ABSTRACT
Denitrification in paddy soils is an environmentally important reaction, which reduces leaching or emission of nitrogen pollutants. This reaction has long been considered to be driven by typical denitrifiers mostly belonging to Alpha-, Beta-, and Gammaproteobacteria. Our recent metatranscriptomic analysis of rice paddy field soil suggested that paddy soil denitrification is a cooperative process of different nitrogen oxide reducers, i.e., NO2−, NO, and N2O reducers. However, it was not clear whether denitrification actually occurred in the soil samples examined, which was not easy to confirm under field conditions. Therefore, we carried out this study (1) to establish a soil microcosm with the steady activity of denitrification, and (2) to identify microbial communities, which drive each step of denitrification in the soil microcosm by metatranscriptomic analysis. As a result, a chemostat-type soil microcosm with steady denitrification activity was successfully established. Metatranscriptomic analysis of the denitrification-induced soils, together with supportive PCR-based clone library analysis, indicated that the community compositions of microbial drivers at each step of denitrification are different from each other. The results verified our above-mentioned suggestion of denitrification in paddy soil being a cooperative process of different nitrogen oxide reducers. Microbial communities of the cooperative denitrification in paddy soil might be established, and might have advantages to eliminate/detoxify microbiologically and/or chemically generated nitrogen oxides smoothly under changing paddy soil environments.
1. Introduction
In anoxic zones of waterlogged paddy soils, various kinds of reductive reactions (i.e., denitrification, metal reduction, sulfate reduction, and methane generation) proceed, mostly driven by microorganisms. Among these reactions, denitrification is an environmentally important reaction, which reduces leaching or emission of nitrogen pollutants (NO3−, NO2−, and N2O) below ground or into the atmosphere (Ishii et al. Citation2011; Xiong et al. Citation2010).
Enzymes involved in denitrification reactions are nitrate reductase (Nar/Nap), nitrite reductase (Nir), nitric oxide reductase (Nor), and nitrous oxide reductase (Nos) (Hallin et al. Citation2018). Genes encoding these denitrification enzymes, nir, nor and nos have been used as marker genes to examine the diversity and community composition of denitrifying microorganisms in the environment. In the PCR-based analyses of soil DNA and RNA from paddy and other agricultural soils, nir, nor and nos genes derived from bacteria belonging to Alpha-, Beta-, and Gammaproteobacteria have been detected frequently (Huang et al. Citation2011; Yoshida et al. Citation2009; Smith et al. Citation2007; Francis et al. Citation2013). Most of the denitrifiers belonging to these phyla can drive more than two successive steps of denitrification, including the reduction of nitrite to nitric oxide, which is a key step defining denitrification (Zumft et al., Citation1997).
Recently, several studies have revealed the presence of previously undetected denitrification genes in the environment. For example, Jones et al. (Citation2013) reported that previously undetected nosZ-harboring, phylogenetically diverse bacteria were present and widespread in various environments. We also found, in the PCR-based analysis of denitrifiers in the environments, that conventional nirK/nirS primers overlooked large varieties of denitrifiers. This is because conventional primers were designed to detect nirK/nirS of cultivable denitrifier strains belonging to Alpha-, Beta-, and Gammaproteobacteria (Wei et al. Citation2015). Clone library analysis using the newly designed primers revealed that nirK and nirS in terrestrial environments are more phylogenetically diverse than those revealed with the conventional primers (Wei et al. Citation2015).
Our recent metatranscriptomic analysis of paddy soil suggested that paddy soil denitrification might be a cooperative process by different nitrogen oxide reducers, i.e., NO2−, NO, and N2O reducers (Masuda et al. Citation2017). However, because denitrification activity (i.e., N2 production) in submerged paddy soil is not easy to detect under field conditions, we still do not know whether the identified nitrogen oxide reducers from the metatranscriptomic analysis are practically responsible for denitrification. Thus, we here established the paddy soil which exhibited the continuous denitrification activity and tried to verify the denitrification as a cooperative process. Consequently, we carried out this study (1) to construct a denitrification-induced soil microcosm with the steady activity of denitrification, and (2) to determine which microbial communities drive each step of denitrification in the denitrification-induced soil by metatranscriptomic and clone library analyses.
