.
- Cheng W, Yagi K, Xu H, Sakai H, Kobayashi K 2005: Influence of elevated concentrations of atmospheric CO2 on CH4 and CO2 entrapped in rice-paddy soil. Chem. Geol., 218, 15–24. doi:10.1016/j.chemgeo.2005.01.016.
- Cissé M, Vlek PLG 2003: Influence of urea on biological N2 fixation and N transfer from Azolla intercropped with rice. Plant Soil., 250, 105–112. doi:10.1023/A:1022830423154.
- Inubushi K, Cheng W, Aonuma S, Hoque MM, Kobayashi K, Miura S, Kim HY, Okada M 2003: Effects of free-air CO2 enrichment (FACE) on CH4 emission from a rice paddy field. Glob. Change Biol., 9, 1458–1464. doi:10.1046/j.1365-2486.2003.00665.x.
- IPCC 2007: Climate change 2007 mitigation of climate change, contribution of working group III to the fourth assessment report of the intergovernmental panel on climate change. In Intergovernmental Panel on Climate Change, Ed, Metz B, Davidson O, Bosch P, Dave R, pp. 851. Cambridge University Press, Cambridge.
- Itoh M, Sudo S, Mori S et al. 2011: Mitigation of methane emissions from paddy fields by prolonging midseason drainage. Agr. Ecosyst. Environ., 141, 359–372. doi:10.1016/j.agee.2011.03.019.
- Jumadi O, Hiola SF, Hala Y, Norton J, Inubushi K 2014: Influence of Azolla (Azolla microphylla Kaulf.) compost on biogenic gas production, inorganic nitrogen and growth of upland kangkong (Ipomoea aquatica Forsk.) in a silt loam soil. Soil Sci. Plant Nutri., 60, 722–730. doi:10.1080/00380768.2014.942879.
- Kimani SM, Kanno T, Tawaraya K, Cheng W 2016: Influence of phosphorous and flooding water depth on Azolla growth and its significance in mitigating evapotranspiration. Atstract of the Annual Meetings, Jan. Soc. Soil Sci. Plant Nutri., 62, 173–173.
- Kollah B, Patra AK, Mohanty SR 2016: Aquatic microphylla Azolla: a perspective paradigm for sustainable agriculture, environment and global climate change. Environ. Sci. Pollut. Res., 23, 4358–4369. doi:10.1007/s11356-015-5857-9.
- Li C 2007: Quantifying greenhouse gas emissions from soils: scientific basis and modeling approach. Soil Sci. Plant Nutr., 53, 344–352. doi:10.1111/j.1747-0765.2007.00133.x.
- Liu J, Xu H, Jiang Y, Zhang K, Hu Y, Zeng Z 2017: Methane emissions and microbial communities as influenced by dual cropping of azolla along with early rice. Sci. Rep., 7, 40635. doi:10.1038/srep40635.
- Lou Y, Inubushi K, Mizuno T, Hasegawa T, Lin Y, Sakai H, Cheng W, Kobayashi K 2008: CH4 emission with differences in atmospheric CO2 enrichment and rice cultivars in a Japanese paddy soil. Glob. Change Biol., 14, 2678–2687.
- Ma Y, Tong C, Wang W, Zeng C 2012: Effect of Azolla on CH4 and N2O emissions in Fuzhou Plain paddy fields. Chinese Journal of Eco-Agriculture., 20, 723−727 Chinese with English summary. doi:10.3724/SP.J.1011.2012.00723.
- Malyan SK, Bhatia A, Kumar A, Gupta DK, Singh R, Kumar SS, Tomer R, Kumar O, Jain N 2016: Methane production, oxidation and mitigation: a mechanistic understanding and comprehensive evaluation of influencing factors. Sci. Total Environ., 572, 874–896. doi:10.1016/j.scitotenv.2016.07.182.
- Mandal B, Vlek PLG, Mandal LN 1999: Beneficial effects of blue-green algae and Azolla, excluding supplying nitrogen, on wetland rice fields: a review. Biol. Fert. Soils., 28, 329–342. doi:10.1007/s003740050501.
- Minamikawa K, Tokida T, Sudo S, Padre A, Yagi K 2015: Guidelines for Measuring CH4 and N2O Emissions from Rice Paddies by a Manually Operated Closed Chamber Method. National Institute for Agro-Environmental Sciences, Tsukuba, Japan.
