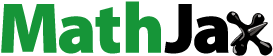
ABSTRACT
The purpose of this study is to estimate the contribution of water-derived 137Cs in the total activity of 137Cs in brown rice collected from Minamisoma, Fukushima. Three different methods used for the estimation were: 1) the concentration ratio of 137Cs from water to brown rice (CR–water–rice), 2) mass balance ratio of the total activity of 137Cs from water to brown rice (MB–water–rice), and 3) the ratio of 137Cs/133Cs. To obtain water-to-brown rice transfer parameters (CR–water–rice and MB–water–rice), rice plants were grown in pots filled with soil collected from Minamisoma, Fukushima and irrigated with three types of water (tap water free of 137Cs containing 0.39 mg L−1 K+, tap water free of 137Cs containing 1.0 mg L−1 K+, and tap water containing 5.6 Bq L−1 137Cs and 1.0 mg L−1 K+). The activity concentrations of 137Cs in irrigation water, soils, and brown rice collected from Minamisoma, Fukushima were 0.032 Bq L−1, 1.04, and 0.0012 Bq g−1 dry wt, respectively. Water-derived 137Cs in brown rice only accounted for 1.9% estimated from the CR–water–rice parameter. It was 2.8% if estimated with the MB–water–rice parameter. The ratio of 137Cs/133Cs in brown rice was 0.00162 Bq ng−1, similar to that in the exchangeable fraction of soils (0.00173 ± 0.00007 Bq ng−1, n = 3). Thus, the contribution of 137Cs from irrigation water to brown rice was negligible. The results estimated by three different methods were in good agreement. Therefore, these methods will be useful tools for assessing the contribution of water-derived 137Cs in the total activity of 137Cs in brown rice collected from Minamisoma, Fukushima. The estimation using 137Cs/133Cs ratio can also be applicable for rice in other regions.
1. Introduction
Radiocaesium exists in irrigation water supplied to the paddy field as dissolved and suspended factions. It was reported that radiocaesium in irrigation water resulted from the 2011 Fukushima nuclear accident is mostly in the suspended form, which is limited to the uptake of rice plants (Yoshikawa et al. Citation2014; Tsukada and Ohse Citation2016). Many irrigation water reservoirs contaminated with radiocaesium are derived from contaminated litter deposited in the mountain forests (Nemoto and Abe Citation2013).
Rice plants can take up radiocaesium from irrigation water and soil. The activity concentration of radiocaesium in brown rice taken up from the soil significantly decreased since the 2011 Fukushima nuclear accident (Tagami et al. Citation2018). Since 2015, the level of radiocaesium in brown rice collected in Fukushima Prefecture has not been found to exceed the regulatory standard (100 Bq kg−1) (Ministry of Agriculture, Forestry and Fisheries July Citation2021a). The reduction was not only due to countermeasures, such as applying potassium fertilizer, decontamination works (Nobori et al. Citation2014; Kato et al. Citation2015; Yamaguchi et al. Citation2016), but also a decrease in the exchangeable fraction of radiocaesium because of the fixation of 137Cs in the frayed edge sites (Krouglov et al. Citation1997; Tsukada et al. Citation2008; Tsukada Citation2014; Yang et al. Citation2016; Yamaguchi et al. Citation2017).
The uptake of 137Cs by the rice plant from irrigation water is due to the direct absorption of dissolved 137Cs via the stem base and through superficial roots. It was indicated that the contribution of newly added 137Cs from irrigation water to the paddy fields is negligible (Tanaka et al. Citation2013; Yoshikawa et al. Citation2014; Yang et al. Citation2016), and 95% of radiocaesium in irrigation water strongly binds to the suspended particles, which is not available for uptake to rice plants (Tsukada et al. Citation2017). However, 137Cs in water are more available for rice than that in soils (Nemoto and Abe Citation2013). Myttenaere, Bourdeau, and Masset (Citation1969) reported that radiocaesium absorbed by flooded rice from the water was 30 to 100 times higher than that from the soil, and the absorption of 137Cs by rice plants is greater through the shoot base than the roots when dipping the shoot and root into a nutrient solution (Myttenaere Citation1972). The increase in the uptake of 137Cs through the shoot base of the rice plant was also reported by D’Souza’s and Mistry (Citation1980) and Uematsu et al. (Citation2017). Moreover, the activity concentration of radiocaesium in brown rice was proportional to an increase in the activity concentration of radiocaesium in water (Suzuki et al. Citation2015). The rice plant is usually flooded with 5–15 cm of water during the crop cultivation season (IAEA Citation2009), and the amount of water needed for the rice paddy field is about 2000 mm per season on average in Japan (Yoshikawa et al. Citation2020). Therefore, radiocaesium in irrigation water is considered an important factor to increase the accumulation of radiocaesium in the rice plant (Nakanishi, Kobayashi, and Tanoi Citation2013; Yang et al. Citation2016). In addition, Tsukada et al. (Citation2017) reported the dissolved fraction of radiocaesium in water collected within the 80 km zone around TEPCO’s Fukushima Daiichi Nuclear Power Station (FDNPS) varied from 0.00075 to a very high level of 6.7 Bq L−1. If the paddy field continues being supplied with irrigation water containing a certain level of radiocaesium, the contributions of water-derived and soil-derived 137Cs in rice should be taken into account and clarified for assessing the fate of 137Cs in the agricultural environment.
