ABSTRACT
The thermal-activated serpentine prepared by heating natural serpentine at different temperatures was used to immobilize cadmium (Cd) in simulated contaminated soils. The results showed that the increasing soil pH induced by adding serpentine was primarily responsible for reducing the content of TCLP-Cd (toxicity characteristic leaching procedure-Cd) in soil. Furthermore, adding thermal-activated serpentine could promote the transformation from exchangeable form of Cd in soil to low bioavailable Cd (Fe-Mn oxides, carbonate, and residual form) by surface adsorption and surface precipitation, and then reduced the bioavailability of Cd in soil. Under the same treatment condition, adding S700 (serpentine activated at 700°C) exhibited better performance to immobilization of Cd, and it could reduce exchangeable Cd by 23.76~36.49%, and increase carbonate, Fe-Mn oxide, and residual form of Cd by 6.03~8.03%, 6.05~8.35%, and 11.17~19.58%, respectively. These results indicated that thermal-activated serpentine would be the great potential for immobilization of Cd in soil.
1. Introduction
With the rapid development of industry and agriculture, heavy metal pollution of farmland soil is becoming more and more serious. The high content of heavy metals in soil will lead to soil degradation, lower crop yields, and lower quality of agricultural products. Cadmium (Cd), as one of the typical heavy metal contaminants, has strong biological toxicity. Cd taken up by plants would poison the plants or enter human body through the food chain. Finally, it would endanger the health of the human body (Wang et al. Citation2009; Hashem et al. Citation2013; Sun et al. Citation2016). In addition, it is difficult to remove Cd from the soil due to its strong stability and the difficulty of degradation. Given the situation of large area soil seriously contaminated by Cd (Liang et al. Citation2014b), it has become an urgent task to develop an effective technology to treat Cd-contaminated soil.
There are usually two strategies to treat heavy metal contaminated soil. The first is to reduce the total amount of heavy metals by removing heavy metals directly from the soil. The second strategy is to change the existing form of metals in soil from unstable to stable form, and then reduce the mobility and bioavailability of heavy metals in the environment (Komárek, Vaněk, and Ettler Citation2013; Khan et al. Citation2017; Liu et al. Citation2018). The directly removal of heavy metals from the soil has the disadvantages of high cost and long implementing period. However, in-situ immobilization remediation technology, i.e., the heavy metal form was changed from unstable to stable form by adding immobilization agent to soil, cannot only reduce the bioavailability of heavy metals, but also have little influence on soil fertility and farmland tillage. At present, in-situ immobilization remediation of the soil contaminated by heavy metal has attracted more and more attention (Diels, van der Lelie, and Bastiaens Citation2002; Kumpiene, Lagerkvist, and Maurice Citation2008; Lee et al. Citation2009; Sun et al. Citation2012; Li and Xu Citation2015).
In-situ immobilization remediation of farmland contaminated by heavy metal is different from the remediation of construction land, especially in the selection of remediation material (heavy metal immobilization agent). The remediation material has not only high efficiency and stable immobilization effect, but also has no adverse effect on soil physical and chemical properties. In recent years, heavy metal immobilization agents have been extensively studied, and it was found that some natural or synthetic materials including phosphate, clay minerals, and red mud could stabilize the heavy metals in soil (Basta et al. Citation2001; Kumpiene, Lagerkvist, and Maurice Citation2008; Liang et al. Citation2011; Chang et al. Citation2013). Among these immobilization agents, the abundant and low-cost clay minerals with a large specific surface area and special crystal layer structure could well immobilize the heavy metals in the soil by adsorption, coordination, coprecipitation, and so on. More important, clay minerals, as one of the components of the soil, do not destroy the soil structure and the ecological environment (Hang et al. Citation2007). Therefore, it is considered as one of the most effective and feasible methods to treat heavy metal in soil by using clay minerals as immobilization agent. In recent years, the effects of zeolite, sepiolite, and montmorillonite on changing the heavy metal form and inactivating heavy metal (reducing availability) has been extensively investigated, and it found they could be more efficiently used for the immobilization remediation of heavy contaminated soils (Addy et al. Citation2012; Shi et al. Citation2013; Sun, Sun, and Xu Citation2013).
