ABSTRACT
Fertilizer-derived P is accumulated in cropland soils and their phytoavailability is variable depending on the speciation of P, which is affected by soil properties. In this study, we aimed to clarify how soil properties affect the speciation of P accumulated in fertilized soils. Soil samples were collected from the cultivated soil layers of 137 vegetable fields in Aichi prefecture, Japan. The speciation of soil P was evaluated by Truog method, sequential extraction by acetic acid, NH4F, and NaOH (Sekiya method), P K-edge X-ray adsorption near-edge structure (XANES). Phosphorus accumulated in cropland consisted predominantly of the inorganic form. Phosphorus extracted by the Truog method and acetic acid was correlated with exchangeable Ca and total C. The XANES analysis indicated that the P species extracted by Truog and acetic acid were associated with Ca, while those extracted by acetic acid also included crystalized calcium phosphate with higher crystallinity than CaHPO4, for example, hydroxyapatite. Due to the high application of P fertilizer and manure with lime, P was accumulated as calcium phosphate except for soils with relatively high contents of oxalate extractable Al and Fe. The amounts of NH4F-extracted P were higher in soils with larger amounts of oxalate extractable Al and Fe. Based on the XANES analyses, the P species extracted by NH4F were associated with Al and Fe, which is inconsistent with the operational definition of NH4F-soluble species. The P in soils extracted by NaOH was correlated with various soil properties, especially with clay content. The P species extracted by NaOH could not be identified. Our results revealed that the important factors controlling available P associated with Ca were total C and exchangeable Ca, whereas those controlling the P extracted by NH4F were oxalate extractable Al and Fe, because this fraction of P was associated with Al and Fe.
1. Introduction
A significant amount of phosphorus (P) accumulates in Japanese arable land, especially in vegetable fields, due to the application of P fertilizer and manure exceeding the amounts of P uptake by crops (Ohara and Nakai Citation2004; Mishima et al. Citation2013). The accumulated P is detrimental to water quality, flowing into water sources via surface and subsurface pathways. For the sustainable management of P in cropland, especially excessively fertilized cropland, P fertilizer application should be reduced by considering the accumulated amounts of P in soils.
Applied P in excess of crop requirements is associated with Ca-carbonate, Al- and Fe-(hydro) oxides, silicate clay minerals, and humic substances (Beauchemin et al. Citation2003; Gérard Citation2016). These P species accumulated in soils are affected by soil physicochemical properties, such as soil pH and the amount of Al and Fe extracted by oxalate, exchangeable Ca, and total carbon (Wright Citation2009; Nishigaki et al. Citation2018; Nakamura et al. Citation2019).
The chemical extraction method is commonly used to evaluate the amount of different forms of P in soils based on their solubility in the extractants. Truog method is generally used for the evaluation of phytoavailable P in Japanese agricultural soils (Mishima et al. Citation2013). Sequential fractionation methods, such as the Hedley method (Hedley, Stewart, and Chauhan Citation1982) and Sekiya method (Sekiya Citation1983), are also used to determine the amounts of P with different mobility and stability. In particular, the Sekiya method, which is modified after the CJ method (Chang and Jackson Citation1957) to adapt to the Andosols and acidic soils, is extensively used for Japanese soils (Otani and Ae Citation1999; Tani et al. Citation2010; Nakamura et al. Citation2019). The Sekiya method defines calcium phosphate as labile P and P associated with Al minerals and Fe minerals as relatively stable P and stable P, respectively. Nakamura et al. (Citation2019) showed that labile P and relatively stable P were controlled by exchangeable Ca and Al oxides in upland sandy soil, respectively, while Tani et al. (Citation2010) showed that relatively stable P was controlled by Al and Fe oxides in Andosols. However, the relationships of P fractions with soil properties were not intensively investigated especially for lowland and clayey soils in cropland.
Since the chemical extraction method operationally fractionates P species based on the solubility in the extractants, it is difficult to specify the chemical forms of P in soils. Characterization of the predominant P species has been achieved by molecular spectroscopic techniques, particularly P K-edge X-ray adsorption near-edge structure (XANES) and 31P nuclear magnetic resonance (NMR) spectroscopy. Phosphorus K-edge XANES can discriminate inorganic P species based on the clearly different spectral features among Ca-, Fe- and Al-bonded phosphate (Beauchemin et al. Citation2003). Solution 31P-NMR has the advantage of identifying the organic P species by referring to the chemical shifts of the known references (Cade-Menun and Liu Citation2014). Recently, many studies have complementary applied chemical extraction methods, P K-edge XANES and solution 31P-NMR spectroscopy to investigate the effects of the excessive application of P fertilizer on the species and the amount of P in long-term fertilized fields (Sato et al. Citation2005; Abdala et al. Citation2015; Eriksson, Gustafsson, and Hesterberg Citation2015; Liu et al. Citation2015; Luo et al. Citation2017; Koch et al. Citation2018). Such complementary studies of chemical extraction and spectroscopy should be effective to clarify the characteristics of P accumulated in soils with different properties.
Our objectives were to elucidate the amount and the species of P accumulated in excessively fertilized cropland soils in Aichi prefecture, Japan, by focusing on the relationships with soil properties. We also aimed to evaluate P species extracted by the Truog method and sequential extraction method (Sekiya method), which is widely applied for the analyses of P in Japanese soils, by comparing those data with the speciation results obtained from P K-edge XANES.
2. Materials and methods
2.1. Soil samples
Soil samples were collected from the cultivated soil layer (soil depth from 0 cm to approximately 15 cm) from 137 agricultural vegetable fields in Aichi prefecture, central Japan (). The soils investigated were classified into eight soil types (Cultivated soil classification committee Citation1995). The lowland soils, which comprise 35% of the cropland in Aichi prefecture, included three soil groups: Brown Lowland soils (BL), Regosolic Lowland soils (RL) and Gray Lowland soils (GL). The BL group was further divided into two groups according to the presence of the humic horizon: BLn, distributed on the west side, and BLh, with a thick humic horizon, distributed on the east side (). Brown Forest soils (BF) compose 5.1% of the cropland in Aichi prefecture and are distributed in the western peninsula. Yellow soils are distributed in 26% of the cropping area, mainly in the eastern peninsula and central part. They were further divided into three groups based on soil texture: coarse (Yc), medium (Ym) and fine (Yf) texture, according to clay contents of < 15%, 15–25% and > 25%, respectively (Cultivated soil classification committee Citation1995). The number of samples investigated in this study is shown in (), according to soil groups. Soil samples were air dried and sieved to < 2 mm. A portion of each sample was finely ground (<0.5 mm) for the analysis of P speciation by chemical extraction and P K-edge XANES spectroscopy.