Stable cesium (133Cs), like radiocaesium, mostly exists in the soil as a fraction that is strongly bound to the soil because it is trapped in the interlayer exchange sites in clay mineral lattices (Wampler et al. Citation2012). The amount of 133Cs in the exchangeable fraction of soils was much lower than that of exchangeable 137Cs in soils (Oughton et al. Citation1992; Tsukada et al. Citation2008). There were several percentages of exchangeable 133Cs in soils while the 137Cs content in the exchangeable fraction in soils was approximately 10% (Tsukada et al. Citation2008). Although the transfer factors of radiocaesium from soils to brown rice and polished rice are several times higher than those of 133Cs (Komamura and Tsumura Citation1994; Tsukada et al. Citation2002a), the approximate isotopic equilibrium between 137Cs and 133Cs in the bioavailability fraction was expected to obtain, and 133Cs in the agricultural field can be a useful analogy in predicting the long-term fate of 137Cs (Tsukada et al. Citation2002a; Tsukada, Hasegawa, and Yamasaki Citation2002b; Uchida and Tagami Citation2007).
In this study, we estimated the contribution of water-derived 137Cs in the total activity of 137Cs in brown rice cultivated at paddy fields in Minamisoma, Fukushima. The estimation was based on three different methods. Method 1 is the concentration ratio of 137Cs from water to brown rice (CR–water–rice). This parameter is the ratio of 137Cs activity concentration in brown rice taken up from water divided by the activity concentration of 137Cs in water. Method 2 is the mass balance ratio of the total activity of 137Cs from water to brown rice (MB–water–rice). This parameter is the ratio of the total activity of 137Cs in brown rice taken up from water divided by the total activity of 137Cs in water. The CR–water–rice and MB–water–rice would be obtained by cultivating rice with the pot experiment. Method 3 is based on the ratios of 137Cs/133Cs in brown rice, the exchangeable fraction of soils, and irrigation water. The results estimated from three methods would be compared to assess the reliability of the methods.
2. Materials and methods
2.1. Preparation for soils and water for the pot experiment
Soils collected from a paddy field in Minamisoma, which is gray lowland soils, were dried at 50°C for 25 days to obtain the constant weight. Dried soils were passed through a 2 mm sieve, and mixed homogeneously by a V-blender (V type blender, Tokuju). The physical and chemical properties of soils were determined and shown in . Radiocaesium interception potential (RIP) was referred from Yamaguchi et al. (Citation2017). The average RIP value of soils in the paddy field in Minamisoma, Fukushima is 1308 ± 719 mmol kg−1 (n = 76).
Table 1. Physical and chemical properties of the soils in the pot experiment (mean ± sd, n = 3)
The 137Cs-contaminated leaves collected near the Ohgaki dam in December 2018 were used to prepare for 137Cs-contaminated water supplied to rice plants. The leaves were soaked into tap water and kept in a refrigerator at 5°C for three days. Then, the residue of leaves was removed, and the obtained solution was filtered through a 0.45 µm membrane filter (Stericup® Quick Release, Merck Millipore). The activity concentration of 137Cs in the monovalent ionic cation Cs+ in the dissolved fraction was determined with an Empore TM Cesium Rad Disk (3 M). Two disks were used to collect 137Cs+ in the dissolved fraction because the yield of Cs+ was over 95% (Tsukada et al. Citation2017). The obtained result indicated that 92% of 137Cs in the filtered solution was in the form of monovalent ionic cation 137Cs+. After that, the solution was kept in the refrigerator at 5°C, and diluted each time when supplying to rice plants.
2.2. Pot cultivation
A 3.2 kg of soils mixed with 6.64 (g) of N: P: K fertilizer with a ratio of 15:12:10 were added into a Wagner pot (n = 9) (1/5000 a, Fujiwara Scientific), and the ratio of potassium content from fertilizer to the soil in each pot was 25 mg K2O 100 g−1 dry soils. The concentrations of 137Cs and 133Cs in soils are shown in . Nine germinated rice seeds (Oryza sativa L.) were transferred into the pot on 16 June 2020. After that, four smaller rice plants were taken out of each pot so that the amount of fertilizer can be enough for the growth of rice plants. Three types of irrigation water supplied to the pots were: 1) tap water free of 137Cs and containing 0.39 mg L−1 K+ for pot A, 2) tap water free of 137Cs and containing 1.0 mg L−1 K+ for pot B, and 3) tap water containing 5.6 Bq L−1 137Cs and 1.0 mg L−1 K+ for pot C (). The potassium contents in water supplied to rice in the pot experiment were 0.39 mg L−1 and 1.0 mg L−1 to ensure that the potassium concentrations range within the range of the potassium concentrations in river water in Japan (Uchida et al. Citation2007).
Table 2. The concentrations of 137Cs and 133Cs in soils, as well as the exchangeable fraction of soils in the pot experiment (mean ± sd, n = 3)
Table 3. The concentrations of cations, 137Cs, and 133Cs in water supplied to the pots
The experiments were performed with each type of water in triplicate. The activity concentration of 137Cs in public tap water in Fukushima reported it was almost below the detection limit (Shizuma et al. Citation2018). Rice plants were supplied with water every two days throughout the growing season, and the total amount of water irrigated for each pot was 30 L, which is approximately 1500 L m−2.
Rice was harvested on 7 October 2020. Soils in the pots supplied with 137Cs-contaminated water were also collected using a soil core sampler (HSC-20, Fujiwara Scientific). The cores were cut into a section of 1 cm from 0 to 4 cm of the depth, a section of 2 cm from 4 to 8 cm of the depth, and the rest of the core. Then, samples were dried at 50°C for 27 days before being passed through a 2 mm sieve.
