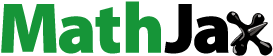
ABSTRACT
Saline-sodic soils commonly display a poor soil structure which is associated with the reduction in soil quality. Therefore, optimal management of soil and water salinity in saline-sodic soils should be regarded as an important factor in sustainable agriculture. The main purpose of the current study was to address the combined effect of irrigation and leaching managements on soil physical and chemical properties and drain water quality. Treatments including three irrigation water qualities, ECi = 0.6, 3 and 6 dS/m, two irrigation managements and three irrigation durations (8, 45 and 100 days). Two irrigation managements included intermittent irrigation at the depletion of 30% field capacity (M1) and daily irrigation with leaching fraction of 0.15 (M2) both followed by heavy leaching. Heavy leaching tests were applied on soil columns after 16, 56 and 114 days. The results showed that irrigation management and duration of the irrigation were found to be much more effective on infiltration, while irrigation water quality had a minor effect. The results revealed that final infiltration capacity under the daily irrigation was higher than the intermittent irrigation. Daily irrigation could control the salt until the medium water quality (ECi = 3 dS/m) and its soil chemical properties were similar to the intermittent irrigation. Daily irrigation had the most effect on EC control of soil and consequently on EC reduction of cumulative drain water compared to the intermittent irrigation. Reduced salinity by heavy leaching under the daily irrigation was more than intermittent irrigation. Under the intermittent irrigation, heavy leaching has increased the soil sodium adsorption ratio compared to the daily irrigation. Overall, under the daily irrigation, the soil physical and chemical properties were more suitable than the intermittent irrigation. According to the results, the intermittent irrigation is recommended in the areas where there are water resource limitations.
1. Introduction
Salinity and sodicity are two main concerns of irrigation water quality in dry and semi-arid regions. In arid and semi-arid areas, the irrigation water is limited for agriculture, and groundwater is the main source of irrigation (Jalali et al. Citation2008). In addition, increased pollution, especially in the drain water system network, threatens the application of these reuse strategies (Fleifle et al. Citation2012). Saline-sodic soils typically show a poor soil structure associated with the reduction in soil hydraulic conductivity, infiltration and aggregate stability (Shaygan et al. Citation2017; Cassel and Sharma Citation2018). Under water resources limitation conditions especially in arid and semi-arid regions, a proper irrigation management can increase agricultural production. Various practices can be used for the management of saline irrigation water. Some of these management practices are the use of freshwater at the end or during the growing period, frequent irrigation to reduce plant water stress, irrigation before cultivation to provide sufficient water storage for agricultural operations, salt leaching down to the root zone and use of the combination of saline water and freshwater. In general, it can be argued that the main reasons for soil salinities are saline irrigation water, high evaporation demand, insufficient leaching, inappropriate irrigation method and inefficient drainage condition (Rajabzadeh et al. Citation2009).
Leaching plays an essential role in the reclamation of saline-sodic soils (Oster et al. Citation1996). Leaching of salts in unsaturated soil is higher than in saturated soil (Gardner and Fireman Citation1958). van Schilfgaarde et al. (Citation1974) reported that in the absence of leaching, salt distribution is different and the accumulation of salts decreases as the depth increases. The effects of irrigation water salinity and Sodium Adsorption Ratio (SAR) on infiltration for a clay loam soil were examined in furrow irrigation. The results showed that the final intake rates decreased significantly with an increase in SAR and Electrical Conductivity (EC) during the irrigation period (Emdad et al. Citation2004). Hanson et al. (Citation1999) stated that the salt distribution follows the pattern of water flow in the soil. For example, the infiltrating water transfers salts from the upper surface to lower depths. They also concluded that soil type, the type of salt or chemical composition of the soil, the amount of water used, and the irrigation method would affect the distribution pattern and the movement of salts in the soil. Ragab et al. (Citation2008) observed that with increasing salinity of irrigation water, the plant-extracted available water in the soil decreased and the amounts of dissolved cations and anions in the soil (especially sodium) increased. Tagar et al. (Citation2010) compared continuous and alternate (intermittent) leaching methods in a clay loam soil. After two months of leaching, 46.1% of the salts were removed from 0 to 60 cm layer under intermittent leaching. The percent of salt leaching in the plots under continuous leaching was 59.6%. These researchers suggested that if time is a limiting factor, continuous leaching is preferable relative to intermittent leaching, otherwise, intermittent leaching is suggested. Ahmed et al. (Citation2012) indicated that irrigation with saline water (EC = 7 dS/m) caused the accumulation of salts in sandy soil as compared to freshwater (EC = 1.2 dS/m). Liu et al. (Citation2013) examined the effect of drip irrigation regime on water and salt distribution in the soil profile. The results showed that irrigation frequency and water quality had a significant effect on soil water content, salinity and root water uptake. Wang et al. (Citation2015) examined the distribution of salinity in irrigated soils with different levels of salinity and irrigation water volume. Irrigation water salinity levels were 3.3, 5 and 6.8 dS/m. The results showed that the effect of irrigation water salinity on soil salinity in the 0–40 cm layer was significant at 5% probability level, but no significant effect was observed in the layer of 40–100 cm.
