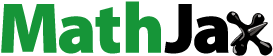
ABSTRACT
Green manure is an efficient nitrogen (N) source when used as an alternative to chemical fertilizer. However, the N taken up by rice derived from green manure, chemical fertilizers or soil native N in complex nutrient systems is unclear. A pot experiment with partial substitution of urea with Chinese milk vetch (a green manure) implemented with 15N-labeled urea and Chinese milk vetch was set up to study the sources of N in rice and the fate of the fertilizers. The dry weights, N contents, N uptake, and urea N use efficiency were notably higher (by 15–16%, 4–13%, 22–30% and 182%-203%, respectively) in the Chinese milk vetch applied with urea treatment than in the urea alone treatment. The uptake of N from Chinese milk vetch and the use efficiency of Chinese milk vetch N were increased with reductions in the urea input amount. The application of Chinese milk vetch substantially changed the fate of urea: higher amounts of urea N were taken up by rice (approximately 29%) and remained as residue in the soil (approximately 15%) in the related treatments than in the treatment with urea alone (10% and 9%). More urea N than Chinese milk vetch N was taken up by rice (29% vs 20%, respectively) and lost (56% vs 14%, respectively), but less urea N than Chinese milk vetch N remained as residue in the soil (15% vs 66%, respectively). The partial substitution of chemical fertilizer with green manure is an effective method of promoting rice growth by supplying N for rice uptake and promoting more efficient N use.
1. Introduction
Rice (Oryza sativa L.) is an important grain (Atique Ur et al. Citation2018). Its planting area and production are greater in China than in any other country (Xie et al. Citation2017). Nitrogen (N) is the main limiting nutrient element in paddy fields (Zhu et al. Citation2018). Thus, a large amount of N fertilizer is needed to meet the demand for rice production (Wang et al. Citation2017). However, in paddy fields in southern China, chemical N fertilizers are typically applied at a rate of 180 to 200 kg N ha−1 per planting season (Zhu et al. Citation2014), which may be greater than the amount needed by rice. Excessive applications of chemical N fertilizer increase farmers’ input costs, bring about low N use efficiency (Wang et al. Citation2017; Liu et al. Citation2018), and bring about many environmental problems (Vitousek et al. Citation2009; Liu et al. Citation2013; He et al. Citation2018). The loss of input N from agroecosystems has been estimated to exceed 1.7 million tons year−1 in China, making it the main contributor to the pollution of water systems (Yu, Xue, and Yang Citation2014; Yu et al. Citation2017; Yu, Ji, and Zhao Citation2018; Wang et al. Citation2019). Rational application of N fertilizer is very important for sustainable rice production.
The use of green manures (GMs) cultivated in agroecosystems is an alternative approach that can be used to solve the problem of excessive N fertilizer application (Zhang et al. Citation2016) and to improve rice production (Lee et al. Citation2010; Zhu et al. Citation2012). Chinese milk vetch (Astragalus sinicus L., CMV) is a common type of GM that has the unique ability to fix atmospheric N2 via root nodules and promotes substantial biomass and nutrient accumulation. Many studies have demonstrated that CMV is an excellent choice as an alternative to traditional N fertilizer (Huang et al. Citation2013; Lv et al. Citation2013). However, the characteristics of fertilizer supply from CMV are different from those of inorganic fertilizer, as the nutrients in CMV are released slowly with decomposition after the CMV is returned to the field. The N supply capacity at the early stage of GM use is its limiting factor, but its continuous nutrient supply is better than that of chemical fertilizer. Earlier studies found that the amount of N provided by GM alone is equivalent to 30–108% of that provided by inorganic fertilizer (Asagi and Ueno Citation2009), but there were differences in N uptake efficiency among GMs. These differences may be influenced by the differences in mineralizing characteristics among GMs. GM supplied with inorganic fertilizer provided more N than inorganic fertilizer or GM alone. Earlier studies found that CMV is equivalent to 100–160% of urea when CMV is supplied with inorganic fertilizer (Zhu et al. Citation2014). However, CMV improved chemical fertilizer N recovery efficiency in rice production (Zhu et al. Citation2014) due to a decrease in the risk of nitrate N leaching (Rinnofner et al. Citation2008) and the maintenance of soil fertility and biological activity (Larkin Citation2008). Thus, a viable option is to grow the GM crop and apply it to the soil to reduce the application of synthetic N fertilizer and to improve subsequent crop productivity (Liu et al. Citation2017).
However, in such a complex nutrient system, it is difficult to ascertain the specific sources of N taken up by the rice. Thus, a method using 15N-labeled plant materials has been used to directly calculate plant N uptake from GMs (Nishida, Sumida, and Kato Citation2008; Asagi and Ueno Citation2009; Zhao et al. Citation2015). However, there is a lack of accurate estimation of the N derived from soil, inorganic fertilizer, and green manure. As a consequence, it is crucial to estimate the amount of N supply and the N uptake by plants from the applied fertilizers in order to improve crop production. The objectives of this study were (1) to investigate the characteristics of urea or fresh CMV N supply for different amounts of urea; (2) to evaluate N uptake by rice from the 15N-labeled urea or CMV under different managements and (3) to determine the fates of 15N-labeled urea and CMV and their use efficiencies in a rice system.