Table 1. Number of samples analyzed for each soil group
Figure 1. Map of sampling locations. Sample names on the map indicate the samples analyzed by P K-edge XANES. The distribution of Non-allophanic Andosols was revised based on Kato (Citation1970) and Matsuyama et al. (Citation1994)
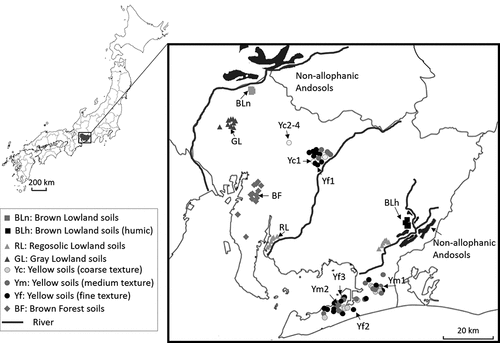
2.2. Physicochemical properties of the soils
Soil pH was measured using a soil-to-solution (H2O) ratio of 1:5 (w/v). Exchangeable Ca was extracted with 1 M ammonium acetate at pH 7. The soil particle size distribution was determined by wet-sieving and the pipet method (Gee and Bauder Citation1986). Extractable Al and Fe were determined by dithionite-citrate-bicarbonate (Fed and Ald) and by 0.2 M ammonium oxalate at pH 3.0 in the dark (Alo and Feo) as described by Courchesne and Turmel (Citation2008). Total carbon (C) was determined by the dry combustion method (JM1000, J-Science Lab CO., Ltd., Kyoto).
2.3. Phosphorus speciation by chemical extraction
Phosphorus fractionation was carried out by the sequential extraction method proposed by Sekiya (Citation1983), which is a modification of the CJ method (Chang and Jackson Citation1957) to adapt it to Japanese acidic soils. Soil (0.5 g) was extracted with 50 mL of 0.44 M (2.5% v/v) acetic acid for 2 h, and the solution phase was filtered after centrifugation. The residual soil was washed twice with 25 mL of 1 M NH4Cl, which was filtered and added to the acetic acid extract. Distilled water was added to the extract to make a total of 100 mL. This first extract (AA-P) was considered to mainly include phosphate associated with Ca. The soil P was subsequently extracted with 50 mL of 1 M NH4F at pH 7.0 for 1 h, and the supernatant (NH4F-P) was collected after centrifugation. Before extracting the subsequent fraction, the sedimented soil was washed twice with 25 mL of saturated NaCl solution, and then the NaCl solution was discarded. The third fraction of P was extracted with 50 mL of 0.1 M NaOH for 17 h, and then the supernatant (NaOH-P) was collected after centrifugation. The sequentially fractionated P designated as NH4F-P and NaOH-P were operationally defined as P associated with the Al-bearing phase and Fe-bearing phase, respectively (Sekiya Citation1983). In addition to the sequential extraction, available P was extracted according to the method proposed by Truog (Citation1930). Soil (0.5 g) was extracted with 100 mL of 0.001 M H2SO4 for 30 min. The phosphate concentration in each extract was determined by the molybdenum blue method (Murphy and Riley Citation1962). Total P in the soil was extracted in 0.5 M H2SO4 after ignition of soil for 1 h at 550°C (Komiyama et al. Citation2009), and the phosphate concentration in the extract was determined by the vanadomolybdate method (Kitson and Mellon Citation1944). The total P of 20 samples was also measured after digestion of soil with concentrated HNO3-HClO4 (Komiyama et al. Citation2009). We confirmed that the results obtained by the ignition method agreed well with those obtained by the digestion method (total P (digestion) = 0.992 * total P (ignition), r2 = 0.997). Soils before ignition were extracted in 0.5 M H2SO4 and organic P was calculated by the subtraction of the P contents extracted by 0.5 M H2SO4 before ignition from that after ignition (Komiyama et al. Citation2009).
2.4. Phosphorus K-edge XANES spectroscopy
Solid-state speciation by XANES was conducted for the 14 samples. One sample was selected from each soil group, but 2, 3 or 4 samples were selected from the Yellow soil group due to the large variation in their soil chemical properties. As standard materials, CaHPO4 (CaHPO4 · 2H2O, FUJFILM Wako Pure Chemical Corporation, Osaka) and hydroxyapatite (Ca10(PO4)6(OH)2, FUJFILM Wako Pure Chemical Corporation, Osaka), AlPO4 (Merck KGaA, Darmstadt), and FePO4 (Merck KGaA, Darmstadt) were used for phosphate associated with Ca, Al and Fe, respectively. In addition, phosphate adsorbed on gibbsite (synthesized after Kyle, Posner, and Quirk Citation1975), ferrihydrite (synthesized after Schwertmann and Cornell Citation1991) and kaolinite (JCSS-1101c, The Clay Science Society of Japan, Tsukuba) were prepared as standard materials by mixing sodium phosphate solution with the minerals at pH 6 for 24 h, and the phosphate-adsorbed minerals were freeze-dried after washing with water. Organic P materials were not used as standard materials because they were difficult to characterize by XANES due to the lack of distinctive spectral features (Beauchemin et al. Citation2003; Luo et al. Citation2017). Standard materials and soil samples with P concentrations above 300 mmol kg−1 were diluted using boron nitride to prevent self-absorption effects (Hurtarte et al. Citation2019).
Phosphorus K-edge XANES experiments were conducted at the tender X-ray beamline in the Aichi Synchrotron Radiation Center, BL6N1 equipped with an InSb (111) monochromator at ambient temperature under a He atmosphere. The electron beam energy was 2.5 GeV at the maximum beam current of 300 mA. The XANES data were collected in fluorescence mode by a silicon drift detector (Vortex-EM, Hitachi, Illinois) from 2120 to 2180 eV. The energy was adjusted against the first derivative maximum of the AlPO4 reference at 2151.4 eV (E0). The Athena ver. 0.9.26 program (Ravel and Newville Citation2005) was used to subtract the pre-edge background and normalize the spectra with respect to absorption at post-edge energy and linear combination fitting (LCF). In the LCFs, the fraction of each standard compound was set as an adjustable parameter to determine relative proportion of P species in soils, and optimization was achieved by minimizing the residual of the fit, defined as the normalized root-square difference between the data and the fit (R).
2.5. Statistical analysis
All statistical analyses were performed using Statcel2 Software (OMS Publishing, Saitama). All data were expressed on a dry-weight basis. The chi-square goodness-of-fit test was used to check whether data were normally distributed. Multiple comparisons were conducted using Bonferroni/Dunn’s test, and p < 0.05 indicated statistical significance. Spearman’s rank correlation analysis was used to detect the relationship between soil properties and the amount of soil P with specific chemical form evaluated by chemical extraction. The relationship between the contribution of P species to total P determined by the LCF of XANES data and the proportion of each soil P fraction to total P evaluated by chemical extraction was confirmed by single regression analysis.
3. Results
3.1. Soil properties
The soil physicochemical properties that potentially influence P speciation in soils are shown in (). Large variations across the soil groups were observed for pH (4.59–8.02), total C (2.3–64.2 g kg−1) and exchangeable Ca (11–235 mmolc kg−1). The large variations were likely attributed to the different application rate of lime and manure. Among the eight soil groups, BLh with humic horizon exhibited the highest amounts of total C, exchangeable Ca, Alo, Feo, and Ald. The Alo+1/2 Feo content was higher in the order of BLh, BLn, and GL and the lowest in BF. The clay content was high in Yf, Ym, BF, and BLh while low in RL and BLn.