- Mori T, Ohta S, Ishizuka S, Konda R, Wicaksono A, Heriyanto J, Hardjono A 2010: Effects of phosphorus addition on N2O and NO emissions from soils of an Acacia mangium plantation. Soil Sci. Plant Nutr., 56, 782–788. doi:10.1111/j.1747-0765.2010.00501.x.
- Mujiyo SBH, Hanudin E, Widada J, Syamsiyah J 2016: Methane emission on organic rice experiment using Azolla. Int. J. App. Env. Sci., 11, 295–308.
- Nakajima M, Cheng W, Tang S, Hori Y, Yaginuma E, Hattori S, Hanayama S, Tawaraya K, Xu X 2016: Modeling aerobic decomposition of rice straw during off-rice season in an Andisol paddy soil in a cold temperate region, Japan: effects of soil temperature and moisture. Soil Sci. Plant Nutr., 62, 90–98. doi:10.1080/00380768.2015.1121116.
- Nishimura S, Akiyama H, Sudo S, Fumoto T, Cheng W, Yagi K 2011: Combined emission of CH4 and N2O from a paddy field was reduced by preceding upland crop cultivation. Soil Sci. Plant Nutr., 57, 167–178. doi:10.1080/00380768.2010.551346.
- Nouchi I, Mariko S, Aoki K 1990: Mechanism of methane transportation from the rhizosphere to the atmosphere through rice plants. Plant Physiol., 94, 59−66. doi:10.1104/pp.94.1.59.
- Singh JS, Strong PJ 2016: Biologically derived fertilizer: a multifaceted bio-tool in methane mitigation. Ecotoxicol. Environ. Saf., 124, 267–276. doi:10.1016/j.ecoenv.2015.10.018.
- Snyder CS, Bruulsema TW, Jensen TL, Fixen PE 2009: Review of greenhouse gas emissions from crop production systems and fertilizer management effects. Agric. Ecosyst. Environ., 133, 247–266. doi:10.1016/j.agee.2009.04.021.
- Sudo S 2006: Method and instrument for measuring atmospheric gas. Industrial Property Digital Library, Patent of Japan (no. 2006–275844).
- Uchida Y, Akiyama H 2013: Mitigation of postharvest nitrous oxide emissions from soybean ecosystems: a review. Soil Sci. Plant Nutr., 59, 477–487. doi:10.1080/00380768.2013.805433.
- Van Der Steen NP, Nakiboneka P, Mangalika L, Ferrer AVM, Gijzen HJ 2003: Effect of duckweed cover on greenhouse gas emissions and odour release from waste stabilisation ponds. Water Sci.Technol., 48, 341–348.
- Wagner GM 1997: Azolla: a review of its biology and utilization. Bot. Rev., 63, 1–26. doi:10.1007/BF02857915.
- Wang C, Li S, Lai DYF, Wang W, Ma Y 2015: The effect of floating vegetation on CH4 and N2O emissions from subtropical paddy fields in China. Paddy Water Environ., 13, 425–431. doi:10.1007/s10333-014-0459-6.
- Wang ZP, DeLaune RD, Patrick WH Jr., Masscheleyn P 1993: Soil redox and pH effects on methane production in flooded rice soil. Soil Sci. Soc. Am. J., 57, 382–385. doi:10.2136/sssaj1993.03615995005700020016x.
- Watanabe I, Liu CC 1992: Improving nitrogen-fixing systems and integrating them into sustainable rice farming. Plant Soil., 141, 57–67. doi:10.1007/BF00011310.
- Xu GC, Liu LX, Wang QS, Yu XC, Hang YH 2017: Integrated rice-duck farming mitigates the global warming potential in rice season. Sci. Total Environ., 575, 58–66.
- Ying Z, Boeckx P, Chen GX, Cleemput OV 2000: Influence of Azolla on CH4 emission from rice fields. Nutr. Cycl. Agroecosyst., 58, 321–326. doi:10.1023/A:1009871308968.
- Zhang W, Zhu X, Luo Y, Rafique R, Chen H, Huang J, Mo J 2014: Responses of nitrous oxide emissions to nitrogen and phosphorus additions in two tropical plantations with N-fixing vs. non-N-fixing tree species. Biogeosciences., 11, 4941–4951. doi:10.5194/bg-11-4941-2014.