Serpentine is a 1:1 layered hydrated magnesium silicate mineral, the unit layer structure of which is composed of the six-square mesh (T-layer) of the silicon-oxygen tetrahedron with the eight-surface layer (O-layer) of magnesium hydroxide. There are unsaturated Si-O-Si, O-Si-O, magnesium bonds and hydroxyl groups on the fracture surface of serpentine, which constitutes the active group of serpentine (Li et al. Citation2003). Serpentine has high chemical activity due to the presence of active groups. Furthermore, serpentine has outstanding resource advantages with the proved reserves over 500 million tons in China (Yang, Zhang, and Zhao Citation2010). At present, only a small amount of serpentine was used to produce calcium magnesium phosphate, magnesium oxide and porous silicon oxide, and our previous study found the thermal-activated serpentine obtained by heating natural serpentine exhibited good adsorption performance to Cd due to increase of surface area and the pH of solution induced by MgO hydrolysis with increase MgO content of thermal-activated serpentine (Cao et al. Citation2017). However, it was rarely reported that the remediation of heavy metal contaminated soil using serpentine as amendment material. Therefore, it is of great theoretical and practical significance to study the effect of serpentine on Cd forms in soil and its mechanism to remediation heavy metal contaminated soil.
In this paper, the nature serpentine was used as low-cost amendment material for the remediation of Cd polluted farmland soil. In order to improve effects of immobilization Cd, the serpentine was modified by thermal activation. The performance of immobilization Cd via using natural and thermal-activated serpentine was systematically investigated by soil incubation experiments with the methods of sequential extraction and toxicity characteristic leaching procedure (TCLP).
2. Materials and methods
2.1. Experimental soils and materials
The agricultural soil samples were collected at a depth of 0–20 cm from a farmland of Jianping County, Chaoyang City, China. The basic physical and chemical characteristics of tested soil analyzed according to Bao (Citation2000) are listed in .
Table 1. Physical and chemical properties of the tested soil.
The Cd-polluted soil was prepared by air-dried soil mixed with CdCl2 solution, and the content of Cd in soil was 8 mg·kg−1, and the soil was incubated for 45 days. The deionized water was added to soil samples every day to maintain 60% field water-holding capacity during the incubation.
The serpentine was obtained from Xiuyan County, Anshan City, Liaoning Province, China. The composition and content of serpentine were determined by Philips MagiX X-ray fluorescence spectrometer and shown in . The serpentine includes Mg, Si, Al, Ca, Fe without other heavy metal such as Cd, Ni, Cu, and Cr, therefore, the application of serpentine cannot introduce other heavy metal. The thermal-activated serpentine was prepared according to the reference (Cao et al. Citation2017), i.e., the serpentine was heated to a given temperature in muffle furnace for 2 h by increasing the temperature at a heating rate of 2°C min−1 to obtain the thermal-activated serpentine, and abbreviated as ST, where T was the activation temperature. The natural serpentine was abbreviated as S0.
Table 2. The composition and content of natural serpentine.
2.2. Experimental designs
The natural and thermal-activated serpentine were blended into the Cd-polluted soil at the rate of 0% (abbreviated as CK), 1%, 3%, and 5% (abbreviated as ST-1, ST-3, and ST-5, respectively), and then it (100 g) was placed into plastic pot, incubated at a constant temperature of 25oC for 15, 30, 60 days, respectively. Loss of water was made up using deionized water to reach 60% of the field water-holding capacity and maintained this humidity by daily watering throughout the cultivation. Each treatment was performed in triplicate. Finally, the pH of soil, the content of TCLP extractable Cd and different Cd forms were measured.