Table 2. Physicochemical properties of soil samples for each soil group
3.2. Available P, sequentially extracted P fractions and total P
() shows total P, available P, and sequentially fractionated P (AA-P, NH4F-P and NaOH-P) as average values of each soil group. The amounts of total P for most of the soils were within the range from 641 to 4,483 mg P kg−1. However, the total P of the three soils characterized by high total C and exchangeable Ca exceeded 7,000 mg P kg−1: 7,900 mg P kg−1 for BLh, 8,324 mg P kg−1 for Ym, and 11,776 mg P kg−1 for Yf.
Table 3. Average value of P stock in soils evaluated by chemical extraction (mg P kg−1)
The available P extracted by the Truog method (Truog-P) ranged from 120 to 3,586 mg P kg−1, and 97% of samples exceeded the criterion of proper P level for vegetable production (218 mg P kg−1 (50 mg P2O5 100 g−1); Aichi department of agriculture, forestry and fisheries Citation2016). The amounts of AA-P ranged from 164 to 8,311 mg P kg−1. The amounts of AA-P were higher than Truog-P in the soil with high total P contents such as Ym and Yf, in accordance with previous studies (Tani et al. Citation2010; Nakamura et al. Citation2019). By excluding Ym and Yf data having especially high P contents, Truog-P and AA-P were lineally correlated (AA-P = 1.068 * Truog-P, r 2 = 0.802, p < 0.001). Truog-P of GL was significantly higher than those of BLn, RL, Yc and Yf (). The amounts of P extracted by NH4F (NH4F-P) ranged from 118 to 4,870 mg P kg−1 and were the highest in BLh, followed by BLn and GL. The amounts of P extracted by NaOH (NaOH-P) ranged from 29 to 807 mg P kg−1 and were the highest in BLh, followed by Yf, and the lowest in RL (). The ratio of Truog-P and AA-P to total P of almost all soil groups was higher than that of NH4F-P and NaOH-P, except for BLh, BLn and Yf (). A high ratio of NH4F-P was observed in BLh and BLn and the ratio of NaOH-P to total P was relatively high in Yf (). The ratio of NaOH-P was less than 15% in 110 out of 137 soil samples.
Correlation coefficients between each fraction of P and selected soil properties are shown in (). Truog-P and AA-P had strong correlations with exchangeable Ca and total C. Significant correlations with NH4F-P were observed for Alo, Feo and Alo +1/2 Feo. NaOH-P was significantly correlated with Ald, Fed and clay contents, while NaOH-P was correlated with almost all the soil properties listed in (), except for soil pH.
Table 4. Correlation coefficients between the amounts of P determined by chemical extraction and selected soil properties
3.3. Organic phosphorus
Organic P evaluated by chemical extraction for most of the soils ranged from 0 to 432 mg P kg−1, and the ratio of organic P to total P was less than 10% (). Organic P was relatively high in BLh and BLn among the eight soil groups, ranging from 85 to 897 mg P kg−1. The ratio of organic P in BLh and BLn ranged from 4 to 14%. Solution 31P-NMR revealed that P extracted by NaOH-EDTA for all 14 soil samples was dominated by orthophosphate, with a sharp peak at 6 ppm (Fig. S1). Peaks corresponding to orthophosphate monoesters (myo-inositol phosphate) between 3.0 and 5.8 ppm were detected in BLh, BLn, RL, GL and Yf2 (Fig. S1).
3.4. Phosphorus K-edge XANES spectroscopy
() shows the normalized XANES spectra of the standard materials. Based on the representative features, the standard materials could be categorized into three types: P associated with Ca (Ca-P), Al (Al-P) and Fe (Fe-P) (). The Ca-P standards, CaHPO4 and hydroxyapatite, exhibited a well-defined shoulder on the high-energy side of white-line peak (an intense absorption at the near-edge region). The shoulder peak was larger and expanded at a higher position in hydroxyapatite than in CaHPO4. This is because the shoulder peak becomes larger with increasing the abundance of Ca in the mineral structure (Ingall et al. Citation2011), decreasing solubility and increasing thermodynamic stability (Sato et al. Citation2005). The Fe-P standards, FePO4 and P adsorbed on ferrihydrite, exhibited a pre-edge peak between 2146 and 2149 eV (), and the pre-edge peak of FePO4 was higher than that of P adsorbed on ferrihydrite, which increases in intensity with increasing mineral crystallinity (Beauchemin et al. Citation2003). The XANES spectra of the Al-P standards were characterized by intense white-line peaks, and the peak height was higher for P adsorbed on kaolinite and gibbsite than for AlPO4. Organic P was difficult to identify by XANES due to the lack of distinctive spectral features (Beauchemin et al. Citation2003; Luo et al. Citation2017).
Figure 2. Phosphorus K-edge XANES spectra for standard materials. Ca-P, Al-P and Fe-P indicate P associated with Ca, Al and Fe, respectively
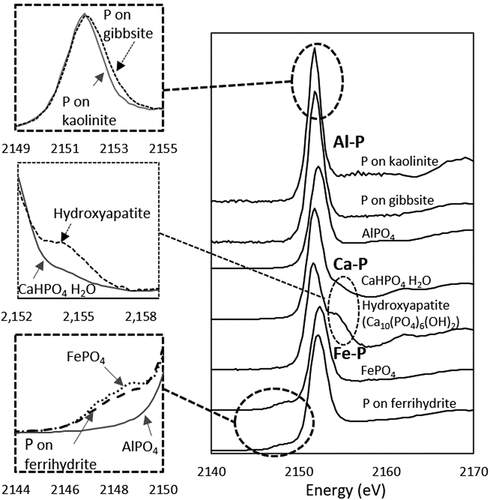
() shows the normalized XANES spectra of selected 14 soil samples with total P contents ranged from 0.8 to 11.8 g P kg−1. The XANES spectra of RL, GL, Yc1 to 4, Ym2, Yf3, and BF were characterized by a shoulder peak on the high-energy side, corresponding to the Ca-P standard. Especially in Yc1 and Yf3, the shoulder peak was distinct and expanded at a higher position of white-line peak than that of CaHPO4 (), corresponding to the spectra feature of hydroxyapatite (). The pre-edge peak was found in RL, Yc3 to 4 and Ym1 indicating the presence of Fe-P. The spectra of BLn, BLh, Yf1, and Yf2 had intense white-line peaks in the narrow energy range corresponding to Al-P.
