ABSTRACT
The nonexchangeable potassium (neK) content of 178 agricultural soils in Japan was determined by subtracting the amount of K extracted with 1 mol L−1 ammonium acetate, i.e., exchangeable K (exK) from that extracted with boiling 1 mol L−1 HNO3. The statistical relationships between the neK content and physico-chemical properties of the soils were examined to investigate the factors controlling neK content. The neK content of agricultural soils in Japan ranged from 0 to 1120 mg kg−1 with an arithmetic mean and median of 303 and 255 mg kg−1, respectively. It showed a significant positive correlation with the total K content, fixed ammonium content, and silt content (p < 0.01) and a significant negative correlation with Alo+1/2 Feo content and total carbon content (p < 0.01). These results suggest that the controlling factors of neK are mainly the total K content and 2:1 type phyllosilicates such as mica and vermiculite, with the indirect negative influence of organic matter and amorphous materials. Terrestrial Regosols, Brown Lowland soils, and Dark Red soils had relatively high neK contents. In contrast, Andosols, Wet Andosols, and Volcanogenous Regosols had relatively low neK contents. The neK content showed no significant correlation with exK content, suggesting that neK is a moderately to slowly available fraction of soil K, which is independent of exK. In conclusion, evaluation of nonexchangeable K in combination with exchangeable K would enhance the rational management of agricultural soils in terms of K fertility by taking account of longer term K-supplying power of soils.
1. Introduction
Potassium (K) is an essential element that is mandatory for all plants’ growth (Brouder Citation2011). Potassium plays a very important role in stomatal movement, water retention, and enhancement of enzyme activity (Marschner Citation1995). Therefore, deficiencies of potassium cause inhibition of plant growth and a decrease of crop yield (Usherwood Citation1985).
Potassium is the seventh most abundant element in the earth’s crust. It is also very abundant in soil, and its content ranges from 0.4 to 30 g K kg−1 with a median value of 14 g K kg−1 (Bowen Citation1979; Helmke and Sparks Citation2000). It is mainly found in soil minerals such as feldspar and mica. In general, plants mainly utilize K that is electrostatically bound to the surfaces of minerals and organic matters, i.e., readily available K called as exchangeable K (exK). On the other hand, it is known that plants also take up K bound more strongly to minerals than exK (Moritsuka et al. Citation2003; Mitsios and Rowell Citation1987), which is basically held between adjacent tetrahedral layers of dioctahedral and trioctahedral micas, vermiculites, and intergrade clay minerals and not bonded covalently within the crystal structures of soil mineral particles (Martin and Sparks Citation1985; Kirkman et al. Citation1994). This fraction of K, regarded as moderately to slowly available K for plants, is called as nonexchangeable K (neK). It should be noted that plants acquire significant proportions or even the majority of their K requirement from this pool (Meyer and Jungk Citation1993, Cox et al. Citation1999, Moritsuka et al. Citation2003; Moritsuka et al. Citation2004).
To evaluate exK content, extraction method with 1 mol L−1 ammonium acetate has been used most intensively as a standard method. On the other hand, to evaluate neK content, many extraction methods have been used such as boiling HNO3, hot HCl, Na-tetraphenylboron with EDTA, and ion-exchange resins such as H- and Ca-saturated resins (Helmke and Sparks Citation2000). Among these methods, the boiling 1 mol L−1 HNO3 method has been used widely to estimate the amount of soil neK (Wood and DeTurk Citation1941; Pratt Citation1965; Schmitz and Pratt Citation1953; Helmke and Sparks Citation2000), as the amount of K extracted by this method has proved a good predictor of plant-available K (Liu and Bates Citation1990; Wood and Schroeder Citation1992).
Although the neK content of agricultural soils would be very important in estimating soil K availability for rational agricultural management, it has not been studied comprehensively for wide varieties of soil in Japan – in contrast to exK content, which has been more widely studied. Furthermore, the factors controlling soil neK content have not been comprehensively investigated in Japan under humid temperate conditions combined with the strong influence of volcanic activities.