2. Materials and methods
The soil was collected from a rice paddy field at Niigata Agricultural Research Institute, Nagaoka, Niigata, Japan (37°44ʹN, 138°87ʹE) in June 2016. The soil samples were sieved with a 4-mm mesh and stored at 4°C. Physicochemical characteristics of the soil were as follows: soil taxonomy, eutric fluvisol; soil type, gley soil; soil texture, clay loam; soil pH, 5.6; total carbon, 12.4 (g•kg−1); total nitrogen, 0.85 (g•kg−1). For preincubation of the soil, 280 g of soil and 560 ml of distilled water (50% moisture content) were added to three 1000 ml screw bottles (Duran, Frankfurt, Germany) and incubated for 3 days at 26.5 °C to decrease soil redox potential and to consume nitrate possibly existing in the soil. Soil redox potential decreased from 190 mV to −101 mV during the preincubation, and nitrate concentration in the soil after the preincubation was under the detection limit (0.1 µM). After the preincubation, the soil was divided into six 500 ml screw bottles (Duran, Frankfurt, Germany). The caps of the screw bottles were equipped with four ports. Two ports were connected with micro-tubes (Saint Gobain, Paris, France) and used for inflow/outflow of solution (chemostat-type soil microcosm): A Na15NO3 solution (3 mM) was continuously injected into three bottles at 12 ml/h using a micro-tube pump (Rikakikai MP-3B, Tokyo, JAPAN), while distilled water was continuously injected into the other three bottles as a control. The other two ports were used for argon gas inflow/outflow: Argon gas was continuously injected into the floodwater part in the bottles through the micro-tube at 1 ml/h to maintain anaerobic soil conditions. Schematic figure of this microcosm is shown in Figure S1.
The flux of 15N2O and 15N2 in the microcosms was measured every day. Bottle caps were exchanged with other ones, ports of which were closed with silicone stoppers. Air phase in the bottles was replaced with argon gas using gas transfer unit SSC-9920 (Senshu Scientific Co., Japan). Just before and 2 h after argon gas replacement, gas samples were collected using a microsyringe through the silicone stopper. The collected gas samples were injected to GCMS-QP2010 Plus (Shimadzu, Kyoto, Japan), and the amount of each gas generated per hour per 1 g of dry soil was calculated.
Soil samples (soil suspensions) were collected on the 0th, 2nd, 4th, 6th, and 8th day of incubation, after thoroughly shaking the bottles by hand. Collected samples were immediately frozen in dry ice/ethanol baths and stored at −80°C until the determination of nitrate concentration and soil RNA analysis. RNA was extracted from the soil collected at day 6 of incubation and purified as described previously (Itoh et al. Citation2013). For metatranscriptomic analysis, purified RNA was reverse-transcribed using the NEB Next Ultra RNA Library Prep Kit for Illumina (New England Biolabs Inc., Beverly, Massachusetts, USA) according to the manufacturer’s instructions, and sequenced as described previously (Masuda et al. Citation2017). Obtained paired-end sequences were processed and analyzed using MG-RAST server ver. 4.0 (Meyer et al., Citation2008) and BLASTX as described previously (Masuda et al. Citation2017). The number of sequence reads obtained by the metatranscriptomics is shown in Table S1. For qPCR and clone library analysis, purified RNA was reverse-transcribed using random hexamers (Takara Bio, Otsu, Shiga, Japan) and SuperScript Reverse Transcriptase III (Invitrogen, Carlsbad, CA, USA). qPCR was performed to amplify nir, nor, nos and 16S rRNA genes using the StepOnePlus System (Life Technologies, Carlsbad, CA, USA) with KOD SYBR qPCR Mix (Toyobo, Osaka, Japan) for nir genes, and TB GreenTM Premix EX TaqTM (Takara Bio, Shiga, Japan) for nor, nos and 16S rRNA genes, respectively. PCR primer sets used in this study are listed in Table S5. Clone library analysis followed the protocols described previously (Yoshida et al. Citation2012). For determination of nitrate concentration in soil, soil samples were centrifuged (3500 x g, 5 min) and supernatant solutions were subjected to an ion chromatograph (Shimadzu ISC-900, Japan). Nitrate concentration in the outflow solution was determined through ion chromatography.
3. Results and discussion
The concentration of nitrate in outflow solution from the nitrate-added soil microcosm was maintained at ca. 1.5 mM after 2 days of incubation, suggesting that about half of the nitrate contained in the inflow solution (3.0 mM) was consumed (Figure S2). The gas flux of 15N2O and 15N2 increased after the initiation of incubation and became stable from day 4 to 6 and day 4 to 8, respectively (Figure S3). We thus successfully constructed the soil microcosm with steady denitrification activity (i.e., day 4 to 6), and used it for the subsequent metatranscriptomic analysis and clone library analysis.