Rice plants were separated into leaves, straws, rice hulls, and brown rice. The leaf and straw samples were washed with tap water to remove adhered soil particles, and dried in an oven at 70°C for 20 days before being pulverized by a stainless steel cutter blender (7011 HBC, Waring Commercial). A small amount of soil and rice was grinded into powder by a Mixer Mill (MM 200, Retsch) before decomposing samples to measure 133Cs.
2.3. Sample collection from the paddy field in Minamisoma, Fukushima
In order to estimate the contribution of 137Cs derived from irrigation water in the total 137Cs in brown rice cultivated at the paddy field, irrigation water supplied to the paddy field in Minamisoma was collected in June 2020. Brown rice and three soil cores were collected on 23 September 2020. Soil cores were cut into a section from 0 to15 cm, and the rest of the core. The sections of 15 cm in depth were used for the study because the roots of the rice plant distribute within this horizon. The treatments for soils and rice are similar to those for soils and rice components collected from the pots.
The treatment for irrigation water collected in Minamisoma was done based on a method published by Tsukada et al. (Citation2017). Irrigation water was passed through a 0.45 µm pore-size membrane filter (HVLP09050, Merck Millipore) to separate the dissolved and suspended fractions of 137Cs. The activity concentration of 137Cs was determined in the dissolved fraction.
2.4. Sample analysis
Samples after being treated were transferred into a container (U-8) for measuring the activity concentration of 137Cs using a Ge detector connected to a multichannel analyzer system (GC 4020, Canberra) at the energy of 662 keV. The dependency of the detection efficiency of the Ge detector on the sample thickness was calibrated using a mixed standard radionuclide material manufactured by the Japan Radioisotope Association. Statistical measurement uncertainties of all samples did not exceed 10% of the measured results.
137Cs and 133Cs in the exchangeable fractions of soils were extracted based on a published method by Tsukada et al. (Citation2008). The concentrations of 137Cs and 133Cs in the exchangeable fraction are given in .
Grinded soils (20–100 mg) and rice components (500 mg) were put in a Teflon pressure decomposition vessel with a mix of acid HNO3 69.0%–70.0% (for trace analysis, Kanto Chemical), and HF 49.5%–50.5%. Grinded brown rice was decomposed by nitric acid (68.0 wt. %) of high purity grade (TAMAPURE AA-100, Tama Chemicals) and HF 49.5%–50.5%. A mix of sample and acid was decomposed by a microwave sample preparation system (Titan MPSTM, Perkinelmer) for 1 h. The sample was evaporated to dryness after the digestion, and then the residue was dissolved with 1 mL of HNO3 65% before diluting to 20 mL with deionized water. Stable cesium in digested samples, exchangeable fractions of soils, and water was determined by inductively coupled plasma-mass spectrometry (ELAN DRC2, Perkinelmer). A standard solution of Indium 30 ng mL−1 was used to compensate for the changes in analytical signals during the operation of ICP-MS. Reference materials of the International Atomic Energy Agency (IAEA) used to validate the decomposing and analytical procedures were Soil-7 and V-10 Hay. The analytical values of stable Cs in the standard samples were in agreement with the certified values. The measured results of Soil-7 and V-10 Hay were 5.3 ± 0.1 (n = 4) and 0.0167 ± 0.0008 mg kg−1 dry wt (n = 4), respectively, which are within the confidence intervals of 4.9–6.4 mg kg−1 dry wt for Soil-7 and 0.016–0.019 mg kg−1 dry wt for V-10 Hay.
The concentrations of potassium, ammonium, sodium, magnesium and calcium cations in water supplied to the pots and irrigation water in Minamisoma were measured with ion chromatography (ICS-1100, Thermo Scientific Dionex).
2.5. Transfer factor of 137Cs from soils to brown rice
Transfer factor of 137Cs from soils to brown rice () is defined as the ratio of the dry weight (dry wt) concentration in brown rice to the dry weight concentration in the specified soil layer (Tsukada et al. Citation2002a; IAEA Citation2010). In the pot experiment, after mixing well soils by the V-blender, the activity concentration of 137Cs in soils (0.43 Bq g−1 dry wt) was for calculating the soil-to-brown rice transfer factor of 137Cs.
2.6. Concentration ratio and mass balance ratio of the total activity of 137Cs from water to brown rice
The concentration ratio of 137Cs from water to brown rice ( is the ratio of 137Cs activity concentration in brown rice taken up from water divided by the activity concentration of 137Cs in water.
The mass balance ratio of the total activity of 137Cs from water to brown rice () is the ratio of the total activity of 137Cs in brown rice taken up from water divided by that in water.
2.7. Estimate the contribution of water-derived 137Cs in the total 137Cs in brown rice collected from the paddy field in Minamisoma
2.7.1. The estimation is based on the 
parameter (Method 1)
Method 1 (M1): The percentage of 137Cs in brown rice derived from water can be estimated from the parameter as follows:
2.7.2. The estimation is based on the 
parameter (Method 2)
Method 2 (M2): The percentage of 137Cs in brown rice derived from water can be estimated from the parameter as follows:
The average yield of brown rice is 0.5 kg m−2 (Ministry of Agriculture, Forestry and Fisheries November Citation2021b), and the total volume of water is 1000 L m−2 (Eguchi Citation2017).