Considering the limitation of water resources in arid and semi-arid regions (e.g., Iran) and use of low-quality irrigation waters, inappropriate management of irrigation in long-term periods can make severe damages to the soil quality. A number of studies conducted in different regions indicate that the use of saline and sodic water, without considering appropriate irrigation and leaching management can increase soil salinity and might lead to soil degradation (Mugai Citation2004; Prathapar et al. Citation2005; Shah et al. Citation2013; Shaygan et al. Citation2017). Nonetheless, little attention is paid to soil water content management and water quality in measuring and modeling the infiltration and leaching considering water requirement calculation. The recent investigations have studied the effect of irrigation water quality, the rate of leaching fraction, the initial soil water content and duration of the irrigation on leaching separately. Accordingly, the main objective of this study is to investigate the combined effect of these factors on soil physical and chemical properties and drain water quality in order to achieve a proper management under various conditions of soil and water quality.
2. Materials and methods
The combined effects of irrigation and leaching managements on soil physical and chemical properties were investigated by a laboratory experiment. Using a constant water head method, infiltration was measured in soil columns. After leaching, chemical properties of the soil profile were determined.
2.1. Treatments
Quantitative and qualitative limitations of water resources were considered as criteria for choosing the appropriate treatments. The experimental design was a factorial completely randomized design. As shown in , the treatments were three different irrigation water qualities, two irrigation managements and three irrigation durations (beginning, middle and end) with four replications (72 columns). One of the repetitions was used for sampling before the leaching test which is considered as the control treatment. The beginning (I1), middle (I2), and end of irrigation period (I3) were 8, 45 and 100 days, respectively. The sandy loam is the most common texture of the soil where surface irrigation is applied. Therefore, soil in this texture was used in this study (WRB: Haplic Calcisols (IUSS Working Group, WRB Citation2006)). Other physical and chemical properties of the studied soil are in . The soil hydraulic conductivity was measured by the constant head method (Klute and Dirksen Citation1986). Electrical conductivity of the saturated extract (ECe) was measured by an EC-meter (model WTW), and sodium adsorption ratio (SARe) was determined using a flame photometer (Jenway 500 731 Economical Flame Photometer) and calcium and magnesium concentrations were measured by EDTA titration method. Then, using the following equation, the SAR was calculated (Lesch and Suarez Citation2009):
Table 1. The experimental treatments
Table 2. Mean values of physical and chemical properties of the studied soil
where Na+, Ca2+ and Mg2+ are sodium, calcium and magnesium concentrations, respectively, which are expressed in Meq/L. Regarding the values of ECe and SARe, the soil was not saline-sodic, and also the values of soil hydraulic conductivity indicate that the soil had a moderate permeability. Generally, the soil is in good condition in terms of physical and chemical properties.
2.1.1. Water irrigation quality treatments
Three levels of water quality, including low, medium and high salinities and sodicities were used (). These three levels of irrigation water quality were chosen based on the studies of Emdad et al. (Citation2004) and Shainberg and Oster (Citation1978). Recent researches have shown that these three water quality have different effects on the physical and chemical properties of the soil. Therefore, these three water qualities were selected so that the interactive effect of irrigation management and water quality on the physical and chemical properties was investigated. Irrigation water salinities were made by adding the required amount of sodium chloride and calcium chloride to the distillation water.
Table 3. The experimental treatments of irrigation water quality
2.1.2. Irrigation management treatments
In this research, field capacity (FC) refers to the relatively constant soil water content reached after 48 h drainage of water from a saturated soil. At this water content, free drainage is negligible. Two following irrigation managements were used according to :
Table 4. Descriptions of two irrigation managements
Intermittent irrigation (M1): In this management, irrigation was done when the soil water content of the column reduced by 30% of field capacity (FC) (conventional management). Using the weighing method, soil water content was measured.
Daily irrigation (M2): In this management, the columns were irrigated daily with leaching fraction (LF) of 0.15 to prevent excessive accumulation of solutes in the soil columns (ideal management). The LF of 0.15 was chosen because the amount of leaching requirement (LR) rarely rises to 0.15 for irrigation water with a salinity of more than 3 dS/m and a yield reduction of less than 10% (Shainberg and Oster Citation1978).
Method of irrigating was flooding irrigation. In both irrigation managements, water depletion from the columns was due to surface evaporation and the soil water content after each irrigation event was reached to the FC. According to FC definition, under the intermittent irrigation, no drainage occurs after each irrigation, but under the daily irrigation, drainage occurs.
2.2. Soil columns preparation
Soil samples for this study were obtained from the 0–40 cm layer in Isfahan province, Iran, which is located at 51°04ʹ03.73” E longitude 32°59ʹ31.49” N latitude with an elevation of approximately 1919 m above mean sea level. Soil columns were prepared in PVC cylinders with the height of 60 cm and the diameter of 25 cm. The bottom of the columns was filled with 5 cm of gravel to provide drainage. The soil bulk density was 1.6 g/cm3 (natural value), and the top 15 cm of the column was kept empty to apply the irrigation water. The maximum uniformity of soil bulk density occurred in the condition that gravitational water content is 12% (Homaee Citation1999). Thus, to fill the columns, the applied soil was under this condition. The soil columns were filled to the depth of 40 cm with the density of 1.6 g/cm3. The columns were saturated from the bottom and to avoid evaporation, the soil columns were covered with plastics. After 48 h drainage of water from a saturated soil, the soil water content was equal to FC. Then, using the gravimetric method, soil water content was calculated at the FC ().