In the soil microcosm, relatively high N2O flux was observed, although N2O flux is generally not detected or quite low in submerged paddy fields (Akiyama et al. Citation2005). This means that both N2O and N2 were generated in the soil microcosm used in this study. Continuous injection of Ar gas into the flood water part might release of dissolved N2O into the headspace. Actually, Tago et al. (Citation2011) reported that denitrifying bacteria isolated from paddy soils generated both N2O and N2 gas as the end products of denitrification.
To clarify the denitrifiers when denitrification was active, soil RNA at day 6 of incubation was subjected to metatranscriptomic analysis. According to the results, a large majority of nir gene transcripts were closely related to nirS or nirK of denitrifiers belonging to class Beta-, Alpha-, and Gammaproteobacteria (, Table S2), in agreement with previous studies on paddy soil denitrifiers (Yoshida et al. Citation2009; Chen et al. Citation2012; Masuda et al. Citation2017). In contrast, nor and nos transcripts showed relatively large sequence diversity. Transcripts of nor were closely related to that of denitrifiers belonging to class Beta-, Gamma-, and Deltaproteobacteria, phyla Acidobacteria, Planctomycetes, and Nitrospirae (, Table S3). Transcripts of nos were closely related to that of denitrifiers belonging to class Beta-, Gamma-, and Deltaproteobacteria, phyla Nitrospirae, Acidobacteria and Chloroflexi (, Table S4). The predominance of nor and nos transcripts derived from phylogenetically diverse bacteria is inconsistent with the results of our previous metatranscriptomic analysis of paddy field soil (Masuda et al. Citation2017). It should be noted that Deltaproteobacteria, Acidobacteria, and Planctomycetes, frequently detected as microbial drivers of nitric oxide reduction, were hardly detected in nitrite reduction (). This suggests that they are important reducers of nitric oxide, but not nitrite.
Figure 1. Taxonomic composition at the bacterial phylum and proteobacterial class level relevant to gene transcripts of nir, nor, and nos based on metatranscriptomic analysis
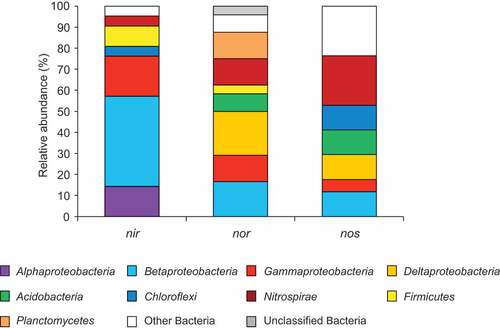
The results of our metatranscriptomic analysis of a denitrification-induced soil microcosm indicated that the community compositions of microbial drivers at each step of denitrification are different from each other, and also indicated that denitrification is cooperatively driven by these different denitrifier communities. These results verified our previous suggestion that paddy soil denitrification is a cooperative process of different nitrogen oxide reducers, i.e. NO2−, NO, and N2O reducers (Masuda et al. Citation2017).
We also performed the qPCR and clone library analysis of denitrification functional gene transcripts using soil RNA extracted from soil samples at day 6 of incubation. In qPCR using multiple primer sets (Table S5), nirS cluster I and qnorB transcripts were quantified, in which number of transcripts were significantly higher in 15NO3− added samples than those in control samples (p< 0.01) (). Transcripts of other genes could not be quantified, probably because primer sequence and/or qPCR conditions were not suitable to amplify the gene transcripts present in the soil. In the clone library of nirS cluster I transcripts, sequences were assigned to nirS of denitrifiers belonging to class Alpha-, Beta-, and Gammaproteobacteria (Table S6). In contrast, in the clone library of qnorB transcripts, there were diverse sequences closely related to qnorB of phyla Acidobacteria, Bacteroidetes, Cyanobacteria, and that of class Delta-, Gamma-, and Betaproteobacteria (Table S6). Results of the clone library analysis indicated that microbial communities driving nitrite reduction and nitric oxide reduction were largely different from each other, and that Deltaproteobacteria and Acidobacteria were major drivers of nitric oxide reduction (Table S6). This is in agreement with the results of the metatranscriptomic analysis described above.