2.7.3. The Estimation is based on the ratio of 137Cs/133Cs (Method 3)
Method 3 (M3): The total number of 137Cs (Bq) and 133Cs (ng) taken up by brown rice from water and soils can be expressed by EquationEquations (6)(6)
(6) and (Equation7
(7)
(7) ).
With
where
137Csbrown rice and 133Csbrown rice are the concentrations of 137Cs (Bq g−1, dry wt) and 133Cs (ng g−1, dry wt) in brown rice, respectively.
137Cswater and 133Cswater are the concentrations of 137Cs (Bq L−1) and 133Cs (ng L−1) in water, respectively.
137Cssoil-ex and 133Cssoil-ex are the concentrations of 137Cs (Bq g−1, dry wt) and 133Cs (ng g−1, dry wt) in the exchangeable fraction of soils, respectively.
Vwater is the total amount of water supplied (L).
mbrown rice is the total weight of brown rice after being harvested (g, dry wt).
msoil is the total amount of soils in each pot (g, dry wt).
is the factor indicating that distributions of 137Cs and 133Cs from water can be absorbed by the rice plant.
is the factor indicating that distributions of 137Cs and 133Cs from soils can be taken up by the rice plant.
EquationEquations (6)(6)
(6) and (Equation7
(7)
(7) ) are modified into Equationequation 8
(8)
(8) as follows:
=
where
and
are the contributions of water-derived 137Cs and soil-derived 137Cs in the total 137Cs in brown rice.
Therefore, the ratio of 137Cs/133Cs can be applied to estimate the percentage of water-derived 137Cs in the total 137Cs in brown rice.
3. Results and discussion
3.1. The concentrations of 137Cs and 133Cs in rice plants cultivated in the pot experiment
There was no significant difference in the dry biomass of brown rice among pots A, B, and C (P > 0.05) (). The activity concentrations of 137Cs in rice components are given in . There were no significant differences in the 137Cs activity concentrations of rice components between pot A and B (P > 0.05). The activity concentrations of 137Cs in brown rice, rice hull, and rice straws (stems and leaves) in pot C were 0.006 ± 0.002, 0.020 ± 0.004, and 0.018 ± 0.004 Bq g−1 (dry wt.), respectively. These results were around 3 times higher than those supplied with tap water (P < 0.05). Brown rice was the part that accumulated the lowest level of 137Cs. On the other hand, the activity concentration of 137Cs in rice straw was similar to that in rice hulls.
Table 4. Total dry mass, transfer factors of 137Cs from soils to brown rice (pots A and B) and transfer parameters of 137Cs from water to brown rice (mean ± sd, n = 3, except TFsoils-rice: mean ± sd, n = 6)
Figure 1. Activity concentrations of 137Cs in rice components. The standard deviation (n = 3) is represented by the vertical lines. The symbols (?, ??) indicate the significant differences in the activity concentrations of 137Cs in rice components between pots A, B, and pot C (P < 0.05).
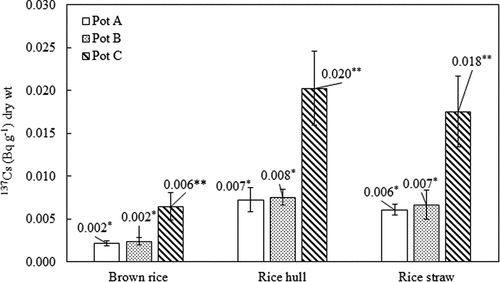
The concentrations of 133Cs in rice components are shown in . Brown rice was the component that accumulated the lowest level of 133Cs compared to those in rice hull and rice straw, similar to the tendency of 137Cs. The distribution of 133Cs in rice components, which is similar to that of 137Cs, was also reported by previous studies (Tsukada, Hasegawa, and Yamasaki Citation2002b; Wakabayashi, Itoh, and Takahashi Citation2017). Therefore, this implies that the translocation of 137Cs in rice is similar to that of 133Cs (Tsukada, Hasegawa, and Yamasaki Citation2002b). The significant differences in the accumulation of 133Cs by rice components between pots A, B, and C were not observed () (P > 0.05) even though the 133Cs concentration in water supplied to pot C was 29 ng L−1, which is two times higher than that in water supplied to pots A and B (14 ng L−1).
Figure 2. Concentrations of 133Cs in rice components. The standard deviation (n = 3) is represented by the vertical lines.
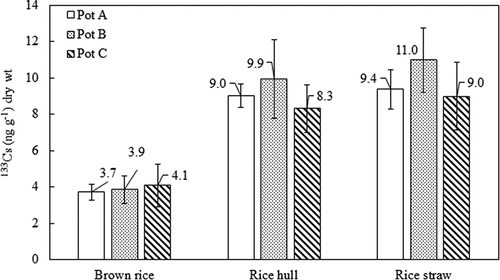
The ratios of 137Cs/133Cs in rice components in pots A and B are shown in . The values ranged from 0.0006 to 0.0008 Bq ng−1, similar to that of 137Cs/133Cs in the exchangeable fraction of soils (0.00072 Bq ng−1). This is because the activity concentration of 137Cs in tap water supplied to rice in pots A and B could not be detected. Therefore, the uptake of 137Cs by rice plants from soils can be predicted from the ratio of 137Cs/133Cs in the exchangeable fraction of soils. Tsukada, Hasegawa, and Yamasaki (Citation2002b) also suggested that 133Cs can be a useful analog to predict the long-term change of 137Cs in the agricultural environment. On the other hand, the ratios of 137Cs/133Cs in brown rice, rice hull, and rice straw in pot C were 0.002, 0.003, 0.002 Bq ng−1, which were one order of magnitude higher than those in pots A and B (). This is because the concentration of 137Cs in the rice plant in pot C was contributed by 137Cs from soils and water.