The objectives of this research were, therefore, (1) to evaluate the neK content of agricultural soils collected from all over Japan, (2) to investigate the relationship between the neK content and physicochemical properties of the soils, and (3) to investigate the relationship between their neK content and exK content.
2. Materials and methods
2.1. Soil samples
Analysis was conducted on 178 soil samples collected from the surface layer (0–15 cm) of agricultural fields from 38 out of 47 prefectures of Japan, from Hokkaido to Okinawa (). A total of 95 samples were collected from paddy fields and 83 from upland fields. The number of samples corresponded to about one per 275 and 261 km2 for paddy and upland, respectively. The soils investigated were classified into 16 soil types (Cultivated soil classification committee Citation1995). The numbers of samples for each soil type and land use are listed in . The soil samples used in the present study were thought to be adequately representative of agricultural soils in Japan, as discussed by Yanai et al. (Citation2012), and information on the content and availability of some elements of the soil samples has been already reported (Sano et al. Citation2004; Yamada et al. Citation2009; Yamada et al. Citation2013; Yanai et al. Citation2012; Yanai et al. Citation2013; Yanai et al. Citation2015; Yanai et al. Citation2016). Soil samples were air-dried, disaggregated using a porcelain pestle and mortar, and sieved to <2 mm. A portion of each sample was further finely ground for specific analysis.
Table 1. Number of samples in relation to soil type and land use.
2.2. Analytical methods
2.2.1. Determination of neK content in soil
The neK content in soils was determined using the 1 mol L−1 hot HNO3 extraction method developed by Helmke and Sparks (Citation2000). A 2.5 g of finely ground soil sample was heated gently with 25 mL of 1 mol L−1 nitric acid (HNO3) in an Erlenmeyer flask on a hot plate for 15 min after boiling started. After cooling for 5 min, the sample was filtrated and the extract was diluted to 100 mL with 0.1 mol L−1 HNO3. The K concentration of the extract solution was determined by atomic absorption spectrometer (AA-6200; Shimadzu, Kyoto, Japan). As the amount of K in the extract can be regarded as the sum of exchangeable K (exK) and neK, the amount of neK was calculated by subtracting the amount of K extracted with 1 mol L−1 ammonium acetate (NH4OAc), i.e., the exK, from the amount of K in the HNO3 extract.
2.2.2. Determination of selected physicochemical properties of the soils
Selected physicochemical properties of the soil were analyzed to investigate the relationships with the neK contents. For the content of total K, the data from Yanai et al. (Citation2012) were used. In the analysis, a 100 mg finely ground soil sample was decomposed with a mixture of HNO3, hydrofluoric acid (HF), and perchloric acid (HClO4) in a Teflon beaker on a hot plate, and heated until strong fumes of HClO4 were produced. To the resultant solution, 1 mL of concentrated HNO3 was added, and the solution was filled up to 50 mL with deionized water. The concentration of K in the prepared solution was determined by the atomic absorption spectrometer (AA-6200; Shimadzu, Kyoto, Japan). The exK content was determined by NH4OAc extraction method. Namely, a 5.0 g air-dried soil sample was shaken with 25 mL of 1 mol L−1 NH4OAc for 30 min in a 50 mL centrifuge tube with a reciprocal shaker. The suspension was centrifuged and the supernatant was collected by filtration. These processes were repeated three times. Approximately, 75 mL of solution collected as supernatant was brought up to 100 mL with 1 mol L−1 NH4OAc, and the concentration of K in the prepared solution was determined by the atomic absorption spectrometer (AA-6200; Shimadzu, Kyoto, Japan). For the fixed NH4+-N, the data from Sano et al. (Citation2004) were mainly used. Namely, the content was determined by the method of Silva and Bremner (Citation1966). A 0.5 g of air-dried soil was ground (<0.25 mm), boiled with 10 mL of alkaline KOBr solution for 5 min, and washed with a 0.25 mol L−1 KCl solution to remove exchangeable NH4+-N and organic nitrogen compounds. Fixed NH4+-N was then extracted with a solution of 5 mol L−1 HF in 1 mol L−1 HCl with 24-h reciprocal shaking, and the amount of extracted NH4+-N was determined by the steam-distillation method (Sano et al. Citation2004). Total carbon (C) content was determined by the dry combustion method (Sumigraph NC analyzer NC-800, Sumika Chem. Anal. Service, Tokyo). The contents of oxalate-extractable aluminum (Alo), iron (Feo), and silicon (Sio) were determined by inductively coupled plasma-atomic emissions spectroscopy (SPS1500, SEIKO, Chiba, Japan) after extraction with a 0.2 mol L−1 acid ammonium oxalate solution (pH 3) for 4 h in the darkness (Blakemore et al. Citation1987). The sand (2 mm to 20 µm), silt (20 to 2 µm), and clay (<2 µm) contents were determined by the sieving and pipetting methods after organic matter removal by oxidation with hydrogen peroxide and ultrasonic dispersion.