Figure 3. Phosphorus K-edge XANES spectra of 14 soil samples. Right panels represent the close-up views of post-edge region and white-line peak to compare spectra features of P associated with Ca (Ca-P) and Al (Al-P), respectively. Ca-P, Al-P and Fe-P indicate P associated with Ca, Al and Fe, respectively. BLn: Brown Lowland soils; BLh: Brown Lowland soils with humic layer; RL: Regosolic Lowland soils; GL: Gray Lowland soils; Yc: Yellow soils coarse texture; Ym: Yellow soils medium texture; Yf: Yellow soils fine texture; BF: Brown Forest soils
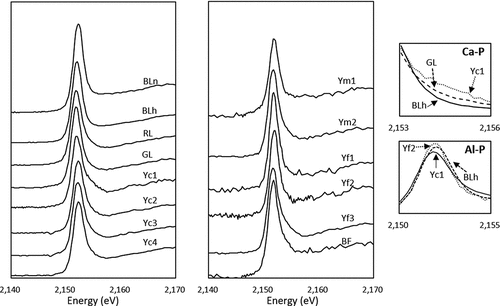
The proportion of P species in soils was calculated by LCF of the XANES spectra by using three standard materials, CaHPO4 as Ca-P, P adsorbed on gibbsite as Al-P and P adsorbed on ferrihydrite as Fe-P (). The LCF revealed that Ca-P was present in all samples, and the proportion of Ca-P was highest in Ym2 and Yf3 and lowest in BLn and BLh. Major P species were Al-P in BLn, BLh, Yf1, and Yf2, while Fe-P in Yc4 and Ym1. However, the XANES spectra were not well fitted by the three standard materials in some soils. The fitted line of Yc1 had a lower post-edge shoulder peak than that of the observed XANES data (). The white-line peak of the fitted line in Yf1 had a lower intensity than that of the observed data (). Hence, the XANES spectra of soil samples were reanalyzed with LCF using other standard materials by adding hydroxyapatite and P adsorbed on kaolinite as standard materials (). The goodness of fit was improved in 5 out of 14 samples by the addition and/or replacement of the standard. The addition of hydroxyapatite to standard compound for LCF improved the fit of shoulder peak in the post-edge region for RL, Yc1 () and Yf3. The shoulder peak in the post-edge region becomes larger with increasing the abundance of Ca in the mineral structure (Ingall et al. Citation2011), decreasing solubility and increasing thermodynamic stability (Sato et al. Citation2005). Therefore, Ca-P in RL, Yc1, and Yf3 may include crystalized calcium phosphate with higher crystallinity than CaHPO4, for example, hydroxyapatite. The addition of P adsorbed on kaolinite as standard materials improved the goodness of fit for Yf1 () and Yf2 because the white-line peak of P adsorbed on kaolinite was narrow compared with that of P adsorbed on gibbsite.
Table 5. Phosphorus K-edge XANES spectra linear combination fitting results showing the relative proportion of P standards giving the best fit to the spectra from the soil samples
Figure 4. Phosphorus K-edge XANES spectra of the best results of linear combination fitting with standard spectra. (a)(b): Linear combination fitting (LCF) using CaHPO4 as Ca-P, PO4 adsorbed on gibbsite as Al-P and P adsorbed on ferrihydrite as Fe-P; (c): LCF result by adding HAP (hydroxyapatite) to the standards used in (a); (d): LCF result by adding Kao (PO4 on kaolinite) to the standards used in (b). Yc: Yellow soils coarse texture; Yf: Yellow soils fine texture
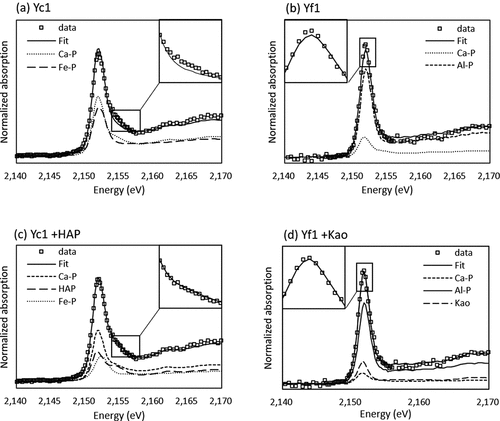
The proportion of P fractions evaluated by chemical extraction and LCF of XANES using standard materials is compared in (). The minor P species less than 5% of total P were difficult to detect by LCF of XANES spectra (Werner and Prietzel Citation2015). Therefore, we did not consider the presence of organic P because organic P evaluated by the wet analyses was less than 10% of total P (). The proportion of Truog-P was positively related to Ca-P, although the plot of Yf3 largely deviated from the 1:1 line (). Based on the LCF results by considering the contribution of hydroxyapatite (), only CaHPO4 (CP) and the sum of CaHPO4 and hydroxyapatite (CP + HAP) are plotted in () as open circles and filled circles, respectively. The proportion of Truog-P was significantly related to the proportion of CaHPO4 (CP) evaluated by LCF fitting of XANES by excluding the contribution of hydroxyapatite. The proportion of AA-P was correlated with that of Ca-P (). The correlation coefficient between the proportions of AA-P and CaHPO4 without the contribution of hydroxyapatite (CP) was lower than that between AA-P and Ca-P or the sum of CaHPO4 and hydroxyapatite (CP + HAP). It was likely that Ca-P species with higher crystallinity than CaHPO4 were extracted by acetic acid in addition to CaHPO4. The proportion of NH4F-P was positively correlated with the summed proportion of Al-P and Fe-P by LCF analyses while it was not related to the proportion of Al-P alone (). Despite the presence of NH4F-P in all the samples, LCF fitting failed to detect a contribution of Al-P to RL, Yc1, Yc2, Yc3, Yc4 and BF (). In addition, the proportion of NH4F-P was higher than that of Al-P (). These results indicated that NH4F-P was associated with not only Al-P but also Fe-P. Since the proportion of NaOH-P was less than 15% except for Yf1 and Yf2 (), it was difficult to detect the contribution of P species associated with NaOH-P by LCF of XANES due to the limitation of sensitivity (Werner and Prietzel Citation2015).
Figure 5. Relationships among the proportions of different P species determined by P K-edge XANES linear combination fitting analysis and chemical fractionation. (a) Truog-P; (b) AA-P; and (c) NH4F-P. Ca-P, Al-P and Fe-P indicate the proportion of CaHPO4, P adsorbed on gibbsite and ferrihydrite calculated by linear combination fitting (LCF), respectively. CP and HAP indicate the proportion of P in CaHPO4 and hydroxyapatite reanalyzed by assuming the presence of hydroxyapatite
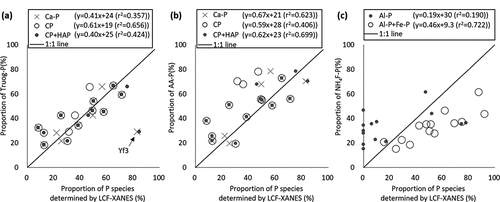
4. Discussion
4.1. Characteristics of P accumulated in eight soil groups
4.1.1. Organic phosphorus
The P concentrations of the cropland soils investigated in this study were high () due to the continuous application of fertilizer (Mishima et al. Citation2013). The accumulated P was dominated by the inorganic form, i.e., orthophosphate (Fig. S1), in accordance with the results of Koch et al. (Citation2018). The ratio of organic P to total P evaluated by NMR and extraction method was at most 12% (, Fig. S1). This result suggested that contribution of organic P to total P in soils was low in the eight soil groups because P had been applied to the soil mainly as inorganic P in chemical fertilizer. Furthermore, in this area, organic fertilizers such as manure were applied once every 2–3 years, and approximately 90% of P in manure compost exists as inorganic form (Sharpley and Moyer Citation2000; Yokota et al. Citation2003). Koch et al. (Citation2018) also reported that the application of organic fertilizer, even over a long period, did not contribute to an increase in the proportion of organic P.