Table 1. The amounts of 16S rRNA, nirS Cluster I, qnorB transcripts of cDNA samples from 15NO3-added microcosm and control. Different characters indicate significant statistical differences between the two groups
We here performed both metatranscriptomic and clone library analyses to cover the methodological limitation of the respective analyses. We unfortunately obtained only a small number of sequence reads for each gene (Table S1) in the metatranscriptomic analysis. Further, the length of those reads did not necessarily have sufficient lengths (30–60 aa), which might cause biases in identifying the taxonomy of gene owners. On the other hand, while clone library analysis is inevitably biased by the use of PCR primers (Wei et al. Citation2015), we obtained the larger number of reads with larger length (approximately 200 aa on average). The longer reads enable more accurate identification, which made the results of metatranscriptomic analysis more convincing.
In this study, denitrification was found to be a cooperative process of different nitrogen oxide reducers as verified by metatranscriptomic and cloning analysis of denitrification-induced paddy soil. Both analyses showed differences in the community composition of different nitrogen oxide reducers (, Table S2 – S4, S6). Nitric oxide is a highly reactive and harmful substance to microbes (Plate and Marletta Citation2012), which could be generated in paddy soil by both microbial (dissimilatory nitrate reduction to ammonium and nitrification) (Schreiber et al. Citation2012) and iron-mediated chemical reactions (Brons et al. Citation1991). To eliminate and detoxify nitric oxide, a diverse microbial community of nitric oxide reduction might be established in paddy soil. Nitrous oxide, on the other hand, is generated through both nitrification and denitrification in paddy soil (Lou et al. Citation2007). Actually, many strains of denitrifiers isolated from paddy soils generate nitrous oxide (Tago et al. Citation2011). To utilize nitrous oxide as an electron acceptor under anaerobic conditions, unique microbial communities of nitrous oxide reduction might be established in paddy soil. The cooperative denitrification verified in this study can be regarded as a cooperative microbial metabolic pathway distributed among a consortium of microorganisms, each of which contains the part of a metabolic pathway that it is best suited to hosting (Zhou et al. Citation2015). This cooperative metabolic pathway enables enhanced production of end-products of a metabolic pathway, where parts of pathways do not require specialized environments or compartments for optimal function (Zhou et al. Citation2015). Similar to this metabolic pathway in the microbial consortium, cooperative denitrification in paddy soil might have advantages to generate the end-product (dinitrogen gas) smoothly under a changing natural soil environment.
TableS6.docx
Download MS Word (160.6 KB)TableS5.docx
Download MS Word (96 KB)TableS4.docx
Download MS Word (140.9 KB)TableS3.docx
Download MS Word (174.2 KB)TableS2.docx
Download MS Word (155.8 KB)TableS1.docx
Download MS Word (117.5 KB)Figure_S3.pdf
Download PDF (43 KB)FigureS2.pdf
Download PDF (39.8 KB)FigureS1.pdf
Download PDF (39.5 KB)Acknowledgments
We thank Yutaka Shiratori of Niigata Agricultural Research Institute for providing soil samples. We also thank Shigeto Otsuka of the University of Tokyo for his valuable comments. This study was supported by JSPS KAKENHI Grant Numbers JP25252013, JP15K14675, and JP17H01464, Japan.
Supplementary material
Supplemental data for the article can be accessed here.
Additional information
Funding
References
- Akiyama H, Yagi K, Yan X 2005: Direct N2O emissions from rice paddy fields: summary of available data. Global Biochem. Cycles, 19(1), GB1005. doi:10.1029/2004GB002378
- Brons HJ, Hagen WR, Zehnder AJ 1991: Ferrous iron dependent nitric oxide production in nitrate-reducing cultures of Escherichia coli. Arch. Microbiol., 155(4), 341–347. doi:10.1007/BF00243453
- Chen Z, Liu J, Wu M, Xie X, Wu J, Wei W 2012: Differentiated response of denitrifying communities to fertilization regime in paddy soil. Microb. Ecol., 63(2), 446–459. doi:10.1007/s00248-011-9909-5