2.2.3. Identification of clay minerals by XRD analysis
To investigate the effect of clay mineral composition on neK content, the clay mineralogy of each soil was determined. A 0.1 g of clay fraction was used for XRD analysis (Miniflex 300, Rigaku, Tokyo) with Ni-filtered Cu Kα radiation produced at 30 kV and 15 mA. The X-ray diffraction patterns were obtained from the clays oriented on glass slides by sedimentation after the clay suspension was saturated with Mg2+. The slides were step-scanned from 2° to 15° (2θ) in steps of 0.02° at a scan speed of 2° min−1. From XRD analysis data, the relative proportions of the peak areas of 0.7-, 1.0-, and 1.4-nm spacings were evaluated using PeakFit software ver. 4.12 (SeaSolve Software Inc Citation2003).
The amounts of clay minerals with 0.7-, 1.0-, and 1.4-nm peaks of each soil were calculated as the products of relative peak areas of 0.7-, 1.0-, and 1.4-nm peaks and crystalline clay content. Here, crystalline clay content was calculated as the difference between total clay content and amorphous clay content calculated as the sum of allophanic material content (mostly allophane and imogolite: estimated by a method of Parfitt and Wilson Citation1985), Al hydroxide content (estimated from Alo not included in allophanic materials and having a composition of Al(OH)3), and ferrihydrite content (estimated from Feo having a composition of 5Fe2O3 9H2O).
2.3. Statistical analyses
Descriptive statistics were calculated for the overall dataset of the neK, exK, and total K contents and selected physicochemical properties of the soils. A correlation analysis was carried out among the neK, exK, and total K contents and selected physicochemical properties of the soils.
3. Results and discussion
3.1. Descriptive statistics of the neK content of agricultural soils in Japan
shows the descriptive statistics of the K-related properties of agricultural soils in Japan, i.e., the median, arithmetic mean, maximum, minimum, and coefficient of variation of exK, neK, hot HNO3 extractable K, and total K contents. The exK content ranged from 43 to 1304 mg kg−1 with a median and arithmetic mean of 209 and 262 mg kg−1, respectively. The hot HNO3 extractable K content ranged from 117 to 1822 mg kg−1 with the median and arithmetic mean of 493 and 564 mg kg−1, respectively. Accordingly, the neK content, which was calculated by subtracting the exK content from the hot HNO3, extractable K ranged from 0 to 1120 mg kg−1 with a median and arithmetic mean of 255 and 303 mg kg−1, respectively. Here, only two samples had negative values and their neK values were evaluated as zero. The arithmetic mean of 303 mg kg−1 was almost similar to the reported values of 300 mg kg−1 for 20 Japanese agricultural soils (Moritsuka et al. Citation2003), and was relatively higher than the reported values of 79 mg kg−1 for 18 upland agricultural soils from the southeast coast and peninsular Thailand, where highly weathered upland soils deficient in K-bearing minerals are dominant (Darunsontaya et al. Citation2010). The arithmetic mean of neK (303 mg kg−1) was slightly higher than the arithmetic mean of exK (262 mg kg−1), which was in accordance with the report that nonexchangeable K content is substantially larger than the exchangeable K content in many soils (Brouder Citation2011).
Table 2. Median, arithmetic mean, maximum, minimum and coefficient of variation of exchangeable K (exK), nonexchangeable K (neK), hot HNO3 extractable K, and total K content of agricultural soils in Japan.