4.1.2. Truog-P and AA-P
Available P is one of the important measures of soil fertility, and the Truog method is widely applied to cropland soil testing in Japan. The major chemical form of Truog-P was P associated with the Ca-bearing phase (). Aye, Araki, and Wada (Citation2011) reported that Truog-P was correlated with the sum of the P fraction extracted by NaHCO3 and NH4OAc, which are operationally defined as the Ca-bearing phase by Jiang and Gu (Citation1989). AA-P extracted by the first step of sequential extraction is operationally defined as Ca-bound P (Sekiya Citation1983), and this was confirmed by XANES analyses ().
The proportion of Truog-P and AA-P to total P was the highest among the P fractions in RL, GL, Yc, Ym, and BF (). Due to the relatively low Alo+1/2Feo for the RL, Yc, Ym, and BF groups (), phosphate adsorption sites such as Al- and Fe-bearing minerals were likely saturated by the application of manure and superphosphate. In addition, the continuous application of lime can also contribute to P that accumulated as calcium phosphate in acidic soils (Condron and Goh Citation1989; Lookman et al. Citation1996). Our results showed that Truog-P and AA-P were higher in the soil with higher exchangeable Ca, total C and pH () and the exchangeable Ca, total C and pH was likely increased due to the application of manure and lime. Hashimoto and Watanabe (Citation2014) also reported that calcium phosphate compounds were not detected in soils with low levels of exchangeable Ca.
The LCF analysis of XANES spectra showed that P associated with Ca was present in all 14 soils (). The addition of hydroxyapatite as a standard material improved the goodness of fit (R) in RL, Yc1, and Yf3, indicating the presence of Ca-P species with higher crystallinity than CaHPO4. Previous studies showed that hydroxyapatite was detected in cropland applied with calcium phosphate fertilizer (Kar et al. Citation2011; Liu et al. Citation2013) and in animal manure compost (Toor et al. Citation2006, Hashimoto et al. Citation2014). The appearance of Ca-P species with higher crystallinity in RL, Yc1 and Yf3 might be attributed to the application of superphosphate and/or animal manure compost.
4.1.3. NH4F-P
NH4F-P is operationally defined as an Al-associated phosphate, which is relatively stable in soil (Sekiya Citation1983). The proportion of NH4F-P was high in BLn and BLh, and the amount of NH4F-P in GL was as high as that in BLn (). These soils were collected from the area near where Non-allophanic Andosols were distributed (), and their Alo + 1/2Feo values were higher than those of the other soil groups (). It was likely that parent materials in BLn, BLh, and GL were partially affected by Quaternary tephra and loess, which were the parent materials of Non-allophanic Andosols in this area (Matsuyama, Saigusa, and Abe Citation1994). The results indicated that the amounts of NH4F-P in soils were related to the amount of Alo and Feo (). The amounts of NH4F-P and Alo +1/2 Feo were also correlated to total C (r = 0.466, p < 0.001 and r = 0.200, p < 0.05, respectively) even when their relationships were analyzed without the data of BLn, BLh and GL groups, which had characteristics of Non-allophanic Andosols. Part of the P added to the soil through the application of manure, which contains water-soluble P (Sato et al. Citation2005), reacted with Al- and/or Fe oxides in the soils and thereby led to the correlation of NH4F-P and Alo +1/2 Feo with total C. It was also reported that Al and Fe contained in manure led to an increase in Alo and Feo in tropical soils with low pH (4.8) and exchangeable Ca (18 mmolc kg−1) and relatively high contents of Alo+1/2 Feo (4.5 g kg−1), and thereby caused increase in P associated with Fe oxide (Abdala et al. Citation2015). In addition to the soil-derived Alo and Feo, manure-derived Alo and Feo might influence the P distribution as NH4F-P.
Based on the relationships between proportions of P determined by LCF analysis of XANES spectra and those of NH4F-P, both Al-P and Fe-P were possible components in NH4F-P (). In the RL, GL, Yc2, Yc3, and Yc4 soils with relatively lower amounts of Alo than Feo, NH4F-P accounted for 28% to 47% of the total P, although the LCF of XANES spectra indicated that contribution of Al-P was negligibly small (). This result suggested that part of the P associated with Fe-bearing minerals was extracted by NH4F. However, further studies are required to elucidate P species associated with Al and Fe because of the difficulty in identifying Fe and Al sorbent phases and the differentiation between adsorption and precipitation by XANES (Eriksson, Gustafsson, and Hesterberg Citation2015), especially when Fe- and Al-bearing phases coexist.
4.1.4. NaOH-P
NaOH-P is operationally defined as a Fe-associated phosphate, which is stable in soil (Sekiya Citation1983). The amounts of NaOH-P were significantly related to all soil properties in except for pH; thus, it was difficult to evaluate what soil properties control the amounts of NaOH-P. A high amount of NaOH-P was found in BLh and Yf with high Ald content and relatively clayey soils among the soil groups (). Since the goodness of fit for the LCF of XANES for Yf1 and Yf2 was low, we added the P adsorbed on kaolinite as a standard material to fit the XANES spectra of these samples (). By adding the contribution of P adsorbed on kaolinite, the goodness of fit was improved. In these areas, kaolinite is one of the widespread clay minerals (Iwata and Kanda Citation1974). Sorption capacity of kaolinite for P is lower than that of Al- and Fe-oxides (Manning and Goldberg Citation1996). Nonetheless, structural Al site of kaolinite can serve as the adsorption site for P when specific surface area associated with kaolinite in soil is higher than gibbsite (Gérard Citation2016). Therefore, kaolinite may provide part of the sorption site for P in Yf1 and Yf2. Although it is often difficult to distinguish P adsorbed or precipitated with different kinds of Al-bearing phases in soil using XANES spectra (Eriksson, Gustafsson, and Hesterberg Citation2015), P associated with different Al minerals has different XANES features (Ingall et al. Citation2011). Further analyses are necessary to confirm the presence of P adsorbed on kaolinite in these samples.
4.2. Comparison of P species evaluated by chemical extraction and LCF of XANES spectra
The P species extracted as Truog-P and AA-P were mainly Ca-P forms, as determined by LCF analysis (), which was consistent with the methodological definition (Sekiya Citation1983; Truog Citation1930). However, the amounts of Truog-P were lower than those of AA-P, especially in RL, Yc1, and Yf3. The goodness of fit of XANES for RL, Yc1 and Yf3 was improved by adding hydroxyapatite as a standard material for fitting in addition to CaHPO4 (). The spectra of Yc1 and Yf3 exhibited a distinct shoulder peak and the shoulder occurred from a higher position of white-line peak than that of CaHPO4, in accordance with the spectra feature of hydroxyapatite (). This result suggested that Ca-P species with higher crystallinity than CaHPO4 could be present in RL, Yc1, and Yf3. By excluding the contribution of hydroxyapatite, Truog-P was well correlated to the proportion of CaHPO4 (CP) evaluated by LCF (). On the other hand, AA-P correlated well with Ca-P without removing the contribution of hydroxyapatite (). These results suggested that Ca-P species with higher crystallinity than CaHPO4 was not dissolved in the 0.001 M H2SO4 used for the extraction of the Truog method but are dissolved in the 0.44 M acetic acid used for the first step of sequential extraction. Based on the comparison with XANES results, P species extracted by 1 M HCl in the sequential extraction method proposed by Hedley, Stewart, and Chauhan (Citation1982) were found to include both CaHPO4 and apatite (Kar et al. Citation2011) or octacalcium phosphate and apatite (Beauchemin et al. Citation2003). Lombi et al. (Citation2006) revealed that exchangeable P evaluated by the isotopic dilution technique was CaHPO4 (monetite). These studies indicated that several different forms of P associated with Ca were extracted by chemical extraction. On the other hand, a recent study on the accuracy of the modified Hedley method reported that extraction by 1 M HCl markedly overestimated the amount of Ca-bound P due to the P redistribution during alkaline extractions and the extraction of occluded P in poorly crystallized minerals (Gu et al. Citation2020). The sequential extraction method proposed by Sekiya (Citation1983) may have an advantage in evaluating P associated with Ca because this method extracts Ca-P in the first step.