2.3. Sample analysis
The pH was measured at a soil: water ratio of 1:2.5 (m/m) using a pH meter. Soil samples were digested using a HNO3-HF-HClO4 (2:2:1, v/v/v) solution at a 1:25 soil/liquid ratio to determine the total Cd concentration. TCLP was conducted to measure the extractable-Cd concentration (Peters Citation1999), i.e., the soil (1 g) was added into extraction solvent (20 g glacial acetic acid solution with pH 2.88 ± 0.05), and then oscillated at room temperature for18 ± 2 h. Cd concentration in the soil extraction solution was detected using atomic absorption spectrometry (AAS) (WYS2200, Wanyi, Anhui). Changes in the form of Cd in soil were analyzed by sequential extraction of Tessier (Tessier, Campbell, and Bisson Citation1979), which separated heavy metals into five different fractions: extractable with 1.0 M MgCl2 (pH = 7) (exchangeable form), extractable with 1.0 M NaOAc (pH = 5) (carbonate form), extractable with 0.04 M NH2OH·HCl in 25% (vol.%) HOAc solution (Fe-Mn oxide form), extractable with 0.02 M HNO3, 30% (wt.%) H2O2 and 3.2 M NH4OAC in 20% (vol.%) HNO3 solution (organic form), and HNO3-HF-HClO4 digested (residual form).
The data were analyzed using the SPSS 19.0 statistical package. Differences in Cd concentrations measured throughout the experiments were compared between treatment groups or within groups with ANOVA using the Tukey test at the 0.05 significance level. Pearson correlation coefficients were calculated in order to determine the relationships between soil pH and the content of TCLP-Cd at the 0.01 significance level.
3. Results and discussion
3.1. Effect of adding serpentine on soil pH
Soil pH can affect the solution and surface complexation reactions of cations, ion exchange, and other metal-binding processes, and then playing a significant role in determining the mobility and bioavailability of heavy metals in soil. The effects of adding different serpentines on soil pH are shown in .
Table 3. Changes of pH in Cd contaminated soil with different serpentine treatments and results of ANOVA.
Compared to CK, the soil pH is increased significantly after adding different serpentines, the content of which is strongly dependent on the thermal activation temperature of serpentine, the serpentine dosage, and the soil incubation time. The soil pH exhibits an increasing and decreasing tendency with the increase of serpentine dosage and incubation time, respectively. The increase of soil pH is the most obvious after adding S700-5 for incubating 15 days. Recently, we investigated the adsorption of cadmium with thermal-activated serpentine, and found the pH of solution after adsorption was increased in different degrees due to hydrolysis of MgO in serpentine (Cao et al. Citation2017). In the soil environment, hydrolysis of MgO in serpentine would still take place, then leading to the increase of soil pH. In the case of incubating 15 days, the soil treated by S700-5 exhibits the maximum pH. If taking the hydrolysis capacity of different serpentines in solution into account, (Cao et al. Citation2017), it will be understood that the soil pH is increased to different degree when adding different serpentines. In addition, the soil is a natural buffer system, therefore, the soil pH exhibits slight decline with the increase of the incubation time.
3.2. Effect of adding serpentine on the content of TCLP-Cd in soil
presents the content changes of TCLP-Cd in soil via different treatments. Compared with CK, the contents of TCLP-Cd in soil decreases significantly after adding different serpentines, the amount of which is dependent on the activation temperature of serpentine, moreover, the decreasing tendency becomes prominent as the incubation time and the dosage of natural and thermal-activated serpentine increase. The S700 additions reduce TCLP-Cd by 33.18 ~ 47.77% compared to the control soil, which is higher than results reported by previous studies, for example, natural calcium-rich attapulgite addition reduced TCLP-Cd by 42.8% (Yin and Zhu Citation2016), modified diatomite treatments addition reduced TCLP-Cd by 33.1% (Ye et al. Citation2015), palygorskite addition reduced TCLP-Cd by 11~32% and sepiolite addition reduced TCLP-Cd by 17~28% compared to the control soil. (Liang et al. Citation2014a).
Table 4. Effects of different serpentines on TCLP-Cd content in soil and results of ANOVA.
The unit layer structure of serpentine is composed of the six-square mesh (T-layer) of the silicon-oxygen tetrahedron with the eight-surface layer (O-layer) of magnesium hydroxide (Li et al. Citation2003). Cadmium ions can be combined with hydroxyl to enrichment on mineral surface and can also be adsorbed in the unit layer. Therefore, the content of TCLP-Cd in soil is significantly decreased after adding serpentine. The O-Si-O bond of serpentine would be broken during the process of thermal activation (Ghoorah et al. Citation2014; Cao et al. Citation2017), which leads to the increase of active adsorption point, meanwhile, the surface area of serpentine would be increased after thermal activation (Cao et al. Citation2017). If considering these, it can understand why the decreased content of TCLP-Cd is most obvious after adding thermal-activated serpentine compared with natural serpentine.