The total-K content ranged from 4.30 to 32.3 g kg−1 with a median and arithmetic mean of 15.1 and 15.6 g kg−1, respectively (Yanai et al. Citation2012). The relative ratios of exK and neK to total-K were, therefore, calculated to be 1.7 and 1.9%, respectively. This result directly indicates that the neK content in soil was similar to or slightly greater than the exK content, suggesting the significant importance of neK for soil K supply to plants, even though the availability of neK would be lower than that of exK. It also suggests that available K corresponded to only several percent of the total-K content in soil as reported by several authors (Brouder Citation2011; Sharpley Citation1989).
3.2. Estimation of the controlling factors of the neK content in soil
shows the correlation coefficients between the neK content and selected physico-chemical properties of the soils. The neK content showed significant positive correlations with the total K content, fixed ammonium content, and silt content (p < 0.01), and significant negative correlations with the Alo+1/2Feo content and total carbon content (p < 0.01). A positive correlation with total K suggested that the neK content is associated with K-bearing minerals, and the negative correlation with the Alo+1/2Feo content as an index of the content of amorphous minerals and total carbon content as an index of organic matter content indicated that neK is released predominantly from crystalline minerals instead of amorphous minerals or organic matter. Significant positive correlations with fixed ammonium (p < 0.01) strongly supported the generally accepted hypothesis that neK is mainly held on the edge of 2:1-type phyllosilicates such as mica and vermiculite (Brouder Citation2011). The fact that there was no significant correlation between the neK and clay content suggested that the type of clay minerals is more important than the total amount of clay minerals. In the case of Japan, the content of amorphous clay minerals varies largely depending on region due to different degrees of historical deposition of volcanic ash. Furthermore, the significant positive correlation between neK and silt content may suggest that K-bearing minerals in the silt fraction also contributed considerably to the neK content.
Table 3. Correlation coefficients between nonexchangeable K (neK) content and selected physicochemical properties of the soils.
3.3. Relationship between neK content and clay mineralogy
Furthermore, to investigate the effect of clay mineralogy on neK, correlation analysis was carried out between the neK content and the amounts of clay minerals with 0.7-, 1.0-, and 1.4-nm peaks by XRD analysis. The amount of the sum of clay minerals with 1.4-nm peaks showed significant positive correlations with the neK content (r = 0.34; p < 0.01) followed by the amounts of clay minerals with 1.0-nm (r = 0.33; p < 0.01) peaks. This result supports the notion that neK is considerably determined by 2:1-type phyllosilicates such as mica and vermiculite (Martin and Sparks Citation1985). However, the content of neK also showed significant positive correlation with the amount of clay minerals with 0.7-nm peak (r = 0.28; p < 0.01). This may be because soils rich in crystalline minerals generally contain 0.7-nm minerals in addition to 1.0- and 1.4-nm ones in the case of moderate weathering conditions in Japanese humid temperate climate.
3.4. Nonexchangeable K (neK) content of agricultural soils with reference to soil type
Evaluation of the neK content of agricultural soils in Japan with reference to soil type would be valuable in order to make the best use of the information discussed above from the standpoint of agricultural and environmental management.
shows the arithmetic mean of the neK content, neK/total-K ratio, exK content, exK/total-K ratio, and total-K content of the soils with reference to soil type. It indicates that neK content varied among soil types; i.e., it was relatively high for Terrestrial Regosols (753 mg kg−1), Brown Lowland soils (525 mg kg−1), and Dark Red soils (451 mg kg−1), and relatively low for Andosols (110 mg kg−1), Wet Andosols (132 mg kg−1), and Volcanogenous Regosols (141 mg kg−1). Terrestrial Regosols, Brown Lowland soils, and Dark Red soils with high neK contents tended to have relatively high silt and fixed ammonium content. These soils would have high content of 2:1-type phyllosilicates such as mica and vermiculite. On the other hand, Andosols, Wet Andosols, and Volcanogenous Regosols with low neK tended to have relatively high total-C content and Alo+1/2Feo content. These soils are mainly composed of amorphous minerals. These results suggest that soil neK content could be estimated based on the soil type.