3.2. Transfer of 137Cs from soils, and water to brown rice in the pot experiment
The transfer factor of 137Cs from soils to brown rice was calculated for pots A and B, and the average value was 0.0053 (). Uchida et al. (Citation2005) referenced and summarized the geometric means of the transfer factor of 137Cs from soils to brown rice and polished rice collected in 1999 were 0.0026 and 0.0011, respectively. Tsukada et al. (Citation2002a) reported the geometric mean of the transfer factor of 137Cs derived from the global fallout deposition for polished rice cultivated in Aomori was 0.0016, and its 95% confidence interval was 0.00012–0.012 while the values of brown rice planted in all Japan were from 0.003–0.06 (Uchida and Tagami Citation2007). Therefore, the transfer factor of 137Cs from soil to brown rice in our study is within the range of values reported for the soil-to-brown rice transfer factor of 137Cs derived from the global fallout deposition.
The 137Cs transfer factors reported for brown rice and polished rice planted in Fukushima paddy fields following the 2011 nuclear accident were relatively higher than those reported for rice planted before March 2011. The values reported for brown rice and polished rice cultivated at Minamisoma City in May 2011 were 0.013–0.017 and 0.005–0.013, respectively (Endo, Kajimoto, and Shizuma Citation2013), and the values were still increased in 2012. However, the transfer factor of 137Cs from soils to brown rice in the subsequent years returned rapidly to values similar to those before the accident happened because 137Cs had approached equilibrium conditions in soils (IAEA Citation2020).
The transfer parameters CR–water–rice (EquationEquation (2)(2)
(2) ) and MB–water–rice (EquationEquation (3)
(3)
(3) ) of 137Cs from water to brown rice are given in . We calculated the
parameter from the data published by Suzuki et al. (Citation2015). The calculated values and the exchangeable potassium are plotted in . When the exchangeable potassium is 37 mg kg−1, the
is very high. However, the
value decreases quickly with increasing in the exchangeable potassium in soils. Therefore, the exchangeable potassium in soils could control the uptake of 137Cs by rice from water. Saito et al. (Citation2012), Kato et al. (Citation2015), and Tsukada and Ohse (Citation2016) also reported that the transfer factor of 137Cs from soils to brown rice decreased with an increase in the exchangeable potassium in soils. For this reason, the transfer of 137Cs from soils and water to rice can be depressed by applying potassium fertilizer.
Figure 4. The relationship between the CR−water-rice parameter and exchangeable K in soils. The K content in fertilizer was also included in the exchangeable K in our study. The triangles represent the CR−water-rice parameters calculated from the data of Suzuki et al. (Citation2015). The circle represents the CR−water-rice parameter in this study.
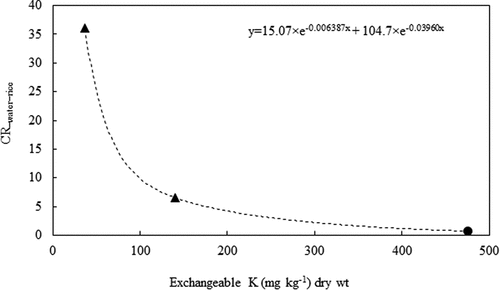
After harvesting rice, the activity concentration of 137Cs in soils collected from pot C was measured. The activity concentration of 137Cs in a 0–1 cm soil layer was 0.69 ± 0.05 Bq g−1 dry wt. However, the activity concentrations of 137Cs in the underneath soil layers were similar to those before the cultivation (). It suggests that 137Cs in water is transferred to the rice plant and also absorbed on the surface soil.
Figure 5. The activity concentration of 137Cs in soils collected from pot C after harvesting rice. The standard deviation (n = 3) is represented by the vertical lines. The symbols (?, ??) indicate the significant differences in the activity concentration of 137Cs between the 0-1 cm soil layer and the underneath soil layers.
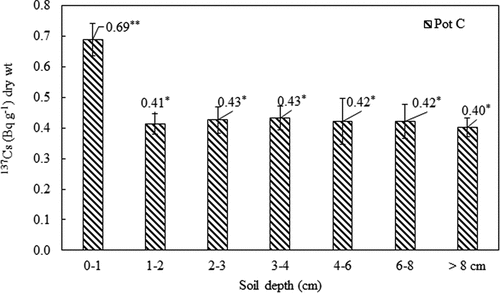
3.3. Contribution of water-derived 137Cs in brown rice collected from Minamisoma
The concentrations of dissolved 137Cs,133Cs, and cations in the water inlet supplied to the paddy field observed in 2020 are shown in . The 137Cs activity concentrations in the soil, the exchangeable fraction of soils, and brown rice are given in . The contributions of water-derived 137Cs in the total 137Cs in brown rice estimated from the CR–water–rice (0.73) and MB–water–rice (0.0005) parameters were only 1.9% and 2.8%, respectively. With the estimation based on M3, the ratio of 137Cs/133Cs in the exchangeable fraction of soils was 0.00173 ± 0.00007 Bq ng−1 (n = 3) while the value of 137Cs/133Cs in irrigation water was 0.00613 Bq ng−1. However, the ratio of 137Cs/133Cs in brown rice did not increase. It was only 0.00162 Bq ng−1, which is similar to that in the exchangeable fraction of soils, and much lower than that in irrigation water. EquationEquations (6(6)
(6) ) & (Equation7
(7)
(7) ), show that if the contributions of 137Cs and 133Cs from irrigation water to brown rice are negligible, the ratio of 137Cs/133Cs in brown rice will be similar to that in the exchangeable fraction of soils. Therefore, it can be concluded that the contribution of 137Cs from irrigation water to brown rice cultivated at the paddy field in Minamisoma, Fukushima is negligible because the activity concentration of 137Cs in irrigation water was very low, and the result estimated by M3 is in good agreement with those estimated by M1 and M2.