Furthermore, soil pH would play a significant role in determining the TCLP-extractable heavy metals concentrations in soil. The precipitates such as metal hydroxides, carbonates and metal-organic complexes were easier to be formed in the higher pH (Gu et al. Citation2011; Simmler et al. Citation2013; Liang et al. Citation2014a), moreover, the higher pH usually results in the generation of more negatively charged sorption sites. Therefore, the increase of pH would decrease the availability and mobility of metals in soil. In order to illuminate the relationship between soil pH and the content of TCLP-Cd in soil, the correlation between soil pH and the content of TCLP-Cd is analyzed (). The results show that the soil pH is significantly negatively correlated with the content of TCLP-Cd in different incubation time, which indicates that the soil pH is indeed an important factor affecting the content of TCLP-Cd. This is consistent with the findings of Jafarnejadi et al. (Citation2013).
Table 5. Regression analysis on TCLP-Cd in soil and soil pH in different periods.
3.3. Effect of adding serpentine on the chemical form of Cd in soil
The content change of exchangeable Cd in soil after adding different serpentines is shown in . The content of exchangeable Cd in soil after adding different serpentines is obviously lower than CK treatment. The content of exchangeable Cd is decreased gradually with the increase of incubation time and serpentine dosage. Under the same incubation time and serpentine dosage, the exchangeable Cd content in soil shows differences by adding different serpentines, and the content of exchangeable Cd in soil is dependent on the activation temperature of serpentine, and the amount of which is increased in the order of S700 < S550 < S850 < S350 < S0. After adding S700-5 for incubating 60 day, the content of exchangeable Cd in soil is 3.27 mg·kg−1, which is minimum among all serpentine treatments, reducing by 3.0 mg·kg−1 compared with CK treatment under the same condition.
Table 6. The changes of exchangeable form of Cd content in soil after the application of different serpentines and results of ANOVA.
It can be seen from that the content of carbonate form of Cd in soil is increased to some extent after adding different serpentines. In the case of the same serpentine, the content of carbonate form of Cd in soil is increased with serpentine dosage and incubation time. The increased content of carbonate form of Cd in soil by thermal-activated serpentine treatment is more obviously compared with natural serpentine. The content of carbonate form of Cd in soil is the highest when the soil is treated with S700-5 for 60 days, which exhibits significant differences compared with other treatment. It was well known that Cd2+ would easily form Cd(OH)2 under the condition of alkalinity, and then Cd(OH)2 would adsorb CO2 to form CdCO3 precipitates. In addition, the content of inorganic carbon in soil is increased with the pH value of soil (Zu et al. Citation2011). Therefore, the content of carbonate as inorganic carbon will also increase, which results in the increase of carbonate form of Cd due to Cd2+ reacting with CO32− to form CdCO3 precipitates. According to the Section 3.1, adding serpentine can raise the soil pH, then leading to the increase of carbonate form of Cd. Furthermore, the amount of carbonate form of Cd will vary due to varied soil pH after adding different serpentines ().
Table 7. The changes of carbonate form of Cd content in soil after the application of different serpentines and results of ANOVA.
As can be seen from , the content of Fe-Mn oxide form of Cd in soil treated by different serpentines is increased obviously compared with CK. In the case of adding the same serpentine, with the increase of serpentine dosage and incubation time, the content of Fe-Mn oxide form of Cd appears an increasing tendency. The content of Fe-Mn oxide form of Cd in soil treated by thermal-activated serpentine is dependent on the activation temperature, which is firstly increased and then decreased with the increase of the activation temperature. When the soil is treated with S700-5 for 60 days, the content of Fe-Mn oxide form of Cd in soil is the highest (1.43 mg·kg−1), which is increased by 0.69 mg·kg−1 compared to CK. Moreover, the exchangeable Cd content in soil treated with S700 exhibits significant differences compared with other treatment.
Table 8. The changes of Fe-Mn oxide form of Cd content in soil after the application of different serpentines and results of ANOVA.