2. Materials and methods
2.1. Soil samples
Paddy soil (0–20 cm) was collected from an arable field in Ningbo, Zhejiang Province, located in the subtropical region of southern China (121°54′E, 29°46′N; altitude: 4 m). the annual mean air temperature is 16.4°C, the annual mean precipitation is 1480 mm and approximately 60% precipitation occurs from May to September. Soil samples were air-dried at room temperature for 7 days and sieved through a 2-mm mesh to remove stones and visible roots. The main properties of the topsoil are shown in .
Table 1. The agrocharacteristics of paddy topsoil prior to the experiment
2.2. Preparation of 15N-labeled green manure
CMV (Zhezi NO.5) was cultivated in two troughs (25 cm width, 100 cm length and 15 cm height), one supplied with 15N-labeled urea (10.30% atom 15N excess) at a rate of 100 mg N kg−1 soil and the other supplied with the same amount of ordinary urea. CMV was harvested at the full bloom stage, and each whole plant was cut into small pieces (less than 5 cm in length) before incorporation. A subsample of the fresh residue of CMV was taken to measure the ratio of fresh to dry weight. Another subsample was oven dried at 75°C and ground with a grinder to analysis the total N content and 15N atom%. The total N content of the dry CMV residue was analyzed with a CNS elemental analyzer (Vario MAX, elementar analysensysteme GmbH, Germany), and 15N enrichment was measured with a stable isotope ratio mass spectrometer (Flash 2000 HT/Conflo IV/Delta V, Thermo Fisher Scientific, Germany). The fresh CMV contained 42.0 g C kg−1, 4.0 g N kg−1, a C-to-N ratio of 10.5, a moisture content of 89.6% and a 15N atom% in labeled and unlabeled CMV of 3.37% and 0.365%, respectively.
2.3. Experimental setup
A pot experiment (the pot with the 20 cm diameter and 22 cm height) was set up with 6 treatments, which were the control (CK), 15N-labeled urea (U), CMV applied with 80% 15N-labeled urea (15UC1), 15N-labeled CMV applied with 80% urea (U15C1), CMV applied with 60% 15N-labeled urea (15UC2) and 15N-labeled CMV applied with 60% urea (U15C2). The 15N-labeled urea was 10.30% atom 15N excess and 15N-labeled CMV was 3.37% atom 15N excess. Hereafter, UC1 is used to refer to 15UC1 and U15C1 when considered together; similarly, UC2 is used for 15UC2 and U15C2 when considered together. For the U treatment, the urea was added at a concentration of 100 mg N kg−1 soil. For the UC1 treatments, the urea was added at a concentration of 80 mg N kg−1 soil, and CMV was added at a concentration of 8 g fresh CMV kg−1 soil (32 mg N kg−1 soil), which were local fertilizer recommendations (Wan et al. Citation2015). In total, the amount of total-N was 112 mg N kg−1 soil. For the UC2 treatments, the urea was added at a concentration of 60 mg N kg−1 soil, and CMV was added the same to the UC1 treatments. In total, the amount of total-N was 92 mg N kg−1 soil. Each pot, with 3 kg of soil (dry weight), was prepared for rice planting. Phosphorus and potassium fertilizers were applied for all treatments in the form of monopotassium phosphate at a rate of 100 mg P2O5 kg−1 dry soil and 60 mg K2O kg−1 dry soil, respectively. The fertilizer and CMV were blended with the soil. Then, distilled water was added to reach a 105% water-holding capacity. The soil was preincubated for 10 days before transplanting the rice. Four replicates were used for each treatment.
Rice seeds (Zaoxian No. 1) were sterilized using 10% H2O2 for 5 min and then washed thoroughly with sterile water in order to improve the germination rate. Rice seeds were placed at 25°C for pregermination, and three seeds were cultivated in each pot. Flooding conditions were maintained after the rice plants sprouted leaves, and the environmental conditions included natural illumination with a temperature maintained at 32 ± 4°C (day) and 28 ± 4°C (night). Rice was harvested at the maturity stage (110 days after cultivating). Destructive sampling was conducted at harvest. A small part of the soil was air-dried and used to analyze the abundance of 15N.
Plant shoots and roots were collected separately at harvest, de-enzymed at 105℃ for 30 mins and then oven dried at 75°C, measured for dry weight and ground to a powder. The total N concentration and abundance of 15N in the subsamples of the plants were determined using a mass spectrometer (Integra CN; Sercon Ltd., Cheshire, UK).
2.4. Calculations
The percentage of N derived from applied urea (%Ndfau) and from CMV (%NdfaCMV) were calculated from EquationEquation (1)(1)
(1) , where a is the atom% of 15N in rice plant or soil, b is the atom% of 15N of the added urea or CMV, and 0.365 means the natural atom% of 15N.
The percentage of N derived from applied soil (Ndfas) was calculated from EquationEquation (2)(2)
(2) .
The amount of N derived from applied urea (Ndfau), from CMV (NdfaCMV) and from soil were calculated from EquationEquation (3)(3)
(3) . Where M means the dry weight of rice and CN means rice N content.
The N use efficiency (NUE) is the use rate of N by rice from the applied N source. The NUE rates of urea (NUEu), CMV (NUECMV) and total applied fertilizers (NUEaN) were calculated from EquationEquation (4)(4)
(4) . Where c is N uptake derived from the applied N in rice plant at harvest,d is the amount of N applied in CMV or urea.