Table 5. The concentrations of 137Cs, 133Cs, and other cations in irrigation water that was collected from Minamisoma, Fukushima
Table 6. The concentrations of 137Cs and 133Cs in soils, the exchangeable fraction of soils, and brown rice that was collected from Minamisoma, Fukushima (mean ± sd, n = 3)
The newly added 137Cs from irrigation water into the paddy field was very small compared to the amount already present in the paddy soils. It was estimated that 137Cs added to the paddy fields in the Towa region of Nihonmatsu from irrigation water only accounted for 0.03%–0.05% of the amount already present in the soil (Yoshikawa et al. Citation2014). This was also found in the paddy field in Oguni, Date, Fukushima (Tsukada and Ohse Citation2016). Thus, the water sources in this area can be used for irrigation to the paddy fields. However, the level of 137Cs in water reservoirs in evacuation zones is still high, the continuous monitoring and water management need to be taken into consideration. Transfer parameters of 137Cs from water to brown rice can be used to assess the contribution of 137Cs derived from irrigation water in the total 137Cs in brown rice cultivated in Minamisoma while the ratio of 137Cs/133Cs can be applied for assessing the contribution of water-derived 137Cs in the total 137Cs in brown rice in many other regions because this method can eliminate the effect of the exchangeable potassium in soils on the uptake of 137Cs by rice plants.
4. Conclusions
The contribution of 137Cs derived from irrigation water in the total 137Cs in brown rice cultivated in Minamisoma, Fukushima was negligible. The estimated contributions based on CR–water–rice and MB–water–rice were only 1.9% and 2.8%, respectively. The estimation based on the ratio of 137Cs/133Cs indicated that the contribution of 137Cs from irrigation water to brown rice was negligible. Therefore, the results estimated by the methods were in good agreement, and irrigation water sources in Minamisoma can be used in paddy fields. The method of water-to-brown rice transfer parameters can be applied for the preliminary assessment for the contribution of water-derived 137Cs in the total 137Cs in brown rice at the paddy field in Minamisoma because these parameters are affected by the exchangeable potassium in soils. On the other hand, the calculations in the method of the ratio of 137Cs/133Cs can eliminate the effect of the exchangeable potassium in soils on the uptake of 137Cs by rice plants. It is due to the activity concentration of 137Cs divided by the concentration of 133Cs. Therefore, the application of this method can be for rice plants not only cultivated in Minamisoma, Fukushima but also cultivated in many other regions.
Acknowledgments
We would like to thank Drs T. Saito and Y. Yagasaki, Mr. Kano, Ms. Naomi, and Ms. Chiba for their support with sample collection and treatment. We thank professor Wada for providing us with 137Cs contaminated leaves and for sharing his experience extracting 137Cs from leaves, as well as Dr. Nihei for providing us with N:P:K fertilizer. We are grateful to Dr. N. H. Thu for her insightful comments on the manuscript. We appreciate Ms. Ohtsuki, Ms. Kikuchi, and Mr. Okizawa’s help in setting up equipment for the rice cultivation, as well as Ms. Goto, and Mr. Kuchiki’s support and sharing of ICP-MS measurement experience. We are deeply grateful to Mr. Sato, a farmer who provided us with soil samples and allowed us to collect samples in his paddy field.
Disclosure statement
No potential conflict of interest was reported by the author(s).
References
- D’Souza, T. J., and K. B. Mistry. 1980. “Absorption of Gamma-emitting Fission Products and Activation Products by Rice under Flooded and Unflooded Conditions from Two Tropical Soils.” Plant Soil 55: 189–198. doi:10.1007/BF02181798.
- Eguchi, S. 2017. “Behavior of Radioactive Cesium in Agricultural Environment.” Journal of the Japanese Society of Soil Physics 135: 90–23.
- Endo, S., T. Kajimoto, and K. Shizuma. 2013. “Paddy-field Contamination with 134Cs and 137Cs Due to Fukushima Dai-ichi Nuclear Power Plant Accident and Soil-to-rice Transfer Coefficients.” Journal of Environmental Radioactivity 116: 59–64. doi:10.1016/j.jenvrad.2012.08.018.
- IAEA. 2009. “Quantification of Radionuclide Transfer in Terrestrial and Freshwater Environments for Radiological Assessments.” IAEA TECDOE-1616. Vienna: International Atomic Energy Agency.
- IAEA. 2010. Handbook of Parameter Values for the Prediction of Radionuclide Transfer in Terrestrial and Freshwater Environments. No. 472. Vienna: International Atomic Energy Agency.
- IAEA. 2020. Environmental Transfer of Radionuclides in Japan following the Accident at the Fukushima Daiichi Nuclear Power Plant. Vienna: IAEA-TECDOC-1927, International Atomic Energy Agency.