The proportion of NH4F-P was correlated more significantly with the sum of Fe-P and Al-P determined by LCF than with only Al-P (). The proportion of NaOH-P was not significantly correlated with any P species determined by LCF (data not shown). These results were different from the operational definition: the P species of NH4F-P and NaOH-P corresponds to P associated with Al and Fe, respectively (Sekiya Citation1983). Nakamura et al. (Citation2019) reported that NH4F-P was correlated with both Al and Fe in the extract of NH4F-P, while NaOH-P was not correlated with either Al or Fe in the extract of NaOH-P. In the Bray method, one of the widely used evaluation methods for available P (Bray and Kurtz Citation1945), soil is extracted with 0.1 M HCl-0.03 M NH4F. This method was designed to dissolve calcium phosphate and a part of P associated with Al and Fe (Bray and Kurtz Citation1945). Therefore, 1 M NH4F used in the second step of sequential extraction by the Sekiya method could possibly dissolve P associated with both Al and Fe, although the concentration of NH4F and the acid conditions in the Bray and Sekiya methods are different.
The proportion of NaOH-P for soils analyzed by XANES was <10%, except for Yf1 and Yf2 (). We could not determine the relationships between NaOH-P and P speciation evaluated by XANES because LCF of XANES cannot evaluate P species at less than 5% (Werner and Prietzel Citation2015). Kar et al. (Citation2011) and Beauchemin et al. (Citation2003) showed that P extracted by 0.1 M NaOH in Hedley sequential extraction method was P associated with both Fe and Al. Our LCF results implied the possible presence of P adsorbed on kaolinite in Yf1 and Yf2 with a high proportion of NaOH-P (). This result suggested that P adsorbed on kaolinite may be one of the P species extracted by NaOH.
4.3. Evaluation of P accumulated in soils for utilization by plants
In 97% of the soil samples collected from 137 vegetable fields in Aichi prefecture, Truog-P exceeded the criterion of proper P level for vegetable production; therefore, application of P fertilizer should be reduced and P accumulated in soils should be utilized by plants. It was reported that crop production did not decrease without P fertilizer in soils with Truog-P > 218 mg P kg−1 (Wada and Tanahashi Citation2017). Furthermore, control of the application of not only P fertilizer but also manure and lime can help to improve P availability in soil by increasing the P associated with Ca in acidic soils, based on the finding that total C and exchangeable Ca control Truog-P ().
Koch et al. (Citation2018) reported that plants could take up stable P by mobilizing it to the available P form. Wheat production was maintained for 15 years after cessation of P fertilizer application in soils with excessive P accumulation because wheat can uptake not only available P extracted by 1 M NH4Cl but also relatively stable P extracted by 0.5 M NH4F (Liu et al. Citation2015). In addition, XANES analyses by Liu et al. (Citation2015) showed a decrease in P associated with Al followed by the increase of Fe-P after crop production because solubility of Al-P was higher than Fe-P in aerobic acid soils (Lindsay and Moreno Citation1960). In order to evaluate P pools that potentially available to plants, the method to discriminate Al-P and Fe-P with different solubility in soil should be needed since NH4F-P included both Al- and Fe-P (). In considering strategies to utilize P accumulated in soil, P speciation is prerequisite information. Our findings indicated that P species accumulated in soils is affected by soil properties which can be estimated from the distribution of parent materials and the application of fertilizer and manure.
Supplemental Material
Download Zip (184.2 KB)Acknowledgments
These experiments were conducted at the BL6N1 of the Aichi Synchrotron Radiation Center, Aichi Science & Technology Foundation, Aichi, Japan. NMR experiment was conducted at Advanced Analyses Center, NARO. We wish to thank Prof. C. S. Langham for English edition.
Disclosure statement
No potential conflict of interest was reported by the authors.
Supplementary material
Supplemental data for this article can be accessed here
Additional information
Funding
References
- Abdala, D. B., I. R. da Silva, L. Vergütz, and D. L. Sparks. 2015. “Long-term Manure Application Effects on Phosphorus Speciation, Kinetics and Distribution in Highly Weathered Agricultural Soils.” Chemosphere 119: 504–514. doi:10.1016/j.chemosphere.2014.07.029.
- Aichi department of agriculture, forestry and fisheries 2016: “Soil Diagnosis Criteria for Crops” 40–63. Aichi prefectural government, Aichi, Japan. (in Japanese)
- Aye, T. A., M. Araki, and S. Wada. 2011. “Evaluation of Five Extraction Methods for Available Phosphorus in Intensively Fertilized Greenhouse Soils.” Journal of the Faculty of Agriculture, Kyushu University 56: 87–92.
- Beauchemin, S., D. Hesterberg, J. Chou, M. Beauchemin, R. R. Simard, and D. E. Sayers. 2003. “Speciation of Phosphorus in Phosphorus-enriched Agricultural Soils Using X-ray Absorption Near-edge Structure Spectroscopy and Chemical Fractionation.” Journal of. Environmental Quality. 32 (5): 1809–1819. doi:10.2134/jeq2003.1809.
- Bray, R. H., and L. T. Kurtz. 1945. “Determination of Total, Organic, and Available Forms of Phosphorus in Soils.” Soil Science 59 (1): 39–45. doi:10.1097/00010694-194501000-00006.
- Cade-Menun, B., and C. W. Liu. 2014. “Solution Phosphorus-31 Nuclear Magnetic Resonance Spectroscopy of Soils from 2005 to 2013: A Review of Sample Preparation and Experimental Parameters.” Soil Science Society Amercian Journal 78 (1): 19–37. doi:10.2136/sssaj2013.05.0187dgs.
- Chang, S. C., and M. L. Jackson. 1957. “Fractionation of Soil Phosphorus.” Soil Science 84 (2): 133–144. doi:10.1097/00010694-195708000-00005.
- Condron, L. M., and K. M. Goh. 1989. “Effects of Long-term Phosphatic Fertilizer Applications on Amounts and Forms of Phosphorus in Soils under Irrigated Pasture in New Zealand.” Journal of Soil Science 40 (2): 383–395. doi:10.1111/j.1365-2389.1989.tb01282.x.