The added N interaction (ANI) is defined as effects of the fertilizer N addition on the uptake of soil N by crop (Asagi and Ueno Citation2009). ANI was calculated from EquationEquation (5)(5)
(5)
Fate of urea or CMV N includes three parts: uptake (%NU), residual (%NR), and lost (%NL).
The fates of urea N uptake by rice (%NU), residual in soil (%NR) and lost (%NL) were calculated from EquationEquations (6(6)
(6) )–(Equation8
(8)
(8) ).
where e is atom% of 15N in soil and f is atom% of 15N of the added urea, M means dry soil weight and CN means soil N content.
The fates of CMV N uptake by rice (%NU), residual in soil (%NR) and lost (%NL) were calculated from EquationEquations (9(9)
(9) )–(Equation11
(11)
(11) ).
where e is atom% of 15N in soil and f is atom% of 15N of the added CMV, M means dry soil weight and CN means soil N content.
2.5. Data analysis
A repeated measure analysis of variance (ANOVA) was used to test the effects of treatment (no fertilizer, urea only and CMV substitute partial urea). The differences of the dry weights, N contents, N uptake, and NUE were tested using the least significant difference (LSD) test at the 0.05 probability level. The differences of CMV fate were tested using student’s T-test at 0.05 probability level. All statistical analyses were conducted using the statistical software SPSS 20.0 (SPSS Inc., Chicago, IL, USA)
3. Results
3.1. Rice dry weight and nitrogen uptake
Rice growth was significantly (p < 0.05) higher in the U, UC1 and UC2 treatments than in the CK treatment, and the dry weights and N contents were significantly increased by 92–123% and 26–40%, respectively (). Accordingly, these practices increased N uptake by rice by 146–219% in the U, UC1 and UC2 treatments than in the CK treatment, respectively. Compared with the U treatment, CMV applied with urea significantly increased dry weights and N contents by 15–16% and 4–13%, respectively, and increased the N uptake by 22–30%. However, there was no significant difference in dry weight, N content or N uptake by rice between the UC1 and UC2 treatments.
Table 2. Rice dry weight, N content and N uptake in shoots and roots affected by urea and Chinese milk vetch application
3.2. Fertilizers nitrogen use efficiency
N uptake derived from urea was most significant in the UC1 treatment (72.2 mg N pot−1), followed by the UC2 treatments (50.4 mg N pot−1) (). However, in the case of the NUEU, there were no significant differences between the UC1 and UC2 treatments (). Further, N uptake from CMV (23.2 mg N pot−1) and the NUECMV (23%) were higher in the UC2 treatment than in the UC1 treatment (17.0 mg N pot−1 and 16%). In general, the NUEaN did not differ between the UC1 and UC2 treatments but was significantly higher in these two treatments than in the U treatment. Finally, N uptake from the soil in the UC2 (129.4 mg N pot−1) and U (127.3 mg N pot−1) treatments were higher than that in the UC1 treatment (102.7 mg N pot−1). The ANI was 69% and 64% higher in the UC2 and U treatments than in the UC1 treatment, respectively ().
Table 3. Rice N use efficiency (NUE) affected by urea and Chinese milk vetch application
Figure 1. Distribution of nitrogen derived from applied urea, Chinese milk vetch (CMV) and soil in the total N taken up by the rice. U means 100% urea as nitrogen fertilization; UC1 means 80% urea plus Chinese milk vetch as nitrogen fertilization; UC2 means 60% urea plus Chinese milk vetch as nitrogen fertilization. The vertical bars indicating the standard errors of three replicates
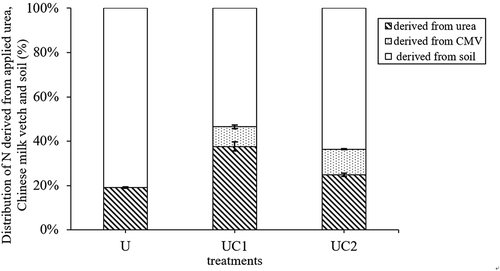
3.3. Fertilizers nitrogen residual and loss
The amount of residual urea N was not significantly (p < 0.05) different in these three treatments (27.8, 30.3, and 30.3 ug N pot−1) (). Compared with the amount of urea that put in, the proportion of residual urea was significantly (p < 0.05) higher in the UC2 treatment than in the U treatment: 9 and 16% of the added urea N remained in the U and UC2 treatments, respectively. However, the residual urea in the UC1 treatment (12%) did not differ significantly from that in the other treatments. Accordingly, CMV applied with urea significantly decreased the loss of urea N (58% and 55% of the added urea N) compared with the U treatment (81%).