- Kato, N., N. Kihou, S. Fujimura, M. Ikeba, N. Miyazaki, Y. Saito, T. Eguchi, and S. Itoh. 2015. “Potassium Fertilizer and Other Materials as Countermeasures to Reduce Radiocesium Levels in Rice: Results of Urgent Experiments in 2011 Responding to the Fukushima Daiichi Nuclear Power Plant Accident.” Journal of Soil Science and Plant Nutrition 61 (2): 179–190. doi:10.1080/00380768.2014.995584.
- Komamura, M., and A. Tsumura. 1994. “Transfer Factors of Long-lived Radionuclides from Soil to Polished Rice Measured by ICP-MS.” Radio Isotopes. 43. 1–8. In Japanese, with English abstract.
- Krouglov, S. V., A. S. Filipas, R. M. Alexakhin, and N. P. Arkhipov. 1997. “Long-term Study on the Transfer of 137Cs and 90Sr from Chernobyl-contaminated Soils to Grain Crops.” Journal of Environmental Radioactivity 34 (3): 267–286. doi:10.1016/0265-931X(96)00043-4.
- Ministry of Agriculture, Forestry and Fisheries. 2021a. “Results of Inspections of Radioactivity Levels in Agricultural Products.” Accessed 2021 July 2, in 2021 July 2. http://www.maff.go.jp/j/kanbo/joho/saigai/s_chosa/
- Ministry of Agriculture, Forestry and Fisheries. 2021b. “Annual Yield per 10a of Paddy Field Produced in 2019 by Perfectures.” Accessed 2021 November 9, in 2021 November 9. https://www.maff.go.jp/j/press/tokei/seiryu/attach/pdf/190315-3.pdf
- Myttenaere, C., P. Bourdeau, and M. Masset. 1969. “Relative Importance of Soil and Water in the Indirect Contamination of Flooded Rice with Radiocaesium.” Health Physics 16 (6): 701–707. PMID: 5795252. doi:10.1097/00004032-196906000-00004.
- Myttenaere, C. 1972. “Absorption of Radiocaesium by Flooded Rice: Relative Importance of Roots and Shoot Base in the Transfer of Radioactivity.” Plant Soil 36: 215–218. doi:10.1007/BF01373473.
- Nakanishi, T. M., N. I. Kobayashi, and K. Tanoi. 2013. “Radioactive Cesium Deposition on Rice, Wheat, Peach Tree and Soil after Nuclear Accident in Fukushima.” Journal of Radioanalytical and Nuclear Chemistry 296 (2): 985–989. doi:10.1007/s10967-012-2154-7.
- Nemoto, K., and J. Abe. 2013. “Radiocesium Absorption by Rice in Paddy Field Ecosystems.” In Agricultural Implications of the Fukushima Nuclear Accident, edited by T. M. Nakanishi, and K. Tanoi, 19–27. Tokyo: Springer . doi:10.1007/978-4-431-54328-2_3.
- Nobori, T., N. I. Kobayashi, K. Tanoi, and T. M. Nakanishi. 2014. “Effects of Potassium in Reducing the Radiocesium Translocation to Grain in Rice.” Soil Science and Plant Nutrition 60 (6): 772–781. doi:10.1080/00380768.2014.947617.
- Oughton, D. H., B. Salbu, G. Riise, H. Lien, G. Ostby, and A. Nøren. 1992. “Radionuclide Mobility and Bioavailability in Norwegian and Soviet Soils.” The Analyst 117 (3): 481–486. doi:10.1039/an9921700481.
- Saito, T., S. Ohkoshi, S. Fujimura, K. Iwabuchi, M. Saito, T. Nemoto, M. Sato, M. Sato, K. Yoshioka, and H. Tsukada. 2012. “Effect of Potassium Application on Root Uptake of Radiocesium in Rice.” In International Symposium on Environmental Mornitoring and Dose Estimation of Residents after Accident of TEPCO’s Fukushima Daiichi Nuclear Power Stations, S. Takahashi, H. Yamana, T. Takahashi, K. Takamiya, S. Fukutani, N. Sato, and M. Nakatani edited by. Kyoto, Japan: Shiran Hall, December 14.
- Shizuma, K., Y. Fujikawa, M. Kurihara, and Y. Sakurai. 2018. “Identification and Temporal Decrease of 137Cs and 134Cs in Groundwater in Minami-Soma City following the Accident at the Fukushima Dai-ichi Nuclear Power Plant.” Environmental Pollution 234: 1–8. doi:10.1016/j.envpol.2017.11.018.
- Suzuki, Y., T. Yasutaka, S. Fujimura, T. Yabuki, M. Sato, K. Yoshioka, and K. Inubushi. 2015. “Effect of the Concentration of Radiocesium Dissolved in Irrigation Water on the Concentration of Radiocesium in Brown Rice.” Soil Science and Plant Nutrition 61 (2): 191–199. doi:10.1080/00380768.2014.1003192.
- Tagami, K., H. Tsukada, S. Uchida, and B. J. Howard. 2018. “Changes in the Soil to Brown Rice Concentration Ratio of Radiocaesium before and after the Fukushima Daiichi Nuclear Power Plant Accident in 2011.” Environmental Science & Technology 52 (15): 8339–8345. doi:10.1021/acs.est.8b01745.
- Tanaka, K., H. Iwatani, Y. Takahashi, A. Sakaguchi, K. Yoshimura, and Y. Onda. 2013. “Investigation of Spatial Distribution of Radiocesium in a Paddy Field as a Potential Sink.” Plos One 8 (11): e80794. doi:10.1371/journal.pone.0080794.