- Courchesne, F., and M. C. Turmel. 2008. “Chapter 26 Extractable Al, Fe, Mn, and Si.” In Soil Sampling and Methods of Analysis, edited by Carter MR, Gregorich EG, 2nded. Canadian Society of Soil Science, 307–317. Boca Raton, Florida, USA.: CRC Press.
- Cultivated soil classification committee. 1995. Classification of Cultivated Soils in Japan (3rd Approximation), 17. Tsukuba, Japan: Miscellaneous publication of the National Institute of Agro-Environmental Sciences.
- Eriksson, A. K., J. P. Gustafsson, and D. Hesterberg. 2015. “Phosphorus Speciation of Clay Fractions from Long-term Fertility Experiments in Sweden.” Geoderma 241–242: 68–74. doi:10.1016/j.geodrma.2014.10.023.
- Gee, G. W., and J. W. Bauder. 1986. “2.4 Particle-size Analysis.” In Methods of Soil Analysis. Part 4―Physical Methods, edited by J. H. Dane and G. C. Topp. SSSA Book Series No. 5, 255–293. Madison, Wisconsin, USA: Soil Science Society of America.
- Gérard, F. 2016. “Clay Minerals, Iron/aluminum Oxides, and Their Contribution to Phosphate Sorption in Soils -A Myth Revisited.” Geoderma 262: 213–226. doi:10.1016/j.geoderma.2015.08.036.
- Gu, C., T. Dam, S. C. Hart, B. L. Turner, O. A. Chadwick, A. A. Berhe, Y. Hu, and M. Zhu. 2020. “Quantifying Uncertainties in Sequential Chemical Extraction of Soil Phosphorus Using XANES Spectroscopy.” Environmental Science and. Technology 54 (4): 2257–2267. doi:10.1021/acs.est.9b05278.
- Hashimoto, Y., A. Takamoto, R. Kikkawa, K. Murakami, and N. Yamaguchi. 2014. “Formations of Hydroxyapatite and Inositol Hexakisphosphate in Poultry Litter during the Composting Period: Sequential Fractionation, P K-edge XANES and Solution 31P NMR Investigations.” Environmental Science and. Technology 48 (10): 5486–5492. doi:10.1021/es404875j.
- Hashimoto, Y., and Y. Watanabe. 2014. “Combined Applications of Chemical Fractionation, Solution 31P-NMR and P K-edge XANES to Determine Phosphorus Speciation in Soils Formed on Serpentine Landscapes.” Geoderma 230–231: 143–150. doi:10.1016/j.geoderma.2014.04.001.
- Hedley, M. J., J. W. B. Stewart, and B. S. Chauhan. 1982. “Changes in Inorganic and Organic Soil Phosphorus Fractions Induced by Cultivation Practices and by Laboratory Incubations.” Soil Science Society of American Journal 46 (5): 970–976. doi:10.2136/sssaj1982.03615995004600050017x.
- Hurtarte, L. C. C., L. F. Souza-Filho, W. O. Santos, L. Vergütz, J. Prietzel, and D. Hesterberg. 2019. “Optimization of Data Processing Minimizes Impact of Self-absorption on Phosphorus Speciation Results by P K-Edge XANES.” Soil Systems 3 (3): 61. doi:10.3390/soilsystems3030061.
- Ingall, E. D., J. A. Brandes, J. M. Diaz, M. D. Jonge, D. Paterson, I. McNulty, W. C. Elliotte, and P. Northrup. 2011. “Phosphorus K-edge XANES Spectroscopy of Mineral Standards.” Journal of. Synchrotron Radiation 18 (2): 189–197. doi:10.1107/S0909049510045322.
- Iwata, H., and S. Kanda. 1974. “Studies on Clay Minerals of Soil in Aichi Prefecture І. Compositions of Clay Minerals in Arable Lands.” Research Bulletin Aichi Agricultural Research Center 6: 151–156. (in Japanese with English summary).
- Jiang, B., and Y. Gu. 1989. “A Suggested Fractionation Scheme of Inorganic Phosphorus in Calcareous Soils.” Fertilizer Research 20 (3): 159–165. doi:10.1007/BF01054551.
- Kar, G., L. S. Hundal, J. J. Schoenau, and D. Peak. 2011. “Direct Chemical Speciation of P in Sequential Chemical Extraction Residues Using P K-edge X-ray Absorption Near-edge Structure Spectroscopy.” Soil Science 176 (11): 589–595. doi:10.1097/SS.0b013e31823939a3.
- Kato, Y. 1970. “Distribution, Soil Profiles and Parent Materials in Andosols in Tokai.” japanese Journal of Soil Science and Plant Nutrition 41: 89–94. in Japanese. doi:10.20710/dojo.41.3_89.
- Kitson, R. E., and M. G. Mellon. 1944. “Colorimetric Determination of Phosphorus as Molybdivanadophosphoric Acid” Industrial & Engineering Chemistry Analytical Edition 16(6): 379–383, doi: 10.1021/i560130a017
- Koch, M., J. Kruse, B. Eichler-Löbermann, D. Zimmer, S. Willbold, P. Leinweber, and N. Siebers. 2018. “Phosphorus Stocks and Speciation in Soil Profiles of a Long-term Fertilizer Experiment: Evidence from Sequential Fraction, P K-edge XANES, and 31P NMR Spectroscopy.” Geoderma 316: 115–126. doi:10.1016/j-geoderma.2017.12.003.
- Komiyama, T., S. Niizuma, E. Fujisawa, and H. Morikuni. 2009. “The Rapid Methods of Total Soil Phosphorus Analysis.” Japanese Journal of Soil Science and Plant Nutrition 80: 616–620. in Japanese. doi:10.20710/dojo.80.6_616.
- Kyle, J. H., A. M. Posner, and J. P. Quirk. 1975. “Kinetics of Isotopic Exchange of Phosphate Adsorbed on Gibbsite.” J. Soil Sci 26 (1): 32–43. doi:10.1111/j.1365-2389.1975.tb01927.x.
- Lindsay, W., and E. Moreno. 1960. “Phosphate Phase Equilibria in Soils.” Soil Science Society of Amercian Journal 24 (3): 177–182. doi:10.2136/sssaj1960.03615995002400030016x.
- Liu, J., J. Yang, B. J. Cade-Menun, X. Liang, Y. Hu, C. W. Liu, Y. Zhao, L. Li, and J. Shi. 2013. “Complementary Phosphorus Speciation in Agricultural Soils by Sequential Fractionation, Solution 31P Nuclear Magnetic Resonance, and Phosphorus K-edge X-ray Absorption Near-edge Structure Spectroscopy.” Journal of Environmental Quality 42 (6): 1763–1770. doi:10.2134/jeq2013.04.0127.
- Liu, J., Y. Hu, J. Yand, D. Abdi, and B. Cade-Menun. 2015. “Investigation of Soil Legacy Phosphorus Transformation in Long-term Agricultural Fields Using Sequential Fractionation, P K-edge XANES and Solution P NMR Spectroscopy.” Environment of Science and Technology 49 (1): 168–176. doi:10.1021/es504420n.