Figure 2. Fate of urea N (uptake, residual and loss) as affected by urea and Chinese milk vetch (CMV) application. Uptake means urea N uptake by rice, residual means urea N residual in soil and loss means urea N lost by ammonia volatilization or denitrification. U means 100% urea as nitrogen fertilization; UC1 means 80% urea plus Chinese milk vetch as nitrogen fertilization; UC2 means 60% urea plus Chinese milk vetch as nitrogen fertilization. The vertical bars indicating the standard errors of three replicates
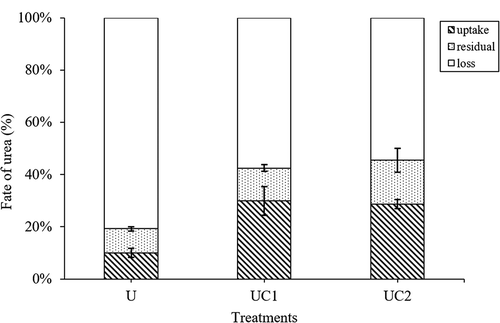
Since the reduction in urea application, the fate of CMV was different between the UC2 and UC1 treatments (). A further reduction in urea significantly increased residual CMV N in the soil from 63.2 to 74.2 mg pot−1, and decreased CMV N loss from 23.5 to 6.6 mg pot−1. The fates of urea N and CMV N were also different: more urea N than CMV N was lost (57% vs 14%, respectively), but less residual urea N than residual CMV N remained in the soil (14% vs 66%, respectively).
Figure 3. Fate of Chinese milk vetch N (uptake, residual and loss) as affected by urea and Chinese milk vetch application. Uptake means Chinese milk vetch N uptake by rice, residual means Chinese milk vetch N residual in soil and loss means Chinese milk vetch N lost by ammonia volatilization, denitrification, etc. UC1 means 80% urea plus Chinese milk vetch as nitrogen fertilization; UC2 means 60% urea plus Chinese milk vetch as nitrogen fertilization. The vertical bars indicating the standard errors of three replicates
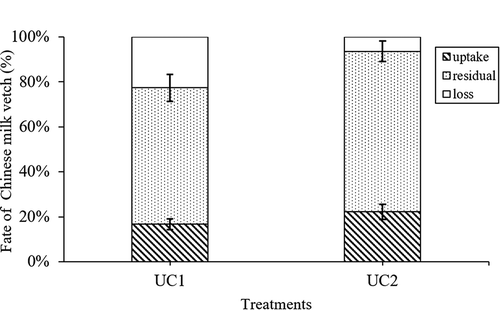
4. Discussion
4.1. Effects of CMV and urea on rice growth and N uptake
In this study, we found that partial substitution of urea with CMV improved rice dry weights and N uptake (). It is because CMV input provided N for rice, as well as promoted rice absorption of urea nitrogen and soil native nitrogen. Zhu et al. (Citation2014) observed that approximately 50% of CMV N was released within the first 10 days after incorporation, and NH4+-N was increased from 80 to 125 mg kg−1 soil. Our results showed that the N taken up by rice derived from CMV was approximately 11% (), similar to the findings of many field and pot experiments (Crews and Peoples Citation2005; Douxchamps et al. Citation2011; Li et al. Citation2015). This source of N not only compensated for the reduced urea N, but also continuously supplied N throughout the rice growing season. Hence, CMV N was released rapidly and intensely, maintaining soil NH4+-N and NO3–N contents, Quan et al. (Citation2016) reported that ryegrass added as green manure did not decrease soil total NO3− accumulation due to its own decay. In addition, 94–96% of N was released continually and slowly in the next 133 days, this N release from CMV could meet the N demand for rice plant growth (Zhu et al. Citation2014).
The amount of the urea in UC1 and UC2 treatments were reduced, but more urea N were uptake by rice than the U treatment (), and urea N use efficiency is higher than the U treatment (). CMV application had effects on urea N release characteristics. The reason for this result is that applying CMV increased urea N immobilization in the early season and improved its subsequent mineralization (Zhang et al. Citation2017; Cao et al. Citation2018). N immobilization in paddy soils is strongly determined by redox conditions and the easily degradable organic matter (Said-Pullicino et al. Citation2014). The addition of CMV supplied labile organic C to heterotrophic microorganisms, leading to an increase in biotic immobilization (Cucu et al. Citation2014; Said-Pullicino et al. Citation2014), which is in line with the results of Quan et al. (Citation2016), who observed that ryegrass coapplication significantly increased 15N-labeled NH4+ compared with soil organic N applied alone from 3% to 17%.
Only 37 to 47% of N was derived from CMV and chemical fertilizers, and most N was from the soil. The result from this study suggested that rice uptake of soil N was significantly higher with fertilizer addition than in the CK treatment. Liu et al. (Citation2017), through a meta-analysis, found that both organic and inorganic fertilizers significantly increased plant uptake of native soil N, consistent with the present study. However, rice uptake of N derived from soil and CMV in the UC1 treatment were lower than UC2 treatment, but no differences in NUEU and NUEaN between UC1 and UC2 treatments. This is maybe because urea N gives priority to soil native N and CMV N (Zhu et al. Citation2014; Li et>al. Citation2015).
In addition, Biological nitrogen fixation does exist in rice ecosystems (Ma et>al. Citation2019), atmospheric N2 can be incorporated into surface water, soil and even the rhizosphere under rice paddy field conditions by biological Nfixation. Some N2-fixing bacteria (e.g., Herbaspirillum sp. and Azospirillum sp.) reside inside rice plants as endophytes (Mano and Morisaki Citation2008; Kaga etal. Citation2009), and promote plant growth (Walley etal. Citation2007; Isawa etal. Citation2010; Liao etal., Citation2018a). However, the amount of nitrogen fixation by asymbiotic microorganisms only contributed 0.2–2.0mg Nkg−1 soil in paddy soil systems (Li, Pan, and Yao Citation2018b; Liao etal., Citation2018b), and rice is more likely to use fertilizers Nand soil Ndirectly rather than investing in asymbiotic nitrogen fixation (Perez-Fernandez and Lamont Citation2016). Therefore, this process was not considered when we analyzed the fate of N.