- Francis CA, O’Mullan GD, Cornwell JC, Ward BB 2013: Transitions in nirS-type denitrifier diversity, community composition, and biogeochemical activity along the Chesapeake Bay estuary. Front Microbiol., 4, 237. doi:10.3389/fmicb.2013.00237
- Hallin S, Philippot L, Löffler FE, Sanford RA, Jones CM 2018: Genomics and ecology of novel N2O-reducing microorganisms. Trends Microbiol., 26(1), 53–55. doi:10.1016/j.tim.2017.07.003
- Huang S, Chen C, Yang X, Wu Q, Zhang R 2011: Distribution of typical denitrifying functional genes and diversity of the nirS-encoding bacterial community related to environmental characteristics of river sediments. Biogeosciences, 8, 3041–3051. doi:10.5194/bg-8-3041-2011
- Ishii S, Ikeda S, Minamisawa K, Senoo K 2011: Nitrogen cycling in rice paddy environments: past achievements and future challenges. Microbes Environ., 26(4), 282–292. doi:10.1264/jsme2.ME11293
- Itoh H, Ishii S, Shiratori Y, Oshima K, Otsuka S, Hattori M, Senoo K 2013: Seasonal transition of active bacterial and archaeal communities in relation to water management in paddy soils. Microbes Environ., 28(3), 370–380. doi:10.1264/jsme2.ME13030
- Jones CM, Graf DR, Bru D, Philippot L, Hallin S 2013: The unaccounted yet abundant nitrous oxide-reducing microbial community: a potential nitrous oxide sink. Isme J, 7(2), 417–426. doi:10.1038/ismej.2012.125
- Lou Y, Ren L, Li Z, Zhang T, Inubushi K 2007: Effect of rice residues on carbon dioxide and nitrous oxide emissions from a paddy soil of subtropical China. Water Air Soil Poll., 178(1), 157–168. doi:10.1007/s11270-006-9187-x
- Masuda Y, Itoh H, Shiratori Y, Isobe K, Otsuka S, Senoo K 2017: Predominant but previously-overlooked prokaryotic drivers of reductive nitrogen transformation in paddy soils, revealed by metatranscriptomics. Microbes Environ., 32(2), 180–183. doi:10.1264/jsme2.ME16179
- Meyer F, Paarmann D, D'Souza M, Olson R, Glass EM, Kubal M, Paczian T, et al. 2008. "The metagenomics RAST server - a public resource for the automatic phylogenetic and functional analysis of metagenomes." BMC Bioinformatics. 9, 386. doi:10.1186/1471-2105-9-386.
- Plate L, Marletta MA 2012: Nitric oxide modulates bacterial biofilm formation through a multicomponent cyclic-di-GMP signaling network. Mol. Cell, 46(4), 449–460. doi:10.1016/j.molcel.2012.03.023
- Schreiber F, Wunderlin P, Udert KM, Wells GF 2012: Nitric oxide and nitrous oxide turnover in natural and engineered microbial communities: biological pathways, chemical reactions, and novel technologies. Front Microbiol., 3, 372. doi:10.3389/fmicb.2012.00372
- Smith CJ, Nedwell DB, Dong LF, Osborn AM 2007: Diversity and abundance of nitrate reductase genes (narG and napA), nitrite reductase genes (nirS and nrfA), and their transcripts in estuarine sediments. Appl. Environ. Microbiol., 73(11), 3612–3622. doi:10.1128/AEM.02894-06
- Tago K, Ishii S, Nishizawa T, Otsuka S, Senoo K 2011: “Phylogenetic and functional diversity of denitrifying bacteria isolated from various rice paddy and rice-soybean rotation fields. Microbes Environ., 26(1), 30–35. doi:10.1264/jsme2.ME10167
- Wei W, Isobe K, Nishizawa T, Zhu L, Shiratori Y, Ohte N, Koba K, Otsuka S, Senoo K 2015: Higher diversity and abundance of denitrifying microorganisms in environments than considered previously. Isme J, 9(9), 1954–1965. doi:10.1038/ismej.2015.9
- Xiong ZQ, Huang TQ, Ma YC, Xing GX, Zhu ZL 2010: Nitrate and ammonium leaching in variable- and permanent- charge paddy soils. Pedosphere, 20(2), 209–216. doi:10.1016/S1002-0160(10)60008-2
- Yoshida M, Ishii S, Fujii D, Otsuka S, Senoo K 2012: Identification of active denitrifiers in rice paddy soil by DNA- and RNA-based analyses. Microbes Environ., 27(4), 456–461. doi:10.1264/jsme2.ME12076
- Yoshida M, Ishii S, Otsuka S, Senoo K 2009: Temporal shifts in diversity and quantity of nirS and nirK in a rice paddy fields. Soil Biol. Biochem., 41(10), 2044–2051. doi:10.1016/j.soilbio.2009.07.012
- Zhou K, Qiao K, Edgar S, Stephanopoulos G 2015: Distributing metabolic pathway among a microbial consortium enhances production of natural products. Nat. Biotechnol., 33(4), 377–383. doi:10.1038/nbt.3095
- Zumft WG 1997: Cell biology and molecular basis of denitrification. Microbiol. Mol. Biol. Rev., 61(4), 533–616.