2.3. Laboratory conditions
This research was conducted at the Research Laboratory of the Department of Water Engineering, Isfahan University of Technology, Isfahan, Iran. To set the air temperature to 25 degrees Celsius, digital thermostats, fans, and air heaters were used.
2.4. Infiltration test through heavy leaching
In many cases, soil properties are assumed constant during the irrigation season, while these properties (especially soil physical properties) are changing throughout the season (Emdad et al. Citation2004). Accordingly, during irrigation season, assumption of the constant soil properties would reduce the performance of surface irrigation systems (Khatri and Smith Citation2006). Thus, infiltration test through heavy leaching with the same irrigation water was performed after the 16, 56 and 114 days for I1, I2 and I3, respectively, for the intermittent irrigation treatment and simultaneously for the daily irrigation treatment. The soil water content before the heavy leaching was equal to 0.70FC. According to by increasing the irrigation period, the irrigation interval was increased due to reduced soil water depletion. Therefore, the time difference between the last irrigation and heavy leaching increased. The water head above each soil column was set constant at 5 cm by using a Marriott-type bottle during the infiltration test. The duration of the test for I1, I2 and I3 were 100, 140 and 140 min, respectively. The final times for infiltration tests were set so that the infiltration rate reached a constant rate (f0). The drainage water was collected from the outlet of soil columns. Kostiakov–Lewis equation was applied to represent the cumulative infiltration as a function of elapsed time. The cumulative infiltration depth concerning time was used to determine the Kostiakov–Lewis coefficients using the Solver tool in MS Excel-2016 software. The equations were obtained with the minimum RMSE. The Kostiakov–Lewis equation and statistical criteria of the root mean squares of errors (RMSE) are used as follows (Ghorbani-Dashtaki et al. Citation2009):
where Z is the cumulative infiltration in cm, t is the intake opportunity time in min, the k and a coefficients are empirical, and fo is the final infiltration rate in cm/min, Ci is the calculated value, Oi is the measured value and n is the number of data.
2.5. Soil sampling
Sampling from soil columns was performed before and 48 h after the heavy leaching when the soil water content was at FC. Soil samples were obtained in three stages at the beginning (I1), middle (I2), and end of irrigation period (I3), from three soil depths of 0–12.5, 12.5–27.5 and 27.5–40.0 cm. The average value was calculated considering the thickness of the soil layers. Soil chemical properties including electrical conductivity of the saturated extract (ECe) and sodium adsorption ratio (SARe) were measured.
2.6. Statistical analysis
The experiment design was a factorial completely randomized design. Analysis of variance (ANOVA) was done to detect differences among the treatments using Statistical Analysis Software version 9.1 (SAS Institute, Cary, NC, USA). The ANOVA was performed for the data before the heavy leaching and after it. Least significant difference (LSD) test was done to separate the means of the effects that were found statistically significant at p ≤ 0.050. The combined effects of irrigation water quality, irrigation management and duration of the irrigation on the following properties were examined: soil physical properties, average soil salinity after leaching (ECa), average soil sodium adsorption ratio after leaching (SARa), ratio of irrigation water salinity (ECi) to cumulative drain water salinity (ECd) in each heavy leaching, ratio of SAR of irrigation water (SARi) to SAR of cumulative drain water (SARd) in each heavy leaching, the percentage of relative salinity (EC) changes due to heavy leaching (ECr) and the percentage of relative SAR changes due to heavy leaching (SARr). ECr and SARr are defined as follows:
where ECb and ECa are average soil salinity values before and after the heavy leaching, respectively, and SARb and SARa are average SAR values of the soil before and after the heavy leaching, respectively.
3. Results and discussion
3.1. The effect of irrigation treatments on control column
shows the chemical properties of control columns before the heavy leaching. Before leaching, ECb and SARb values, in general, remained higher in the surface layer (0–12.5 cm) than the subsurface (12.5–40 cm) layer in accord with the results of Chaudhari et al. (Citation2010). The capillary rise and surface evaporation of the soil caused the transfer of salts from the depths to the soil surface. Comparison of two irrigation managements showed that daily irrigation with LF of 0.15 could control the soil EC and SAR. Only at the end of irrigation period, leaching with high EC-SAR irrigation water failed to control well the soil SAR. This was due to the fact that LFs were not great enough to prevent salt accumulation in line with Bauder and Brock (Citation2001). This caused the accumulation of Na+ in the soil at the end of the period. Therefore, it caused a discrepancy in the soil chemical properties under the two irrigation managements. Therefore, the results showed that leaching could control Ca2+ and Mg2+ more than Na+ in the soil. The reason for this process might be the equilibrium between the solution and exchange phase of the soil. Because Na+ concentration was high in the irrigation water, it might be exchanged between the soil solution and the exchange phase and might release the Ca2+ and Mg2+ cations into the solution, thereby ultimately increased the soil SAR (Bohn et al. Citation2002). Similar to the results of other researchers (e.g., Gharaibeh et al. Citation2009), our results showed that leaching without the use of amendment materials could lead to soil sodification.