Table 4. Arithmetic mean of nonexchangeable K (neK), neK/Total-K ratio, exchangeable K (exK), exK/Total-K ratio, and total K content of agricultural soils in Japan with reference to soil type.
It should be also noted that volcanic soils such as Andosols have considerable amounts of neK (though less than the other soils) in spite of the fact that those soils are mainly derived from volcanic ash or erupted materials that originally contain little 2:1-type phyllosilicate except for basaltic ones. This may suggest that Aeolian dust-containing mica minerals cover a wide range of Japanese soils and affect soil formation and soil mineralogical characteristics, as reported by Nakao et al. (Citation2015).
3.5. Nonexchangeable K (neK) content of agricultural soils with reference to region
shows the arithmetic mean of the neK, neK/total-K ratio, exK, exK/total-K ratio, and total-K contents of the soils for each of nine regions in Japan. The neK contents were relatively high for the Kinki (542 mg kg−1), Hokuriku-Chubu (426 mg kg−1), and Shikoku (405 mg kg−1) regions and relatively low for the Kanto (173 mg kg−1), Kyushu (239 mg kg−1), and Hokkaido (248 mg kg−1) regions.
Table 5. Arithmetic mean of nonexchangeable K (neK), neK/Total-K ratio, exchangeable K (exK), exK/Total-K ratio, and total K content of agricultural soils in Japan with reference to region.
At the Kinki and Hokuriku-Chubu regions with relatively high neK contents, granite is one of the dominant parent materials, i.e., about 11.3 and 16.1%, respectively (The Geological Survey of Japan Citation1992). As granite contains mica as one of its primary minerals, it is reasonable that soils in these regions tend to have higher neK contents. On the other hand, at the Kanto, Kyushu, and Hokkaido regions with relatively low neK contents, volcanic ash and volcanic materials are widely distributed, as shown in . As volcanic ash is mainly composed of amorphous minerals with scant crystalline minerals, it is reasonable that soils in these regions tend to have low neK content. Thus, there was a clear regional trend of soil neK contents reflecting their parent materials.
3.6. Relationship between neK content and exK content of agricultural soils: implications for the management of K status in agricultural soils
shows the relationship between the contents of neK and exK. The neK content showed no significant correlation with the exK content, and the contribution of the neK and exK contents to the sum of neK and exK varied among soil types (). For example, the contribution of neK was relatively high for Lowland soils (Lowland Paddy soils, Gley Lowland soils, Gray Lowland soils, and Brown Lowland soils), Upland soils (Gray Upland soils and Brown Forest soils), and Red-Yellow soils (Red soils and Yellow soils) while contribution of the neK was relatively low for Andosols (Wet Andosols, Non-allophanic Andosols, and Andosols) and Volcanogenous Regosols. Thus, the tendency of the neK content differed from the exK content among soil types, suggesting that neK has different importance as a part of available K among different soil types. These facts also suggest that we should take account of both neK and exK to evaluate soil K availability. Namely, soils with relatively high neK content are expected to supply K in the long term, so K fertilization may be required at an earlier stage of cultivation. On the other hand, soils with relatively low neK content are not expected to supply K in the long term, so we may need to add K as a fertilizer as exK decreases. It should be also noted, however, that volcanic glasses nonextractable to boiling 1 mol L−1 HNO3 but relatively vulnerable to weathering may also contribute to soil available K in some specific cases, as implicated by Yamada and Shoji (Citation1982).
Figure 2. Relationship between the content of exchangeable K (exK) and nonexchangeable K (neK). Andosols (53 samples): Wet Andosols, Non-allophanic Andosols, and Andosols. Lowland soils (96): Lowland Paddy soils, Gley Lowland soils, Gray Lowland soils, and Brown Lowland soils. Red Yellow soils (10): Red soils and Yellow soils. Dark Red soils (2). Terrestrial Regosols (2). Regosols (4): Sand-dune Regosols and Volcanogenous Regosols. Peat soils (8). Upland soils (3): Gray Upland soils and Brown Forest soils.