- Tsukada, H., A. Takeda, S. Hisamatsu, and J. Inaba. 2008. “Concentration and Specific Activity of Fallout 137Cs in Extracted and Particle-size Fractions of Cultivated Soils.” Journal of Environmental Radioactivity 99 (6): 875–881. doi:10.1016/j.jenvrad.2007.11.014.
- Tsukada, H., H. Hasegawa, S. Hisamatsu, and S. Yamasaki. 2002a. “Transfer of 137Cs and Stable Cs from Paddy Soil to Polished Rice in Aomori, Japan.” Journal of Environmental Radioactivity 59 (3): 351–363. doi:10.1016/S0265-931X(01)00083-2.
- Tsukada, H., and K. Ohse. 2016. “Concentration of Radiocaesium in Rice and Irrigation Water, and Soil Management Practices in Oguni, Date, Fukushima. Integr.” Environmental Assessment and Management 12 (4): 659–661. doi:10.1002/ieam.1827.
- Tsukada, H., S. H. Hasegawa, and S. Yamasaki. 2002b. “Rice Uptake and Distributions of Radioactive 137Cs, Stable 133Cs and K from Soil.” Environmental Pollution 117 (3): 403–409. doi:10.1016/S0269-7491(01)00199-3.
- Tsukada, H., S. Nihira, T. Watanabe, and S. Takeda. 2017. “The 137Cs Activity Concentration of Suspended and Dissolved Fractions in Irrigation Waters Collected from the 80 km Zone around TEPCO’s Fukushima Daiichi Nuclear Power Station.” Journal of Environmental Radioactivity 178–179: 354–359. doi:10.1016/j.jenvrad.2017.08.002.
- Tsukada, H. 2014. “Behavior of Radioactive Cesium in Soil with Aging.” Soil Science and Plant Nutrition 85 (2): 77–79. Japanese.
- Uchida, S., H. Takeda, K. Tagami, T. Takahashi, N. Ogiu, and T. Aono. 2007. “Element Concentrations in Japanese Rivers 2002-2006.” 4–19. ISBN 978-4-938987-42-8.
- Uchida, S., and K. Tagami. 2007. “Soil-to-plant Transfer Factors of Fallout 137Cs and Native 133Cs in Various Crops Collected in Japan.” Journal of Radioanalytical and Nuclear Chemistry 273 (1): 205–210. doi:10.1007/s10967-007-0737-5.
- Uchida, S., K. Tagami, I. Hirai, and M. Komamura. 2005. “Transfer Factors of Radionuclides and Stable Elements from Soil to Rice and Wheat.” Radioprotection 40: S129–S134 . doi:10.1051/radiopro:2005s1-021.
- Uematsu, S., H. Vandenhove, L. Sweeck, M. V. Hees, J. Wannijn, and E. Smolders. 2017. “Foliar Uptake of Radiocaesium from Irrigation Water by Paddy Rice (Oryza sativa): An Overlooked Pathway in Contaminated Environments.” New Phytologist 214 (2): 820–829. doi:10.1111/nph.14416.
- Wakabayashi, S., S. Itoh, and S. Takahashi. 2017. “Influence of Flooding on Exchangeability and Release of Stable and Radioactive Cesium in Contaminated Paddy Soil.” Soil Science and Plant Nutrition 63 (2): 110–118. doi:10.1080/00380768.2017.1294466.
- Wampler, J. M., E. J. Krogstad, W. C. Elliott, B. Kahn, and D. Kaplan. 2012. “Long-term Selective Retention of Natural Cs and Rb by Highly Weathered Coastal Plain Soils.” Environmental Science & Technology 46 (7): 3837–3843. doi:10.1021/es2035834.
- Yamaguchi, N., H. Tsukada, K. Kohyama, Y. Takata, A. Takeda, S. Isono, and I. Taniyama. 2017. “Radiocesium Interception Potential of Agricultural Soils in Northeast Japan.” Soil Science and Plant Nutrition 63 (2): 119–126. doi:10.1080/00380768.2017.1294467.
- Yamaguchi, N., I. Taniyama, T. Kimura, K. Yoshioka, and M. Saito. 2016. “Contamination of Agricultural Products and Soils with Radiocesium Derived from the Accident at TEPCO Fukushima Daiichi Nuclear Power Station: Monitoring, Case Studies and Countermeasures.” Soil Science and Plant Nutrition 62 (3): 303–314. doi:10.1080/00380768.2016.1196119.
- Yang, B., Y. Onda, Y. Wakiyama, K. Yoshimura, H. Sekimoto, and Y. Ha. 2016. “Temporal Changes of Radiocesium in Irrigated Paddy Fields and Its Accumulation in Rice Plants in Fukushima.” Environmental Pollution 208: 562–570. doi:10.1016/j.envpol.2015.10.030.
- Yoshikawa, N., H. Obara, M. Ogasa, S. Miyazu, N. Harada, and M. Nonaka. 2014. “137Cs in Irrigation Water and Its Effect on Paddy Fields in Japan after the Fukushima Nuclear Accident.” Science of the Total Environment 481: 252–259. doi:10.1016/j.scitotenv.2014.01.129.
- Yoshikawa, N., K. Nakashima, Y. Suzuki, S. Miyazu, K. Suzuki, N. Nogawa, and N. Harada. 2020. “Influence of Irrigation Water Intake on Local Increase of Radiocesium Activity Concentration in Rice Plants near a Water Inlet.” Journal of Environmental Radioactivity 225: 106441. doi:10.1016/j.jenvrad.2020.106441.