Table 5. Chemical properties of the control treatments before the heavy leaching
3.2. Soil and cumulative drain water properties as affected by the treatments
The analysis of variance (ANOVA) related to the effect of irrigation water quality, irrigation management and duration of the irrigation on the soil physical and chemical properties and cumulative drain water is presented in . The main effect of irrigation water quality on the final infiltration capacity was not significant. The main effect of irrigation management on the averages of soil salinity after leaching (ECa) and soil sodium adsorption ratio after leaching (SARa) was not significant. The main effect of duration of the irrigation on all the studied properties was significant. Except for ECr and SARr, the interactive effects of water quality and irrigation management on the studied properties were not significant. Except for final infiltration capacity, the interactive effects of irrigation water quality and duration of the irrigation on other properties were significant. Except for ECa and ECr, the interactive effects of irrigation management and duration of the irrigation on the other studied properties were significant. Except for SARa and SARr, the interactive effects of irrigation water quality, irrigation management and duration of the irrigation on the other studied properties were not significant.
Table 6. Analysis of variance of soil and cumulative drain water properties as affected by the treatments
3.2.1. Soil physical properties
Soils always undergo drying and wetting periods after irrigation and this condition can influence soil physical and chemical properties (Yan et al. Citation2017). According to , soil sealing was observed only in the intermittent irrigation that could significantly reduce infiltration rate (f0); this result was in agreement with the findings of Dinka and Lascano (Citation2012). Therefore, cumulative infiltrations (i.e., leaching volume) under the daily irrigation were higher than under the intermittent irrigation at the middle and end of irrigation periods (). The effect of irrigation water quality on final infiltration capacity was not significant in two managements. The destructive effects of SAR on soil structure would depend on the soil salinity, so that a soil with high salinity would be less affected by sodicity which means low sodium hazard (Ayers and Westcot Citation1994; Bauder and Brock Citation2001). The interactive effect of irrigation management and duration of the irrigation on the final infiltration capacity is presented in . Final infiltration capacity under the daily irrigation was higher than under the intermittent irrigation. Soil water management became effective during the wetting and drying periods. When the soil was dried and wetted successively, it caused the surface crusting (Rycroft et al. Citation2002). In order to solve this problem, researchers have proposed ways of breaking the surface crust by mechanical methods, maintaining high soil water content by repeated irrigation or increasing soil organic matter levels (Chu et al. Citation1991; Goyal Citation1982; Miller and Gifford Citation1974). Therefore, maintaining soil water in the daily irrigation would prevent surface sealing. Various studies have shown that wetting and drying periods are effective on soil aggregate stability, encapsulated air in soil, adhesion, and aggregate size distribution (Hussein and Adey Citation1998; Watts et al. Citation2001; Zhang et al. Citation2005). Probably, wetting and drying periods of the soil in daily irrigation reduced aggregate stability, and inducing crack formation in the soil surface. These cracks are increased with decreasing water and soil quality. Therefore, these cracks increased the cumulative infiltration and final infiltration capacity (f0). Wetting and drying periods of the soil for intermittent irrigation probably led to encapsulating air in the soil. The dispersion of soil particles and encapsulated air in the soil, in turn, reduced the infiltration rate (Emdad et al. Citation2004; Suarez et al. Citation2006; Zhou et al. Citation2008; Shaygan et al. Citation2017).
Table 7. Kostiakov–Lewis coefficients and cumulative infiltration for the studied treatments
Figure 1. Pictures of the surface of soil columns in the beginning of the period and before applying irrigation treatments (a), the treatment of intermittent irrigation after 10 irrigations with water of poor quality (b) and the treatment of daily irrigation after 10 irrigations with water of poor quality (c)
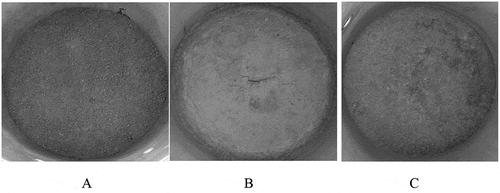
3.2.2. Average soil salinity after heavy leaching (ECa)
According to , salinity distribution (ECa) became uniform after the heavy leaching. Because the amount of salinity at the surface was greater than the subsoil, the heavy leaching transferred more salt from the surface, which were accumulated in the subsoil in accord with Hanson et al. (Citation1999). shows that the amount of salinity after heavy leaching was the same in both irrigation managements. The interactive effect of irrigation water quality and duration of the irrigation on soil salinity after leaching (ECa) is presented in . The highest salinity was related to poor water quality treatment for which the soil was irrigated 100 days (Q3.I3, ECa = 4.03 dS/m). At the beginning of irrigation period (I1), the higher irrigation water salinity increased the soil salinity. Increasing the duration of the irrigation and approaching the end of irrigation period caused the ECa to be increased. At the end of the period (I3), due to the high accumulation of salt, salinity was much higher than at the beginning of the period. These results are similar to the findings of Ragab et al. (Citation2008). In general, soil solution quality before the heavy leaching (ECb) and salinity of irrigation water had greater effects on salinity after leaching. This finding is in agreement with Tagar et al. (Citation2010), Corwin et al. (Citation2007) and Tedeschi and Dell’Aquila (Citation2005).