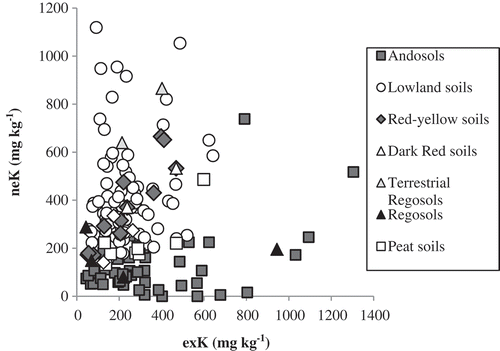
Finally, this information can be useful to establish a K management strategy to reduce the risk of radiocesium (137Cs) in soil for agricultural production by controlling the transfer factor of Cs from the soil to plants, because it enables us to estimate the buffering power of neK in maintaining exK content, which directly leads to the reduction of the effective Cs availability in soils (Eguchi et al. Citation2015; Kondo et al. Citation2015).
4. Conclusion
The neK content of agricultural soils in Japan ranged from 0 to 1120 mg kg−1 with a median and arithmetic mean of 255 and 303 mg kg−1, respectively. It had a significant positive correlation with the total K content, fixed ammonium content, and silt content (p < 0.01) and a significant negative correlation with Alo+1/2Feo content and total carbon content (p < 0.01), suggesting that the controlling factors of neK are mainly the total K content and 2:1-type phyllosilicates such as mica and vermiculite. The neK content was relatively high for Terrestrial Regosols, Brown Lowland soils, and Dark Red soils, and relatively low for Andosols, Wet Andosols, and Volcanogenous Regosols. The neK content and exK content showed no significant correlation, indicating that they are independent with each other. Therefore, judging from the fact that neK and exK represent ‘moderately to slowly’ and ‘readily’ available K in soil, respectively, it is important to estimate both neK and exK, to evaluate K availability more comprehensively. This information can be useful for risk management of K deficiency in agricultural soils. It should be also noted, however, that not all of the neK content can be absorbed by plants, because neK is released gradually over the long term in accordance with the availability, as demonstrated by Moritsuka et al. (Citation2002), and the release of neK is dependent on many factors such as solution K concentration, pH, and other cation concentrations (e.g., Sparks Citation1987; Wang et al. Citation2011). In this context, further study is needed on the kinetics of neK release in soils for more precise evaluation of soil K availability.
Acknowledgments
The authors wish to thank Ms. Ayane Fujii, Dr. Naoki Moritsuka, Kyoto University, and Prof. Takashi Kosaki, Aichi University, for their collaboration in the analysis of soil exchangeable potassium, and Dr. Shuji Sano, Research Institute of Environment, Agriculture and Fisheries, Osaka Prefecture, for his collaboration in collecting soil samples and for valuable information on the soil samples investigated. This research was partly funded by the Japan Society for the Promotion of Science through a Grant-in-Aid for Scientific Research (B) (No. 16H04892).
Additional information
Funding
References
- Blakemore LC, Searle PL, Daly BK 1987: Extractable Iron, Aluminium and Silicon. Methods for Chemical Analysis of Soils, NZ Soil Bureau Scientific Report 80, Ed. Blakemore LC, Searle PL, Daly BK, pp. 71–76. NZ Soil Bureau Department of Scientific and Industrial Research, Lower Hutt, New Zealand.
- Bowen HJM 1979: Environmental Chemistry of the Elements, pp. 71–141. Academic press Inc., London, UK.
- Brouder S 2011: Potassium cycling. In Soil Management: Building a Stable Base for Agriculture, Eds. Hatfield JL, Sauer TL, American Society of Agronomy and Soil Science Society of America, Madison, WI, pp. 79–102.
- Cox AE, Joern BC, Brouder SM, Gao D 1999: Plant-available potassium assessment with a modified sodium tetraphenylboron method. Soil Sci. Soc. Am. J., 63, 902–911. doi:10.2136/sssaj1999.634902x
- Cultivated soil classification committee 1995: Classification of Cultivated Soils in Japan (3rd Approximation), p. 17. Miscellaneous publication of the National Institute of Agro-Environmental Sciences, Tsukuba, Japan.