As can be seen from , the content of organic form of Cd in the soil treated by different serpentines is obviously increased compared with CK treatment, and the increasing degree is related to the thermal activation temperature, incubation time and serpentine dosage. After adding S700-5 treating for 30 days, the content of organic form of Cd in the soil is maximum (0.112 mg·kg−1), which is increased by 67.3% compared with CK. This is because that the solubility of organic matter in soil will be increased with the pH value, which results in the increase of the complexation capacity of heavy metals (Curtin, Peterson, and Anderson Citation2016). Therefore, the content of organic form of Cd in soil is increased after adding the serpentine.
Table 9. The changes of organic form of Cd content in soil after the application of different serpentines and results of ANOVA.
provides the content change of residual form of Cd in soil after adding different serpentines. The content of residual form of Cd in soil treated by different serpentines is different, but it is much higher than that of CK treatment. In the same treatment condition, the content of residual form of Cd in soil is decreased in the order of S700 > S550 > S850 > S350 > S0. Moreover, the residual form of Cd content in soil treated with S700 exhibits significant differences compared with other treatment. And the residual form of Cd shows an increasing tendency with the increase of incubation time and serpentine dosage.
Table 10. The changes of residual form of Cd content in soil after the application of different serpentines and results of ANOVA.
The primary way for reducing the harm of heavy metals in soil is to convert the exchangeable form to other low bioavailable form, especially residual form, and then reducing the content of bioavailable form of the heavy metal. The effect of different serpentines on the distribution of various Cd forms in soil is shown in .
Figure 1. Distribution of various Cd forms after the application of different serpentines (incubation time 60 days).
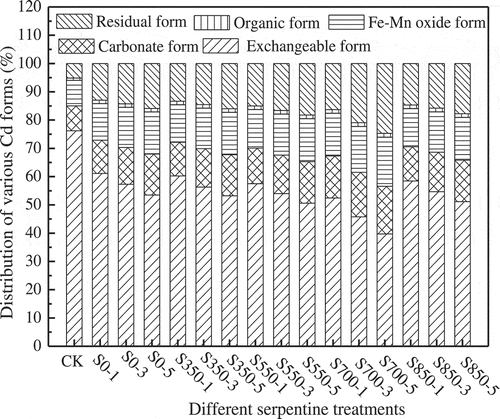
In the case of CK, Cd concentration is in the order of exchangeable form > Fe-Mn oxide form > carbonate form > residual form > organic form. However, the form of Cd is greatly changed due to adding the serpentine. The exchangeable Cd is obviously decreased, and the residual form, Fe-Mn oxide form and carbonate form are increased, especially residual form, the content of which is strongly dependent on the activation temperature and dosage of serpentine. And the change of organic form is not obvious. This demonstrate serpentine addition mainly converts the exchangeable Cd into carbonated, Fe-Mn oxide, and residual forms, and it illustrates that the serpentine (especially S700) addition can effectively reduce the bioavailable Cd speciation and convert it to a much less bioavailable speciation, which will help to account for the reduction in leachability and bioaccessibility. Under the same treatment condition, compared with CK treatment, adding S700 can reduce exchangeable Cd by 23.76~36.49%, increase carbonate, Fe-Mn oxide, organic form, and residual form of Cd by 6.03~8.03%, 6.05~8.35%, 0.49~0.54%, and 11.17~19.58%, respectively, and S700 exhibits a better immobilization effect.
3.4. Immobilization mechanism
In the immobilization remediation of heavy metal contaminated soil, it is generally believed that silicate minerals can reduce the bioavailability of heavy metals mainly by adsorption (Koptsik and Zakharenko Citation2014; Kumararaja et al. Citation2017). The natural serpentine is a layered silicate mineral with a larger specific surface area and a plurality of exchangeable cationic active points. Therefore, adding serpentine is beneficial to the chemical adsorption, physical adsorption, and ion exchange adsorption of heavy metal ions in soil, which results in reducing the content of bioavailable form of the heavy metal. When the natural serpentine is heated at different temperature, the specific surface area is further increased. In the case of serpentine activated by 700°C, the surface is increased to twice times due to the original layered structure collapsed to form slit-shaped pore-like structure (Cao et al. Citation2017), which further increases its adsorption capacity of heavy metal in soil. This is beneficial to reduction of extractable-Cd concentration, which is revealed by , i.e., the content of TCLP-Cd drops monotonously with the increase of BET surface area. In addition, the increase of pH induced by adding serpentine is conducive to the heavy metal affiliated with OH− and CO32− in soil, and then form hydroxide precipitation and carbonate precipitation of heavy metals, and then resulting in reduction of extractable-Cd concentration (). Therefore, adding serpentine can promote the transformation from exchangeable form of Cd in soil to low bioavailability of carbonate form, Fe-Mn oxides form and residual form by surface adsorption and surface precipitation, thus reducing the bioavailability of Cd in soil.