No differences in dry weight were found among CMV applied treatments (). This lack of differences could be explained by the fact that excessive use of fertilizers may not continue to increase production. Qiao et al. (Citation2012) found that using approximately 50–100% of the conventional N application rate made no significant difference in grain yield. Similar results have been observed in many different regions in China (Zhang et al. Citation2016). These results suggest that substituting 40% urea with CMV may be the best choice.
4.2. Fates of urea N and CMV N
The present study showed that only 30.1 mg pot−1 (about 10% of total input urea N) urea N recovered by rice and 27.8 mg pot−1 (about 9% of total input urea N) residual in soil in the U treatment ( and ), which was lower than the results of other studies. This discrepancy is possibly due to the higher pH under our conditions (Ishii et al. Citation2011; Wang et al. Citation2016; Li et al. Citation2018a), which leads to more urea N lost through ammonia volatilization (Li et al. Citation2015) and denitrification (Jiang et al. Citation2015; Lan, Han, and Cai Citation2015; Liao et al. Citation2018b). In addition, the urea we used is for laboratory, which is easier to dissolve and volatilize than the urea which is used for agricultural production.
CMV application significantly changed the fate of urea N, and more urea N was uptake (). This could be explained by CMV application increasing UREU (). However, there were little differences in the percentage of urea N residual between CMV applied and no CMV treatments. The low C:N ratio of the CMV may cause no net immobilization during the decomposition of the CMV though concurrent immobilization and mineralization caused some uptake of urea N.
CMV application have significantly reduced the percentages of urea N lost (), in addition to more absorption, which may be due to the reduction in urea. Replacing approximately 20% to 40% of total urea with CMV consequently reduced excessive N losses through NH3 volatilization and N2O emission from a rice-based rotation system, thereby essentially increasing N recovery (Xie et al. Citation2017).
showed that approximately 20% of input CMV N was absorbed by rice, and approximately 60–70% was residual in the soil. Crews and Peoples (Citation2005) showed that almost 58% of 15N-labeled legume material was residual in soil and approximately 24% was lost. A similar percentage of GM N in soil was reported by Li et al. (Citation2015), who reported that 22% of added GM N was taken up by wheat and approximately 52% was residual in the soil over a two-year field experiment. In total, 18% of N was taken up in the first year, and 4% was taken up in the second year. This result could be explained by N applied through CMV being a good partial substitute for urea. If we considered total fertilizers N residual in soil and N uptake by roots, the net N residual in soil (total fertilizers N residual in the soil plus roots uptake N minus rice uptake N derived from the soil) were 65.7 and 49.3 mg·pot−1 in UC1 and UC2 treatments, respectively. Hence, the N applied through CMV is beneficial for the next crop (Edmeades Citation2003).
The fates of urea and CMV were significantly different ( and ). Urea N was easily taken up and lost due to urea N being easily hydrolyzed to NH4+-N after being input to soil. More CMV N was residual in soil because most CMV N residues in soil were organic N, which was not easily volatilized or leached. In addition, after 120 days since CMV was applied to the soil, a small amount of the CMV still existed in the soil in the form of plant residues. In addition, this part of CMV N was unlikely to be detected through a 15N-isotope-labeled experiment. Asagi and Ueno (Citation2009) used the bag method to test GM residuals, and they found that 24 to 54% of GMs were residual in the soil after 86 days since green manure applied to the soil. Zhu et al. (Citation2014) used the same method and found that approximately 5.5% of CMV residue remained in bags. If we consider a longer timescale, CMV may be better for the next planting season.
5. Conclusion
Our results indicated that partially substituting chemical fertilizer with CMV is an effective method of promoting rice growth, especially in alkaline soils. This effectiveness occurred because CMV supplied approximately 11% of the N taken up by rice, and CMV promoted urea N immobilization and release to increase NUEU. Compared with the fate of urea N, most CMV N was residual in the soil, which is beneficial for subsequent plant growth. Further research should be conducted in field experiments to evaluate the impacts of different soil conditions and determine the microbial community structure to verify the results in the present study.
Acknowledgments
We thank Yongxiang Yu, Xien long, Xuechen Zhang, Xing Li, Jiantao Xue, Yuzhen luo, Ningguo Zheng for technical assistance.
Disclosure statement
No potential conflict of interest was reported by the authors.
Additional information
Funding
References
- Asagi, N., and H. Ueno. 2009. “Nitrogen Dynamics in Paddy Soil Applied with Various (15)n-labelled Green Manures.” Plant and Soil 322: 251–262. doi:10.1007/s11104-009-9913-4.
- Atique Ur, R., M. Farooq, A. Rashid, F. Nadeem, S. Stuerz, F. Asch, B. RW, and S. KHM. 2018. “Boron Nutrition of Rice in Different Production Systems. A Review.” Agronomy for Sustainable Development 38: 24. doi:10.1007/s13593-018-0504-8.