- Lombi, E., K. G. Scheckel, R. D. Armstrong, S. Forrester, J. N. Cutler, and D. Paterson. 2006. “Speciation and Distribution of Phosphorus in A Fertilized Soil: A Synchrotron-based Investigation.” Soil Science Society of Amercian Journal 70 (6): 2038–2048. doi:10.2136/sssaj2006.0051.
- Lookman, R., H. Geerts, P. Grobet, R. Merckx, and K. Vlassak. 1996. “Phosphate Speciation in Excessively Fertilized Soil: A 31P and 27Al MAS NMR Spectroscopy Study.” Euroupean Journal of Soil Science 47 (1): 125–130. doi:10.1111/j.1365-2389.1996.tb01379.x.
- Luo, L., Y. Ma, R. L. Sanders, C. Xu, J. Li, and S. C. B. Myneni. 2017. “Phosphorus Speciation and Transformation in Long-term Fertilized Soil: Evidence from Chemical Fractionation and P K-edge XANES Spectroscopy.” Nutrient Cycling in Agroecosyst. 107: 215–226. doi:10.1007/s10705-017-9830-5.
- Manning, B. A., and S. Goldberg. 1996. “Modeling Arsenate Competitive Adsorption on Kaolinite, Montmorillonite and Illite.” Clays Clay Miner 44 (5): 609–623. doi:10.1346/CCMN.1996.0440504.
- Matsuyama, N., M. Saigusa, and A. Abe. 1994. “Distributions of Allophanic Andosols and Non Allophanic Andosols in Kanto and Chubu Districts.” Japanese Journal of Soil Science and Plant Nutrition 65: 304–312. (in Japanese). doi:10.20710/dojo.65.3_304.
- Mishima, S., S. D. Kimura, S. Eguchi, and Y. Shirato. 2013. “Changes in Soil Available-nutrient Stores and Relationships with Nutrient Balance and Crop Productivity in Japan.” Soil Science and Plant Nutrition 59 (3): 371–379. doi:10.1080/00380768.2013.786646.
- Murphy, J., and J. P. Riley. 1962. “A Modified Single Solution Method for the Determination of Phosphate in Natural Waters.” Analytical Chemical Acta 27: 31–36. doi:10.1016/S0003-2670(00)88444-5.
- Nakamura, Y., K. Ando, A. Tsunekawa, and M. Kasuya. 2019. “Effects of Continuous Use of Livestock Manure on Phosphorus Leaching and Phosphorus Forms in Sandy Upland Field.” Japanese Journal of Soil Science and Plant Nutrition 90: 212–216. (in Japanese). doi:10.20710/dojo.90.3_212.
- Nishigaki, T., S. Sugihara, K. Kobayashi, Y. Hashimoto, M. Kilasara, H. Tanaka, T. Watanabe, and S. Funakawa. 2018. “Fractionation of Phosphorus in Soils with Different Geological and Soil Physicochemical Properties in Southern Tanzania.” Soil Science and Plant Nutrition 64 (3): 291–299. doi:10.1080/00380768.2018.1436406.
- Ohara, H., and M. Nakai. 2004. “Available Phosphate of Arable Lands in Japan. Changes of Soil Characteristics in Japanese Arable Lands (II).” Japanese Journal of Soil Science and Plant Nutrition 75: 59–67. in Japanese). doi:10.20710/dojo.75.1_59.
- Otani, T., and N. Ae. 1999. “Extraction of Organic Phosphorus in Andosols by Various Methods.” Soil Science and Plant Nutrition 45 (1): 151–161. doi:10.1080/00380768.1999.10409331.
- Ravel, B., and M. Newville. 2005. “Data Analysis for X-ray Absorption Spectroscopy Using IFEFFIT.” Journal of Synchrotron Radiatiation 12 (4): 537–541. doi:10.1107/S0909049505012719.
- Sato, S., D. Solomon, C. Hyland, Q. M. Ketterings, and J. Lehmann. 2005. “Phosphate Speciation in Manure and Manure Amended Soils Using XANES Spectroscopy.” Environmental Science and Technology 39 (4): 7485–7491. doi:10.1021/es0503130.
- Schwertmann, U., and R. M. Cornell. 1991. “Ferrihydrite.” In Iron Oxides in the Laboratory. Preparation and Characterization, 89–94. Weinheim: VCH.
- Sekiya, K. 1983. “Phosphorus.” In Methods of Soil Analysis (Dojou Youbun Bunsekihou), edited byy Ministry of Agriculture, Forestry and Fisheries, 225–257. Tokyo, Japan: Youkendou (in Japanese).
- Sharpley, A., and B. Moyer. 2000. “Phosphorus Forms in Manure and Compost and Their Release during Simulated Rainfall.” Journal Environmental Quality 29 (5): 1462–1469. doi:10.2134/jeq2000.0047245002900050021x.
- Soil Survey Staff. 2014. Keys to Soil Taxonomy (12th Ed.). Washington, DC: United States Department of Agriculture & Natural Resources Conservation Service.
- Tani, M., C. Mizota, T. Yagi, T. Kato, and M. Koike. 2010. “Vertical Distribution and Accumulated of Phosphate in Virgin Soils and Arable Soils of Tokachi District, Hokkaido.” Japanese Journal of Soil Science and Plant Nutrition 81: 350–359. (in Japanese). doi:10.20710/dojo.81.4_350.
- Toor, G. S., S. Hunger, J. D. Peak, J. T. Sims, and D. L. Sparks. 2006. “Advances in the Characterization of Phosphorus in Organic Wastes: Environmental and Agronomic Applications.” Advances in Agronomy 89: 1–72. doi:10.1016/S0065-2113(05)89001-7.
- Truog, E. 1930. “The Determination of the Readily Available Phosphorus of Soils.” Journal - American Society of Agronomy 22 (10): 874–882. doi:10.2134/agronj1930.00021962002200100008x.
- Wada, T., and T. Tanahashi. 2017. “Index for Reduction of Phosphate Fertilizer Application to Leaf Vegetables Komatsuna (Brassica Rapa L.) And Spinach (Spinacia Oleracea L.).” Japanese Journal of Soil Science and Plant Nutrition 88: 129–133. (in Japanese). doi:10.20710/dojo.88.2_129.
- Werner, F., and J. Prietzel. 2015. “Standard Protocol and Quality Assessment of Soil Phosphorus Speciation by P K-Edge XANES Spectroscopy.” Environmental Science Technology 49 (17): 10521–10528. doi:10.1021/acs.est.5b03096.
- Wright, A. L. 2009. “Soil Phosphorus Stocks and Distribution in Chemical Fractions for Long-term Sugarcane, Pasture, Turfgrass, and Forest Systems in Florida.” Nutrient Cycling in Agroecosyst 83 (3): 223–231. doi:10.1007/s10705-008-9213-z.
- Yokota, T., T. Ito, T. Ono, M. Takahashi, and M. Saigusa. 2003. “Composition of Inorganic Phosphate in Cattle Manure Compost with Different Productions.” Japanese Journal of Soil Science and Plant Nutrition 74: 133–140. (in Japanese). doi:10.20710/dojo.74.2_133.