Table 11. Relationship between TCLP-Cd in soil and surface area of serpentine.
4. Conclusion
Adding serpentine could significantly increase soil pH, and the improvement of pH value was dependent on the thermal activation temperature of serpentine, dosage and the soil incubation time. After adding different serpentines, the contents of TCLP-Cd in soil was decreased significantly, especially, adding serpentine activated at 700°C. Through association analysis, it could be found that the soil pH was significantly negatively correlated with the content of TCLP-Cd. The different serpentines exhibited different immobilization capabilities for Cd-contaminated soils. The serpentine (especially S700) could effectively reduce the bioavailable Cd speciation (exchangeable form) and convert it to a much less bioavailable speciation (Fe-Mn oxide and residual form), which helped to account for the reduction in leachability and bioaccessibility.
Disclosure statement
No potential conflict of interest was reported by the authors.
Additional information
Funding
References
- Addy, M., B. Losey, R. Mohseni, E. Zlotnikov, and A. Vasiliev. 2012. “Adsorption of Heavy Metal Ions on Mesoporous Silica-modified Montmorillonite Containing a Grafted Chelate Ligand.” Applied Clay Science 59-60: 115–120. doi:10.1016/j.clay.2012.02.012.
- Bao, S. D. 2000. Soil and Agricultural Chemistry Analysis. Beijing: China Agricultural Press.
- Basta, N. T., R. Gradwohl, K. L. Snethen, and J. L. Schroder. 2001. “Chemical Immobilization of Lead, Zinc, and Cadmium in Smelter-contaminated Soils Using Biosolids and Rock Phosphate.” Journal of Environmental Quality 30 (4): 1222–1230. doi:10.2134/jeq2001.3041222x.
- Cao, C.-Y., C.-H. Liang, Y. Yin, and L.-Y. Du. 2017. “Thermal Activation of Serpentine for Adsorption of Cadmium.” Journal of Hazardous Materials 329: 222–229. doi:10.1016/j.jhazmat.2017.01.042.
- Chang, Y.-T., H.-C. Hsi, Z.-Y. Hseu, and S.-L. Jheng. 2013. “Chemical Stabilization of Cadmium in Acidic Soil Using Alkaline Agronomic and Industrial By-products.” Journal of Environmental Science and Health, Part A Toxic/Hazardous Substances and Environmental Engineering 48 (13): 1748–1756. 815571. doi:10.1080/10934529.2013.
- Curtin, D., M. E. Peterson, and C. R. Anderson. 2016. “pH-dependence of Organic Matter Solubility: Base Type Effects on Dissolved Organic C, N, P, and S in Soils with Contrasting Mineralogy.” Geoderma 271: 161–172. doi:10.1016/j.geoderma.2016.02.009.
- Diels, L., N. van der Lelie, and L. Bastiaens. 2002. “New Developments in Treatment of Heavy Metal Contaminated Soils.” Reviews in Environmental Science and Biotechnology 1: 75–82. doi:10.1023/A:1015188708612.
- Ghoorah, M., B. Z. Dlugogorski, H. C. Oskierski, and E. M. Kennedy. 2014. “Study of Thermally Conditioned and Weak Acid-treated Serpentinites for Mineralization of Carbon Dioxide.” Minerals Engineering 59: 17–30. doi:10.1016/j.mineng.2014.02.005.