Table 8. Soil chemical properties of the treatments after the heavy leaching
3.2.3. Average soil sodium adsorption ratio after leaching (SARa)
According to , SAR values remained higher in the surface layer (0–12.5 cm) than the subsurface layer (12.5–40 cm). In order to achieve the equilibrium between the solution and exchange phases, the sodium decreased in exchange phase at surface soil and accumulated in the subsoil, causing an increase in the SAR at subsoil than before the heavy leaching. The interactive effect of irrigation water quality, irrigation management and duration of the irrigation on the soil SAR after leaching (SARa) is shown in . The highest and lowest values of SARa were determined for the daily irrigation after 100 days irrigation with poor quality (Q3.M2.I3, SARa = 14.56) and intermittent irrigation after 8 days irrigation with good quality (Q1.M1.I1, SARa = 0.87) treatments, respectively. At the end of the period, due to the high accumulation of Na+, SARa was much higher than at the beginning of the period. These results are similar to the findings of Ganjegunte et al. (Citation2018). Daily irrigation with LF of 15% showed that the greatest difference was observed between the two managements at the end of the period and under irrigation with poor water quality. As a result, until the middle of the irrigation period, no significant difference was observed in SARa values between the irrigation managements. At the end of the period, the soil SAR difference before the heavy leaching intensified the difference between the two management after the leaching.
3.2.4. Ratio of irrigation water salinity (ECi) to cumulative drain water salinity (ECd)
The interactive effect of irrigation water quality and duration of the irrigation on the ratio of ECi/ECd is presented in . The highest and lowest values of ECi/ECd were observed after the 8 days irrigation with poor quality and after 100 days irrigation with good quality, respectively. The ECi/ECd was smaller than 1, indicating that the cumulative drain water (leachate) salinity is greater than the salinity of irrigation water. According to and , the salt accumulation throughout the soil profile increased as it approached the end of the irrigation period. These results are in the line with Ghane et al. (Citation2009). At this time, leaching caused the removal of a high amount of salts from the soil column. The leaching of salts reduced the ratio of ECi/ECd. In addition, with the better quality of irrigation water, this ratio was reduced.
Table 9. Chemical properties of the cumulative drain water in the studied treatments
Figure 5. Means comparisons of ratio of irrigation water salinity to cumulative drain water salinity (ECi/ECd) as affected by the interaction of irrigation water quality and duration of the irrigation (LSD0.05)
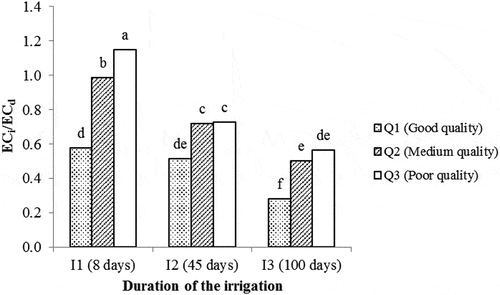
The interactive effect of irrigation management and duration of the irrigation on the ratio ECi/ECd is presented in . The highest and lowest ECi/ECd ratios were belonged to the intermittent irrigation after 8 days irrigation and the intermittent irrigation after 100 days irrigation, respectively. At the beginning of irrigation period, both irrigation managements did not differ significantly. Over the time and approaching to the end of irrigation period, a significant difference between the irrigation management was observed. This difference might be attributed to the leaching fraction of 0.15 in the daily irrigation, which was able to control the accumulation of salts in the soil. Under heavy leaching, removal of salt in the daily irrigation was lower than leaching of salt in the intermittent irrigation.
3.2.5. Ratio of SAR of irrigation water (SARi) to SAR of cumulative drain water (SARd)
The interactive effect of irrigation water quality and duration of the irrigation on SARi/SARd is presented in . The highest and lowest values of SARi/SARd were observed after 8 days irrigation with poor quality water and after 100 days irrigation with good quality water treatments, respectively. As previously explained, the salt accumulation throughout the soil profile was increased as it approached the end of the irrigation period. At the end of the irrigation period, leaching caused the removal of high amount of salts from the soil column. The leaching of salts reduced the ratio of SARi/SARd. The results showed that the SARd was lower than SARi. These findings showed that soluble Mg2+ and Ca2+ are removed from the soil more than Na+ due to leaching.
Figure 7. Means comparisons of ratio of SAR of irrigation water to SAR of cumulative drain water (SARi/SARd) as affected by the interaction of irrigation water quality and duration of the irrigation (LSD0.05)
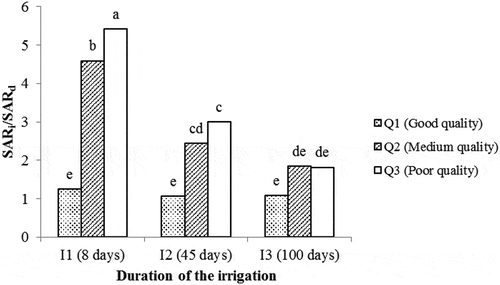
The interactive effect of irrigation management and duration of the irrigation on the ratio SARi/SARd is presented in . The highest and lowest values of SARi/SARd were observed after the 8 and 100 days irrigation in the daily irrigation, respectively. This ratio at the beginning of irrigation period did not differ significantly in both irrigation managements. Approaching to the mid of the irrigation period, a significant difference between the irrigation managements was observed. The reason for this difference was the leaching fraction in the daily irrigation, which was able to control the Ca2+ and Mg2+ of soil. In the intermittent irrigation, there was no leaching fraction. As a result, by performing heavy leaching, the sodium cation in the soil solution was moved to the exchange phase, and as a consequence, the calcium and magnesium moved back to the solution phase. This process reduced SAR in the cumulative drain water (SARi/SARd>1). Daily irrigation caused the soil sodification, so heavy leaching was able to transfer the sodium cation to solution phase (see ). This process increased the SAR in cumulative drain water as compared to the intermittent irrigation; thus, the ratio SARi/SARd in the daily irrigation was lower than that in the intermittent irrigation.