- Cao, Y. S., H. F. Sun, J. N. Zhang, G. F. Chen, H. T. Zhu, S. Zhou, and H. Y. Xiao. 2018. “Effects of Wheat Straw Addition on Dynamics and Fate of Nitrogen Applied to Paddy Soils.” Soil & Tillage Research 178: 92–98. doi:10.1016/j.still.2017.12.023.
- Crews, T. E., and M. B. Peoples. 2005. “Can the Synchrony of Nitrogen Supply and Crop Demand Be Improved in Legume and Fertilizer-based Agroecosystems? A Review.” Nutrient Cycling in Agroecosystems 72: 101–120. doi:10.1007/s10705-004-6480-1.
- Cucu, M. A., D. Said-Pullicino, V. Maurino, E. Bonifacio, M. Romani, and L. Celi. 2014. “Influence of Redox Conditions and Rice Straw Incorporation on Nitrogen Availability in Fertilized Paddy Soils.” Biology and Fertility of Soils 50: 755–764. doi:10.1007/s00374-013-0893-4.
- Douxchamps, S., E. Frossard, S. M. Bernasconi, R. van der Hoek, A. Schmidt, I. M. Rao, and A. Oberson. 2011. “Nitrogen Recoveries from Organic Amendments in Crop and Soil Assessed by Isotope Techniques under Tropical Field Conditions.” Plant and Soil 341: 179–192. doi:10.1007/s11104-010-0633-6.
- Edmeades, D. C. 2003. “The Long-term Effects of Manures and Fertilisers on Soil Productivity and Quality: A Review.” Nutrient Cycling in Agroecosystems 66: 165–180. doi:10.1023/a:1023999816690.
- He, L. L., Y. C. Bi, J. Zhao, C. M. Pittelkow, X. Zhao, S. Q. Wang, and G. X. Xing. 2018. “Population and Community Structure Shifts of Ammonia Oxidizers after Four-year Successive Biochar Application to Agricultural Acidic and Alkaline Soils.” The Science of the Total Environment 619: 1105–1115. doi:10.1016/j.scitotenv.2017.11.029.
- Huang, J., J. S. Gao, S. J. Liu, W. D. Cao, and Y. Z. Zhang. 2013. “Effect of Chinese Milk Vetch in Winter on Rice Yield and Its Nutrient Uptake.” Soil and Fertilizer Sciences in China (1): 88–92. in Chinese.
- Isawa, T., M. Yasuda, H. Awazaki, K. Minamisawa, S. Shinozaki, and H. Nakashita. 2010. “Azospirillum Sp Strain B510 Enhances Rice Growth and Yield.” Microbes and Environments / JSME 25: 58–61. doi:10.1264/jsme2.ME09174.
- Ishii, S., S. Ikeda, K. Minamisawa, and K. Senoo. 2011. “Nitrogen Cycling in Rice Paddy Environments: Past Achievements and Future Challenges.” Microbes and Environments / JSME 26: 282–292. doi:10.1264/jsme2.ME11293.
- Jiang, X. J., X. Y. Hou, X. Zhou, X. P. Xin, A. Wright, and Z. J. Ji. 2015. “pH Regulates Key Players of Nitrification in Paddy Soils.” Soil Biology & Biochemistry 81: 9–16. doi:10.1016/j.soilbio.2014.10.025.
- Kaga, H., H. Mano, F. Tanaka, A. Watanabe, S. Kaneko, and H. Morisaki. 2009. “Rice Seeds as Sources of Endophytic Bacteria.” Microbes and Environments / JSME 24: 154–162. doi:10.1264/jsme2.ME09113.
- Lan, T., Y. Han, and Z. C. Cai. 2015. “Denitrification and Its Product Composition in Typical Chinese Paddy Soils.” Biology and Fertility of Soils 51: 89–98. doi:10.1007/s00374-014-0953-4.
- Larkin, R. P. 2008. “Relative Effects of Biological Amendments and Crop Rotations on Soil Microbial Communities and Soilborne Diseases of Potato.” Soil Biology & Biochemistry 40: 1341–1351. doi:10.1016/j.soilbio.2007.03.005.
- Lee, C. H., K. D. Park, K. Y. Jung, M. A. Ali, D. Lee, J. Gutierrez, and P. J. Kim. 2010. “Effect of Chinese Milk Vetch (astragalus Sinicus L.) As a Green Manure on Rice Productivity and Methane Emission in Paddy Soil.” Agriculture, Ecosystems & Environment 138: 343–347. doi:10.1016/j.agee.2010.05.011.
- Li, F. C., Z. H. Wang, J. Dai, Q. Li, X. Wang, C. Xue, H. Liu, and G. He. 2015. “Fate of Nitrogen from Green Manure, Straw, and Fertilizer Applied to Wheat under Different Summer Fallow Management Strategies in Dryland.” Biology and Fertility of Soils 51: 769–780. doi:10.1007/s00374-015-1023-2.
- Li, Y. Y., S. J. Chapman, G. W. Nicol, and H. Y. Yao. 2018a. “Nitrification and Nitrifiers in Acidic Soils.” Soil Biology & Biochemistry 116: 290–301. doi:10.1016/j.soilbio.2017.10.023.