- Gu, -H.-H., H. Qiu, T. Tian, -S.-S. Zhan, T.-H.-B. Deng, R. L. Chaney, S.-Z. Wang, Y.-T. Tang, J.-L. Morel, and R.-L. Qiu. 2011. “Mitigation Effects of Silicon Rich Amendments on Heavy Metal Accumulation in Rice (Oryza Sativa L.) Planted on Multi-metal Contaminated Acidic Soil.” Chemosphere 83 (9): 1234–1240. doi:10.1016/j.chemosphere.2011.03.014.
- Hang, X., J. Zhou, H. Wang, and P. Shen. 2007. “Remediation of Heavy Metal Contaminated Soils Using Clay Minerals.” Chinese Journal of Environmental Engineering 1 (9): 113–120.
- Hashem, A., E. Adam, H. A. Hussein, M. A. Sanousy, and A. Ayoub. 2013. “Bioadsorption of Cd (II) from Contaminated Water on Treated Sawdust: Adsorption Mechanism and Optimization.” Journal of Water Resource and Protection 5: 82–90. doi:10.4236/jwarp.2013.51010.
- Jafarnejadi, A. R., G. Sayyad, M. Homaee, and A. H. Davamei. 2013. “Spatial Variability of Soil Total and DTPA-extractable Cadmium Caused by Long-term Application of Phosphate Fertilizers, Crop Rotation, and Soil Characteristics.” Environmental Monitoring and Assessment 185: 4087–4096. doi:10.1007/s10661-012-2851-2.
- Khan, M. A., S. Khan, A. Khan, and M. Alam. 2017. “Soil Contamination with Cadmium, Consequences and Remediation Using Organic Amendments.” Science of the Total Environment 601-602: 1591–1605. doi:10.1016/j.scitotenv.2017.06.030.
- Komárek, M., A. Vaněk, and V. Ettler. 2013. “Chemical Stabilization of Metals and Arsenic in Contaminated Soils Using Oxides - A Review.” Environmental Pollution 172: 9–22. doi:10.1016/j.envpol.2012.07.045.
- Koptsik, G. N., and A. I. Zakharenko. 2014. “The Effects of Different Amendments on the Mobility and Toxicity of Nickel and Copper in Polluted Soils.” Moscow University Soil Science Bulletin 69: 29–34. doi:10.3103/S0147687414010062.
- Kumararaja, P., K. M. Manjaiah, S. C. Datta, and B. Sarkar. 2017. “Remediation of Metal Contaminated Soil by Aluminium Pillared Bentonite: Synthesis, Characterisation, Equilibrium Study and Plant Growth Experiment.” Applied Clay Science 137: 115–122. doi:10.1016/j.clay.2016.12.017.
- Kumpiene, J., A. Lagerkvist, and C. Maurice. 2008. “Stabilization of As, Cr, Cu, Pb and Zn in Soil Using Amendments - A Review.” Waste Management 28 (1): 215–225. doi:10.1016/j.wasman.2006.12.012.
- Lee, S. H., J. S. Lee, Y. J. Choi, and J. G. Kim. 2009. “In Situ Stabilization of Cadmium-, Lead-, and Zinc-Contaminated Soil Using Various Amendments.” Chemosphere 77 (8): 1069–1075. doi:10.1016/j.chemosphere.2009.08.056.
- Li, J., and Y. Xu. 2015. “Immobilization of Cd in a Paddy Soil Using Moisture Management and Amendment.” Chemosphere 122: 131–136. doi:10.1016/j.chemosphere.2014.11.026.
- Li, X.-J., L.-J. Wang, A.-H. Lu, and C.-Q. Wang. 2003. “A Discussion on Activation Mechanism of Atom Groups in Serpentine.” Acta Petrologica Et Mineralogica 22 (4): 386–390. doi:10.1016/S0955-2219(02)00073-0.
- Liang, X., J. Han, Y. Xu, Y. Sun, L. Wang, and X. Tan. 2014a. “In Situ Field-scale Remediation of Cd Polluted Paddy Soil Using Sepiolite and Palygorskite.” Geoderma 235-236: 9–18. doi:10.1016/j.geoderma.2014.06.029.
- Liang, X., J. Han, Y. Xu, L. Wang, Y. Sun, and X. Tan. 2014b. “Sorption of Cd2+ on Mercapto and Amino Functionalized Palygorskite.” Applied Surface Science 322: 194–201. doi:10.1016/j.apsusc.2014.10.092.