3.2.6. The percentage of relative salinity changes due to heavy leaching (ECr)
shows the interactive effect of irrigation water quality and duration of the irrigation on the percentage of relative salinity changes (ECr). The value of ECr close to zero indicates that leaching has no effect on the soil salinity. When the ECr value is greater than zero, it shows that heavy leaching has increased the soil salinity. The highest and lowest values belonged to the poor water quality after 8 days irrigation and the medium water quality after 100 days irrigation, respectively. In the beginning of the irrigation period, leaching has increased soil salinity due to low soil salinity. Similar to the results of daily irrigation, heavy leaching has been able to reduce salinity at the end of the period.
Figure 9. Means comparisons of the percentage of relative salinity changes (ECr) as affected by the interaction of irrigation water quality and duration of the irrigation (LSD0.05)
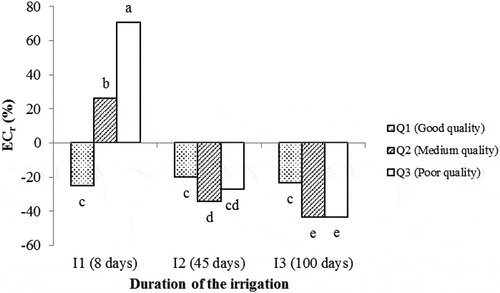
shows the interactive effect of irrigation water quality and irrigation management on the percentage of relative salinity changes (ECr). Under both irrigation managements, the salinity reduction was considerable in good and medium water quality treatments. The salinity reduction due to heavy leaching in the daily irrigation was higher than in the intermittent irrigation. As previously mentioned, cumulative infiltrations (i.e., leaching volumes) under the daily irrigation were higher than under the intermittent irrigation. Therefore, it can transfer more salt from the soil.
3.2.7. The percentage of relative SAR changes due to heavy leaching (SARr)
shows the interactive effects of irrigation water quality, irrigation management and duration of the irrigation on the percentage of relative SAR changes (SARr). The highest and lowest ratios were obtained for the intermittent irrigation after 100 days irrigation with good quality water and the daily irrigation after 8 days irrigation with medium quality water treatments, respectively. From the beginning of the irrigation period, the SARr was different between the treatments due to changes in the water quality and irrigation management. Due to the high amount of sodium in the leaching water, the SAR increased after heavy leaching. This increase was higher for the intermittent irrigation. Removal of Ca2+ and Mg2+ was high in the intermittent irrigation. As a result, leaching in the intermittent irrigation led to an increase in the soil SAR. With increasing sodium in the irrigation water and number of irrigation, heavy leaching had no effect on the SARa (SARr = 0). For good quality of irrigation water, with increasing the duration of the irrigation, the effect of leaching on SARr was greater and increased the SARa. As mentioned earlier, the relative changes in the soil solution quality due to leaching in a non-salt soil were higher.
4. Conclusions
In this research, the effects of irrigation and leaching management on selected soil physical and chemical properties and drain water quality were investigated. Two irrigation managements included intermittent irrigation at the discharge of 30% field capacity (M1) and daily irrigation with leaching fraction of 0.15 (M2). The results also showed that the effect of initial soil water content on infiltration coefficients under the daily irrigation was greater as compared to the intermittent irrigation. The number of wetting and drying periods that caused soil crust formation, depended on the irrigation management and duration of the irrigation. Final infiltration capacity under the daily irrigation was higher than the intermittent irrigation as the daily irrigation did not induce the formation of soil crust but the intermittent irrigation did. In order to further examine the other effects of wetting and drying periods, it is recommended to measure the aggregate stability and monitor the soil structure changes during the irrigation period using non-destructive methods such as computed tomography.
In general, until the middle of irrigation period, all soil chemical properties and cumulative drain water quality were similar in both irrigation managements. The greatest difference was observed between the two managements at the end of the period and under irrigation with poor water quality. At the end of the period, leaching fraction of 0.15 under the daily irrigation using high EC-SAR irrigation water failed to control the EC and SAR of soil. This caused the accumulation of salts at the end of the period. Thus, leaching fraction of the present study was not great enough to prevent salt accumulation, and heavier leaching should be performed under the daily irrigation. Daily irrigation had the most effect on EC control of soil, though it had a minor effect on SAR control of soil. Therefore, cumulative drain water salinity under the daily irrigation was less than the intermittent irrigation, whereas the SAR has an inverse behavior. Reduced salinity by heavy leaching under the daily irrigation was more than the intermittent irrigation. Under the intermittent irrigation, heavy leaching increased the soil SAR; however, in the daily irrigation the soil SAR was not significantly altered. Daily irrigation failed to control the SAR of soil. Therefore, the soil SAR was closer to the SAR of irrigation water. The effect of heavy leaching on the soil chemical properties under the daily irrigation was significant. Overall, under the daily irrigation, the soil physical and chemical properties were more suitable compared to the intermittent irrigation. According to the results, the daily irrigation is recommended in areas where there is no limitation of water resources. It is worth to mention that these recommendations are only for areas with medium-textured soils associated with natural or artificial drainage systems.