- Li, Y. Y., F. X. Pan, and H. Y. Yao. 2018b. “Response of Symbiotic and Asymbiotic Nitrogen-fixing Microorganismsto Nitrogen Fertilizer Application.” Journal of Soils and Sediments. doi:10.1007/s11368-018-2192-z.
- Liao, H. K., S. J. Chapman, Y. Y. Li, and H. Y. Yao. 2018. “Dynamics of Microbial Biomass and Community Composition after Short-term Water Status Change in Chinese Paddy Soils.” Environmental Science and Pollution Research 25: 2932–2941. doi:10.1007/s11356-017-0690-y.
- Liao, H. K., Y. Y. Li, and H. Y. Yao. 2018. “Fertilization with Inorganic and Organic Nutrients Changes Diazotroph Community Composition and N-fixation Rates.” Journal of Soils and Sediments 18: 1076–1086. doi:10.1007/s11368-017-1836-8.
- Liu, X. J., Y. Zhang, W. X. Han, A. H. Tang, J. L. Shen, Z. L. Cui, P. Vitousek, et al. 2013. “Enhanced Nitrogen Deposition over China.” Nature 494: 459–462. doi:10.1038/nature11917.
- Liu, X. J. A., K. J. van Groenigen, P. Dijkstra, and B. A. Hungate. 2017. “Increased Plant Uptake of Native Soil Nitrogen following Fertilizer Addition - Not a Priming Effect?” Applied Soil Ecology 114: 105–110. doi:10.1016/j.apsoil.2017.03.011.
- Liu, Y. H., H. D. Zang, T. D. Ge, J. Bai, S. B. Lu, P. Zhou, P. Q. Peng, et al. 2018. “Intensive Fertilization (N, P, K, Ca, and S) Decreases Organic Matter Decomposition in Paddy Soil.” Applied Soil Ecology 127: 51–57. doi:10.1016/j.apsoil.2018.02.012.
- Lv, Y. H., C. Z. Liu, Z. L. Pan, B. Y. Li, and W. D. Cao. 2013. “Soil Nutrients and Rice Yield as Affected by Different Incorporation Periods of Chinese Milk Veich.” Soil and Fertilizer Sciences in China (1): 85–87. in Chinese.
- Ma, J., Q. C. Bei, X. J. Wang, P. Lan, and G. Liu. 2019. “Impacts of Mo Application on Biological Nitrogen Fixation and Diazotrophic Communities in a Flooded Rice-soil System.” The Science of the Total Environment 649: 686–694. doi:10.1016/j.scitotenv.2018.08.318.
- Mano, H., and H. Morisaki. 2008. “Endophytic Bacteria in the Rice Plant.” Microbes and Environments / JSME 23: 109–117. doi:10.1264/jsme2.23.109.
- Nishida, M., H. Sumida, and N. Kato. 2008. “Fate of Nitrogen Derived from (15)n-labeled Cattle Manure Compost Applied to a Paddy Field in the Cool Climate Region of Japan.” Soil Science and Plant Nutrition 54: 459–466. doi:10.1111/j.1747-0765.2008.00255.x.
- Perez-Fernandez, M. A., and B. B. Lamont. 2016. “Competition and Facilitation between Australian and Spanish Legumes in Seven Australian Soils.” Plant Species Biology 31: 256–271. doi:10.1111/1442-1984.12111.
- Qiao, J., L. Z. Yang, T. M. Yan, F. X. Xue, and D. Zhao. 2012. “Nitrogen Fertilizer Reduction in Rice Production for Two Consecutive Years in the Taihu Lake Area.” Agriculture, Ecosystems & Environment 146: 103–112. doi:10.1016/j.agee.2011.10.014.
- Quan, Z., B. Huang, C. Y. Lu, Y. Shi, X. Chen, H. Y. Zhang, and Y. T. Fang. 2016. “The Fate of Fertilizer Nitrogen in a High Nitrate Accumulated Agricultural Soil.” Scientific Reports 6: 9. doi:10.1038/srep21539.
- Rinnofner, T., J. K. Friedel, R. de Kruijff, G. Pietsch, and B. Freyer. 2008. “Effect of Catch Crops on N Dynamics and following Crops in Organic Farming.” Agronomy for Sustainable Development 28: 551–558. doi:10.1051/agro:2008028.
- Said-Pullicino, D., M. A. Cucu, M. Sodano, J. J. Birk, B. Glaser, and L. Celi. 2014. “Nitrogen Immobilization in Paddy Soils as Affected by Redox Conditions and Rice Straw Incorporation.” Geoderma 228: 44–53. doi:10.1016/j.geoderma.2013.06.020.
- Vitousek, P. M., R. Naylor, T. Crews, M. B. David, L. E. Drinkwater, E. Holland, P. J. Johnes, et al. 2009. “Nutrient Imbalances in Agricultural Development.” Science 324: 1519–1520. doi:10.1126/science.1170261.
- Walley, F. L., G. W. Clayton, P. R. Miller, P. M. Carr, and G. P. Lafond. 2007. “Nitrogen Economy of Pulse Crop Production in the Northern Great Plains.” Agronomy Journal 99: 1710–1718. doi:10.2134/agronj2OO6.0314s.