- Darunsontaya T, Suddhiprakarn A, Kheoruenromne I, Gilkes RJ 2010: A comparison of extraction methods to assess potassium availability for Thai upland soils. 19th World Congress of Soil Science, Soil Solutions for a Changing World, 1 – 6 August 2010, Brisbane, Australia. Published on DVD.
- Eguchi T, Ohta T, Ishikawa T, Matsunami H, Takahashi Y, Kudo K, Yamaguchi N, Kihou N, Shinano T 2015: Influence of the nonexchangeable potassium of mica on radiocesium uptake by paddy rice. J. Environ. Radioactivity., 147, 33–42. doi:10.1016/j.jenvrad.2015.05.002
- Helmke PA, Sparks DL 2000: Potassium, Rubidium, and Cesium. Methods of Soil Analysis Part 3. Chemical Methods, pp. 559–562. SSSA Book Series. no. 5, Madison, WI.
- Kirkman JH, Basker A, Surapaneni A, MacGregor AN 1994: Potassium in the soils of New Zealand – A review. New Zealand J. Agric. Res., 37, 207–227. doi:10.1080/00288233.1994.9513059
- Kondo M, Maeda H, Goto A et al. 2015: Exchangeable Cs/K ratio in soil is an index to estimate accumulation of radioactive and stable Cs in rice plant. Soil Sci. Plant Nutr., 61, 133–143. doi:10.1080/00380768.2014.973347
- Liu L, Bates TE 1990: Evaluation of soil extractants for the prediction of plant available potassium in Ontario soils. Canadian J. Soil Sci., 70, 607–615. doi:10.4141/cjss90-063
- Marschner H 1995: Mineral Nutrition of Higher Plants, Academic Press, London, pp. 889.
- Martin HW, Sparks DL 1985: On the behavior of nonexchangeable potassium in soils. Commun. Soil Sci. Plant Anal., 16, 133–162. doi:10.1080/00103628509367593
- Meyer D, Jungk A 1993: A new approach to quantify the utilization of non-exchangeable soil potassium by plants. Plant Soil, 149, 235–243. doi:10.1007/BF00016614
- Mitsios IK, Rowell DL 1987: Plant uptake of exchangeable and non-exchangeable potassium. I. Measurement and modelling for onion roots in a chalky boulder clay soil. J. Soil Sci., 38, 53–63. doi:10.1111/j.1365-2389.1987.tb02122.x
- Moritsuka N, Yanai J, Fujii A, Sano S, Kosaki T 2003: Evaluation of readily available nonexchangeable potassium in soil by sequential extractions with 0.01 molar hydrochloric acid. Soil Sci. Plant Nutri., 49, 631–639.
- Moritsuka N, Yanai J, Kosaki T 2002: A sensitive method for detecting the depletion of nonexchangeable potassium in the rhizosphere using a sequential extraction with 0.01 molar hydrochloric acid. Soil Sci. Plant Nutr., 48, 577–583. doi:10.1080/00380768.2002.10409241
- Moritsuka N, Yanai J, Kosaki T 2004: Possible processes releasing nonexchangeable potassium from the rhizosphere of maize. Plant Soil, 258, 261–268. doi:10.1023/B:PLSO.0000016556.79278.7f
- Nakao A, Nakao A, Ogasawara S, Yanai J 2015: Aeolian-dust-derived micaceous minerals control radiocesium retention in Andosols in Japan. Soil Sci. Soc. Am. J., 79, 1590–1600. doi:10.2136/sssaj2015.05.0173
- Parfitt RL, Wilson AD 1985: Estimation of allophane and halloysite in three sequences of volcanic soils, New Zealand. Catena Suppl., 7, 1–8.
- Pratt PF 1965: Potassium. In Methods of Soil Analysis, Part 2, Chemical and Microbiological Properties, Agronomy Monograph 9.2, Ed. Black CA. pp. 1022–1030. American Society of Agronomy, SSSA.