References
- Ahmed CB, Magdich S, Rouina BB, Boukhris M, Abdullah FB 2012: Saline water irrigation effects on soil salinity distribution and some physiological responses of field grown Chemlali olive. Environ. Manage., 113, 538–544. doi:10.1016/j.jenvman.2012.03.016
- Ayers RS, Westcot DW 1994: Food, agriculture organization of the United Nations (FAO), water quality for agriculture. Irrigation and Drainage, Rome, Paper, (29).
- Bauder JW, Brock TA 2001: Irrigation water quality, soil amendment, and crop effects on sodium leaching. Arid Land Res. Manage., 15(2), 101–113. doi:10.1080/15324980151062724
- Bohn HL, Myer RA, O’Connor GA 2002: Soil Chemistry. John Wiley & Sons.
- Cassel F, Sharma S 2018: Delocalization of salt solution in a semiarid farmland topsoil. Water Air Soil Pollut., 229(8), 254. doi:10.1007/s11270-018-3905-z
- Chaudhari SK, Singh R, Kumar A 2010: Suitability of a hydraulic‐conductivity model for predicting salt effects on swelling soils. J. Plant Nutr. Soil Sci., 173(3), 360–367. doi:10.1002/jpln.200800075
- Chu YN, Coble CG, Jordan WR 1991: Cotton emergence force as affected by soil temperature, moisture, and compression. Crop. Sci., 31(2), 405–409. doi:10.2135/cropsci1991.0011183X003100020035x
- Corwin DL, Rhoades JD, Šimůnek J 2007: Leaching requirement for soil salinity control: steady-state versus transient models. Agric. Water. Manage., 90(3), 165–180. doi:10.1016/j.agwat.2007.02.007
- Dinka TM, Lascano RJ 2012: Challenges and limitations in studying the shrink-swell and crack dynamics of vertisol soils. Open J. Soil Sci., 2(2), 82. doi:10.4236/ojss.2012.22012
- Emdad MR, Raine SR, Smith RJ, Fardad H 2004: Effect of water quality on soil structure and infiltration under furrow irrigation. Irrig.Sci., 23(2), 55–60. doi:10.1007/s00271-004-0093-y
- Fleifle AE, Saavedra Valeriano OC, Nagy HM, Elfetiany FA, Tawfik A, Elzeir M 2012: Simulation-optimization model for intermediate reuse of agriculture drainage water in Egypt. J. Environ. Eng., 139(3), 391–401. doi:10.1061/(ASCE)EE.1943-7870.0000605
- Ganjegunte G, Ulery A, Niu G, Wu Y 2018: Treated urban wastewater irrigation effects on bioenergy sorghum biomass, quality, and soil salinity in an arid environment. Land Degrad. Dev., 29(3), 534–542. doi:10.1002/ldr.2883
- Gardner WR, Fireman M 1958: Laboratory studies of evaporation from soil columns in the presence of a water table. Soil Sci., 85(5), 244–249. doi:10.1097/00010694-195805000-00002
- Ghane E, Feizi M, Mostafazadeh-Fard B, Landi E 2009: Water productivity of winter wheat in different irrigation/planting methods using saline irrigation water. Int. J. Agric. Biol.. 11(2), 131–137.
- Gharaibeh MA, Eltaif NI, Shunnar OF 2009: Leaching and reclamation of calcareous saline-sodic soil by moderately saline and moderate‐SAR water using gypsum and calcium chloride. J. Plant Nutr. Soil Sci., 172(5), 713–719. doi:10.1002/jpln.200700327
- Ghorbani-Dashtaki S, Homaee M, Mahdian MH, Kouchakzadeh M 2009: Site-dependence performance of infiltration models. Water. Resour. Manage., 23(13), 2777–2790. doi:10.1007/s11269-009-9408-3
- Goyal MR 1982: Soil crusts vs seedling emergence. No. REP-12308. CIMMYT.
- Hanson B, Grattan SR, Fulton A 1999: Agricultural salinity and drainage. University of California irrigation program. University of California, Davis.
- Homaee M1999: Root water uptake under non-uniform transient salinity and water stress. PhD Thesis, Wageningen Agriculture University, The Netherlands.
- Hussein J, Adey MA 1998: Changes in microstructure, voids and b-fabric of surface samples of a vertisol caused by wet/dry cycles. Geoderma., 85(1), 63–82. doi:10.1016/S0016-7061(98)00014-7
- IUSS Working Group, WRB 2006: World reference base for soil resources. World Soil Resources Report, 103.