- Wan, S. X., H. B. Zhu, S. Tang, X. S. Guo, and Y. Q. Wang. 2015. “Effects of Astragalus Sinicus Manure and Fertilizer Combined Application on Biological Properties of Soil in Anhui Double Cropping Rice Areas along the Yangtze River.” Journal of Plant Nutrition and Fertilizer 21: 387–395. in Chinese. doi: 10.11674/zwyf.2015.0213.
- Wang, J., S. J. Chapman, Q. Ye, and H. Y. Yao. 2019. “Limited Effect of Planting Transgenic Rice on the Soil Microbiome Studied by Continuous 13CO2 Labeling Combined with High-throughput Sequencing.” Applied Microbiology and Biotechnology 103: 4217–4227. doi:10.1007/s00253-019-09751-w.
- Wang, J., Y. Zhao, J. B. Zhang, W. Zhao, C. Muller, and Z. C. Cai. 2017. “Nitrification Is the Key Process Determining N Use Efficiency in Paddy Soils.” Journal of Soil Science and Plant Nutrition 180: 648–658. doi:10.1002/jpln.201700130.
- Wang, X. B., W. Zhou, G. Q. Liang, X. X. Pei, and K. J. Li. 2016. “The Fate of N-15-labelled Urea in an Alkaline Calcareous Soil under Different N Application Rates and N Splits.” Nutrient Cycling in Agroecosystems 106: 311–324. doi:10.1007/s10705-016-9806-x.
- Xie, Z. J., Y. Q. He, S. X. Tu, C. X. Xu, G. R. Liu, H. M. Wang, W. D. Cao, and H. Liu. 2017. “Chinese Milk Vetch Improves Plant Growth, Development and N-15 Recovery in the Rice-Based Rotation System of South China.” Scientific Reports 7: 11. doi:10.1038/s41598-017-03919-y.
- Yu, Y. L., L. H. Xue, and L. Z. Yang. 2014. “Winter Legumes in Rice Crop Rotations Reduces Nitrogen Loss, and Improves Rice Yield and Soil Nitrogen Supply.” Agronomy for Sustainable Development 34: 633–640. doi:10.1007/s13593-013-0173-6.
- Yu, Y. X., H. T. Ji, and C. Y. Zhao. 2018. “Evaluation of the Effects of Plastic Mulching and Nitrapyrin on Nitrous Oxide Emissions and Economic Parameters in an Arid Agricultural Field.” Geoderma 324: 98–108. doi:10.1016/j.geoderma.2018.03.012.
- Yu, Y. X., C. Y. Zhao, H. T. Jia, B. C. Niu, Y. Sheng, and F. Z. Shi. 2017. “Effects of Nitrogen Fertilizer, Soil Temperature and Moisture on the Soil-surface CO2 Efflux and Production in an Oasis Cotton Field in Arid Northwestern China.” Geoderma 308: 93–103. doi:10.1016/j.geoderma.2017.07.032.
- Zhang, D. B., P. W. Yao, N. Zhao, C. W. Yu, W. D. Cao, and Y. J. Gao. 2016. “Contribution of Green Manure Legumes to Nitrogen Dynamics in Traditional Winter Wheat Cropping System in the Loess Plateau of China.” European Journal of Agronomy 72: 47–55. doi:10.1016/j.eja.2015.09.012.
- Zhang, X. X., R. J. Zhang, J. S. Gao, X. C. Wang, F. L. Fan, X. T. Ma, H. Q. Yin, C. W. Zhang, K. Feng, and Y. Deng. 2017. “Thirty-one Years of Rice-rice-green Manure Rotations Shape the Rhizosphere Microbial Community and Enrich Beneficial Bacteria.” Soil Biology & Biochemistry 104: 208–217. doi:10.1016/j.soilbio.2016.10.023.
- Zhao, W., B. Liang, X. Y. Yang, and J. B. Zhou. 2015. “Fate of Residual N-15-labeled Fertilizer in Dryland Farming Systems on Soils of Contrasting Fertility.” Soil Science and Plant Nutrition 61: 846–855. doi:10.1080/00380768.2015.1066232.
- Zhu, B., L. X. Yi, L. M. Guo, G. Chen, Y. G. Hu, H. M. Tang, C. F. Xiao, et al. 2012. “Performance of Two Winter Cover Crops and Their Impacts on Soil Properties and Two Subsequent Rice Crops in Dongting Lake Plain, Hunan, China.” Soil & Tillage Research 124: 95–101. doi:10.1016/j.still.2012.05.007.
- Zhu, B., L. X. Yi, Y. G. Hu, Z. H. Zeng, C. W. Lin, H. M. Tang, G. L. Yang, and X. P. Xiao. 2014. “Nitrogen Release from Incorporated N-15-labelled Chinese Milk Vetch (astragalus Sinicus L.) Residue and Its Dynamics in a Double Rice Cropping System.” Plant and Soil 374: 331–344. doi:10.1007/s11104-013-1808-8.
- Zhu, Z. K., T. D. Ge, S. L. Liu, Y. J. Hu, R. Z. Ye, M. L. Xiao, C. L. Tong, Y. Kuzyakov, and J. S. Wu. 2018. “Rice Rhizodeposits Affect Organic Matter Priming in Paddy Soil: The Role of N Fertilization and Plant Growth for Enzyme Activities, CO2 and CH4 Emissions.” Soil Biology & Biochemistry 116: 369–377. doi:10.1016/j.soilbio.2017.11.001.