- Liang, X., Y. Xu, L. Wang, G. Sun, X. Qin, and Y. Sun. 2011. “In-situ Immobilization of Cadmium and Lead in a Contaminated Agricultural Fields by Adding Natural Clays Combined with Phosphate Fertilizer.” Acta Scientiae Circumstantiae 31 (5): 1011–1018. doi:10.3724/SP.J.1011.2011.00468.
- Liu, L., W. Li, W. Song, and M. Guo. 2018. “Remediation Techniques for Heavy Metal-contaminated Coils: Principles and Applicability.” Science of the Total Environment 633: 206–219. doi:10.1016/j.scitotenv.2018.03.161.
- Peters, R. W. 1999. “Chelant Extraction of Heavy Metals from Contaminated Soils.” Journal of Hazardous Materials 66: 151–210. doi:10.1016/S0304-3894(99)00010-2.
- Shi, W.-Y., H. Li, S. Du, K.-B. Wang, and H.-B. Shao. 2013. “Immobilization of Lead by Application of Zeolite: Leaching Column and Rhizobox Incubation Studies.” Applied Clay Science 85: 103–108. doi:10.1016/j.clay.2013.08.022.
- Simmler, M., L. Ciadamidaro, R. Schulin, P. Madejon, R. Reiser, L. Clucas, P. Weber, and B. Robinson. 2013. “Lignite Reduces the Solubility and Plant Uptake of Cadmium in Pasturelands.” Environmental Science & Technology 47 (9): 4497–4504. doi:10.1021/es303118a.
- Sun, Y., G. Sun, and Y. Xu. 2013. “Assessment of Sepiolite for Immobilization of Cadmium-contaminated Soils.” Geoderma 193-194: 149–155. doi:10.1016/j.geoderma.2012.07.012.
- Sun, Y., G. Sun, Y. Xu, L. Wang, D. Lin, X. Liang, and X. Shi. 2012. “In Situ Stabilization Remediation of Cadmium Contaminated Soils of Wastewater Irrigation Region Using Sepiolite.” Journal of Environmental Sciences 24 (10): 1799–1805. doi:10.1016/S1001-0742(11)61010-3.
- Sun, Y., Y. Xu, Y. Xu, L. Wang, X. Liang, and Y. Li. 2016. “Reliability and Stability of Immobilization Remediation of Cd Polluted Soils Using Sepiolite under Pot and Field Trials.” Environmental Pollution 208: 739–746. doi:10.1016/j.envpol.2015.10.054.
- Tessier, A., P. G. C. Campbell, and M. Bisson. 1979. “Sequential Extraction Procedure for the Speciation of Particulate Trace Metals.” Analytical Chemistry 51 (7): 844–850. doi:10.1021/ac50043a017.
- Wang, Y., X. Tang, Y. Chen, L. Zhan, Z. Li, and Q. Tang. 2009. “Adsorption Behavior and Mechanism of Cd(II) on Loess Soil from China.” Journal of Hazardous Materials 172: 30–37. doi:10.1016/j.jhazmat.2009.06.121.
- Yang, B., Z. Zhang, and F. Zhao. 2010. “Current Situation and Tendency of Comprehensive Utilization of Serpentine.” Materials Review 24: 381–384.
- Ye, X., S. Kang, H. Wang, H. Li, Y. Zhang, G. Wang, and H. Zhao. 2015. “Modified Natural Diatomite and Its Enhanced Immobilization of Lead, Copper and Cadmium in Simulated Contaminated Soils.” Journal of Hazardous Materials 289: 210–218. doi:10.1016/j.jhazmat.2015.02.052.
- Yin, H., and J. Zhu. 2016. “In Situ Remediation of Metal Contaminated Lake Sediment Using Naturally Occurring, Calcium-rich Clay Mineral-based Low-cost Amendment.” Chemical Engineering Journal 285: 112–120. doi:10.1016/j.cej.2015.09.108.
- Zu, Y., R. Li, W. Wang, D. Su, Y. Wang, and L. Qiu. 2011. “Soil Organic and Inorganic Carbon Contents in Relation to Soil Physicochemical Properties in Northeastern China.” Acta Ecologica Sinica 31 (18): 5207–5216.