- Jalali M, Merikhpour H, Kaledhonkar MJ, Van Der Zee SEATM 2008: Effects of wastewater irrigation on soil sodicity and nutrient leaching in calcareous soils. Agric. Water. Manage., 95(2), 143–153. doi:10.1016/j.agwat.2007.09.010
- Khatri KL, Smith RJ 2006: Real-time prediction of soil infiltration characteristics for the management of furrow irrigation. Irrig. Sci., 25(1), 33–43. doi:10.1007/s00271-006-0032-1
- Klute A, Dirksen C 1986: Hydraulic conductivity and diffusivity: laboratory methods. Methods of soil analysis: part 1—physical and mineralogical methods, (methodsofsoilan1), 687–734.
- Lesch SM, Suarez DL 2009: A short note on calculating the adjusted SAR index. Trans. ASABE., 52(2), 493–496. doi:10.13031/2013.26842
- Liu M, Yang J, Li X, Liu G, Yu M, Wang J 2013: Distribution and dynamics of soil water and salt under different drip irrigation regimes in northwest China. Irrig. Sci., 31(4), 675–688. doi:10.1007/s00271-012-0343-3
- Miller DE, Gifford RO 1974: Modification of soil crusts for plant growth. Tech. Bull. Agric. Exp. Stn. Univ. Ariz., 214, 7–16.
- Mugai EN 2004: Salinity characterization of the Kenyan saline soils. Soil Sci. Plant Nutr., 50(2), 181–188. doi:10.1080/00380768.2004.10408467
- Oster JD, Shainberg I, Abrol IP 1996: Reclamation of salt-affected soil. Soil Erosion, Conserv. Rehabil., 315–352.
- Prathapar SA, Aslam M, Kahlown MA, Iqbal Z, Qureshi AS 2005: Gypsum slotting to ameliorate sodic soils of Pakistan. Irrig. Drain., 54(5), 509–517. doi:10.1002/ird.205
- Ragab AAM, Hellal FA, El-Hady MA 2008: Water salinity impacts on some soil properties and nutrients uptake by wheat plants in sandy and calcareous soil. Ajbas., 2(2), 225–233. doi:10.1002/ird.205
- Rajabzadeh F, Pazira E, Mahdian MH, Mahmoudi S, Heidarizadeh M 2009: Leaching saline and sodic soils along with reclamation-rotation program in the mid-part of Khuzestan, Iran. J. Appl. Sci., 22, 4020–4025. doi:10.3923/jas.2009.4020.4025
- Rycroft DW, Kyei‐Baffour N, Tanton T 2002: The effect of sodicity on the strength of a soil surface. Irrig. Drain., 51(4), 339–346. doi:10.1002/ird.64
- Shah Z, Haq IU, Rehman A, Khan A, Afzal M 2013: Soil amendments and seed priming influence nutrients uptake, soil properties, yield and yield components of wheat (Triticum aestivum L.) in alkali soils. Soil Sci. Plant Nutr., 59(2), 262–270. doi:10.1080/00380768.2012.762634
- Shainberg I, Oster JD 1978: Quality of Irrigation Water.
- Shaygan M, Reading LP, Baumgartl T 2017: Effect of physical amendments on salt leaching characteristics for reclamation. Geoderma., 292, 96–110. doi:10.1016/j.geoderma.2017.01.007
- Suarez DL, Wood JD, Lesch SM 2006: Effect of SAR on water infiltration under a sequential rain–irrigation management system. Agric. Water. Manage., 86(1–2), 150–164. doi:10.1016/j.agwat.2006.07.010
- Tagar AA, Siyal AA, Brohi AD, Mehmood F 2010: Comparison of continuous and intermittent leaching methods for the reclamation of a saline soil. Pak. J. Agri., Eng., Vet. Sci., 26, 36–47.
- Tedeschi A, Dell’Aquila R 2005: Effects of irrigation with saline waters, at different concentrations, on soil physical and chemical characteristics. Agric. Water. Manage., 77(1–3), 308–322. doi:10.1016/j.agwat.2004.09.036
- van Schilfgaarde J, Bernstein L, Rhoades JD, Rawlins SL 1974: Irrigation management for salt control. J. Irrig. Drain. Div.. 100(3), 321–338.
- Wang X, Yang J, Liu G, Yao R, Yu S 2015: Impact of irrigation volume and water salinity on winter wheat productivity and soil salinity distribution. Agric. Water. Manage., 149, 44–54. doi:10.1016/j.agwat.2014.10.027
- Watts CW, Whalley WR, Longstaff DJ, White RP, Brook PC, Whitmore AP 2001: Aggregation of a soil with different cropping histories following the addition of organic materials. Soil Use Manage., 17(4), 263–268. doi:10.1111/j.1475-2743.2001.tb00036.x
- Yan M, Junzeng X, Qi W, Shihong Y, Linxian L, Suyan C, Qi L 2017: Organic carbon content and its liable components in paddy soil under water-saving irrigation. Plant Soil Environ., 63(3), 125–130. doi:10.17221/817/2016-PSE
- Zhang B, Horn R, Hallett PD 2005: Mechanical resilience of degraded soil amended with organic matter. Soil Sci. Soc. Am. J., 69(3), 864–871. doi:10.2136/sssaj2003.0256
- Zhou X, Lin HS, White EA 2008: Surface soil hydraulic properties in four soil series under different land uses and their temporal changes. Catena., 73(2), 180–188. doi:10.1016/j.catena.2007.09.009