- Sano S, Yanai J, Kosaki T 2004: Evaluation of soil nitrogen status in Japanese agricultural lands with reference to land use and soil types. Soil Sci. Plant Nutr., 50, 501–510. doi:10.1080/00380768.2004.10408506
- Schmitz GW, Pratt PF 1953: Exchangeable and nonexchangeable potassium as indexes to yield increases and potassium absorption by corn in the greenhouse. Soil Sci., 76, 345–354. doi:10.1097/00010694-195311000-00004
- SeaSolve Software Inc 2003: Peak Fit Ver4 Users Guide, p. 295. SeaSolve Software Inc., Framingham.
- Sharpley AN 1989: Relationship between soil potassium forms and mineralogy. Soil Sci. Soc. Am. J., 53 4, 1023–1028. doi:10.2136/sssaj1989.03615995005300040006x
- Silva JA, Bremner JA 1966: Determination and isotope-ratio analysis of different forms of nitrogen in soils: 5. Fixed Ammonium. Soil Sci. Soc. Am. J., 30, 587–594. doi:10.2136/sssaj1966.03615995003000050017x
- Sparks DL 1987: Potassium dynamics in soils. Adv. Soil . Sec., 6, 1–63.
- Soil Survey Staff 2014: Soil Taxonomy (12th Ed.), United States Department of Agriculture & Natural Resources Conservation Service, Washington, DC, pp. 360.
- The Geological Survey of Japan 1992: An illustrated guide to the rocks and minerals of Japan, Tokai University Press, Hatano. p. 151.
- Usherwood NR 1985: The role of potassium in crop quality. In Potassium in Agriculture, Ed. Munson RD, pp. 489–514. American Society of Agronomy, Crop Science Society of America, Soil Science Society of America, Madison, Wisconsin USA.
- Wang HY, Shen HQ, Zhou JM, Wang J, Du CW, Chen XQ 2011: Plants use alternative strategies to utilize nonexchangeable potassium in minerals. Plant Soil, 343, 209–220. doi:10.1007/s11104-011-0726-x
- Wood LK, DeTurk EE 1941: The absorption of potassium in soils in non-replaceable forms. Soil Sci. Am. J., 5, 152–161. doi:10.2136/sssaj1941.036159950005000C0026x
- Wood RA, Schroeder BL 1992: Release of nonexchangeable potassium reserves from a range of sugar industry soils. Potash Rev., 2, 1–14.
- Yamada H, Kamada A, Usuki, Yanai J 2009: Total selenium content of agricultural soils in Japan. Soil Sci. Plant Nutr., 55, 616–622. doi:10.1111/j.1747-0765.2009.00397.x
- Yamada H, Tanaka Y, Aoki S, Ozaki M, Shimotoyodome M, Yanai J 2013: Iodine and bromine concentrations in agricultural soils of Japan and their controlling factors. Jpn. J. Soil Sci. Plant Nutr., 84, 80–84. (in Japanese with English summary).
- Yamada I, Shoji S 1982: Retention of potassium by volcanic glasses of the topsoils of Andosols in Tohoku, Japan. Soil Sci., 133, 208–212. doi:10.1097/00010694-198204000-00002
- Yanai J, Aoki S, Ozaki M, Tanaka Y, Shimotoyodome M, Yamada H 2013: Iodine and bromine concentrations in agricultural soils of Japan in relation to soil type and region. Jpn. J. Soil Sci. Plant Nutr., 84, 85–89. (in Japanese with English summary).
- Yanai J, Mizuhara S, Yamada H 2015: Soluble selenium content of agricultural soils in Japan and its determining factors with reference to soil type, land use and region. Soil Sci. Plant Nutr., 61, 312–318. doi:10.1080/00380768.2014.997147
- Yanai J, Okada T, Yamada H 2012: Elemental composition of agricultural soils in Japan in relation to soil type, land use and region. Soil Sci. Plant Nutr., 58, 1–10. doi:10.1080/00380768.2012.658349
- Yanai J, Taniguchi H, Nakao A 2016: Evaluation of available silicon content and its determining factors of agricultural soils in Japan. Soil Sci. Plant Nutr., 62, 511–518. doi:10.1080/00380768.2016.1232601