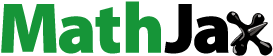
ABSTRACT
Applying soil amendments such as biochar has been proposed as a means to improve soil properties and reduce greenhouse gas emission from agricultural soils. The objective of this study was to evaluate and compare the effects of biochar and straw application on rice yield, select soil properties, global warming potential (GWP), and greenhouse gas intensity (GHGI) over the 3-year period. The study treatments included control (CN), barley straw biochar (BC, 2,000 kg ha−1), barley straw (BS, 2,000 kg ha−1), and BC+BS (each 1,000 kg ha−1). During the rice-growing season, significant interactive effects of BC and BS treatment were observed in major soil properties. The average rice yields in BC, BS, and BC+BS treatments increased by 4.6%, 4.7%, and 18.7%, compared with CN treatment, respectively. Also, BC+BS treatment produced relatively stable yield during the 3-year study. Both BC and BS applications, either alone or in combination, lowered soil bulk density values compared to the CN treatment. Further, BC application produced a low total CH4 flux than other treatments. The total produced GWP over the 3 years occurred in the order: BS ≧ CN > BC+BS > BC treatment. These results clearly demonstrate the advantages of biochar and straw applications in improving rice yield and soil fertility while reducing GWP and GHGI.
1. Introduction
Global atmospheric concentration of greenhouse gases has increased markedly from pre-industrial levels (Qin et al. Citation2016; Qi et al. Citation2018). Researches are underway in multifold sectors to reduce greenhouse gas emissions and to adapt to climatic variability (Sun et al. Citation2018; Yang et al. Citation2019). Agriculture is a major source of three primary greenhouse gas [carbon dioxide (CO2), methane (CH4), and nitrous oxide (N2O)] emissions and thus anthropogenic radiative climate forcing (Acharya, Rasmussen, and Eriksen Citation2012). As such, climate-smart technologies, improved agricultural management, and conservation measures are necessary to lower greenhouse gas emissions.
The warming of climate systems is unequivocal; one principal cause being the anthropogenic emission of CO2, CH4, and N2O into atmosphere (Citation2007; Thangarajan et al. Citation2013). The greenhouse effect of CH4 and N2O is 25 and 298 times, respectively, to that of CO2, making them important greenhouse gases (Linquist et al. Citation2012; IPCC Citation2013; Wang et al. Citation2017). Paddy fields, which produce the most important food resource in many Asian countries (Ghimire et al. Citation2017), are particularly recognized as major sites of CH4 and N2O emission and must be managed to prevent further climate warming (Ghimire et al. Citation2017; Jeong et al. Citation2017).
CH4 emission from rice fields is affected by methanogenic bacteria, organic matter, and soil redox potential levels; methanogenesis varying with soil, climate, and management factors (Liu and Wu Citation2004; Liu et al. Citation2011). Particularly, CH4 fluxes depend on agricultural water and fertilizer management. In addition, the level of CH4 generated greatly depends on measurement time and area. N2O emissions, on the other hand, are primarily affected by N fertilizer – type (inorganic fertilizer, straw, compost, manure, etc.), placement, time, and application methods (single vs. split) and environmental factors such as soil moisture, temperature, and aeration (Bouwman, Boumans, and Batjes Citation2002; Ruser et al. Citation2006). In recent years, there has been surge in studies on methods to stabilize or reduce CH4 and N2O emissions from rice-based cropping systems but long-term studies are generally few (Zhang et al. Citation2014; Gutekunst, Vargas, and Seyfferth Citation2017; Liang et al. Citation2017; Zhou et al. Citation2018).
Rice continues to be one of the most important food crops and major staple food for over half of the global population including South Korea (Cheng Citation2020). It provides up to 50% dietary caloric supply and a source of income for Asia’s poor population (Muthayya et al., Citation2014). Despite Asia contributing about 90% of global rice production, there are considerable uncertainties on future growth in rice yield and water savings due to climatic change. In South Korea, with the introduction of rice tariffication law and diminishing rice prices, rice acreage is decreasing (Jeong et al. Citation2021). For example, rice production in South Korea in 2016 was 14.6% lower than that in 2009. Global rice production continues to face challenges from excessive use of inorganic fertilizers, strong summer rainfall, and intensive tillage practices. Such practices on cropland could significantly deteriorate in soil health and physical quality and increase CH4 and N2O emissions. Globally, rice fields contribute to 30% and 11% of agricultural CH4 and N2O emissions, respectively (Hussain et al. Citation2015). As such, improved management is necessary to enhance soil properties and crop yield and reduce greenhouse gas emission. While researchers globally have attempted to use amendments like straw, green manures, and compost to overcome environmental challenges and sustain productivity in the face of climate change, little to no research is available on the use of biochar in South Korea.
Biochar is a carbon-rich material obtained after pyrolyzing biomass under anaerobic conditions. Applying biochar to soil systems has been proposed as a ‘carbon negative strategy’ to reduce greenhouse gas emission (Lehmann Citation2007; Major Citation2010; Sriphirom et al. Citation2020). Kang et al. (Citation2021) highlighted biochar potential in reducing CH4 and N2O from paddy fields in South Korea. However, in a meta-analysis, He et al. (Citation2017) reported that biochar increased soil CO2 fluxes by 22.14%, reduced N2O fluxes by 30.92%, and had no effect on CH4. Decrease in N2O fluxes is often associated with increase in aeration, soil pH, and N immobilization. Similarly, decrease in CH4 may result from decrease in methanogenic archaea to methanotrophic proteobacteria ratio. Alternate wetting and drying water management has shown potential to reduce CH4 emission by 19% without significantly affecting rice yields (Sriphirom et al. Citation2020). Although biochar amendments have shown increased rice production and reduced greenhouse gas emissions in East and Southeast Asia, most of these available results are reported from short-term studies (Yagi et al. Citation2020). Indeed, the effects of biochar on GHG fluxes are highly uncertain and site-specific depending on soil properties, crop management, and biochar rate and properties (He et al., Citation2017). Over the last few years, BC has, however, gained much attention as a soil conditioner and an amendment (Blanco-canqui et al., Citation2020; Kul et al. Citation2021). Liu et al. (Citation2016) reported 4.4–10.6% increases in rice yield with biochar application compared with control. Biochar amendment may improve soil quality, fertilizer use efficiency, nutrient bioavailability, and crop productivity apart from environmental advantages like waste management (Blanco-Canqui Citation2017; Bohara et al. Citation2019; Blanco-canqui et al., 2020).
Barley straw could serve as an important biomass to ameliorate soil physical properties, but soils amended with barley straw may show significant increase in organic acids and decrease in nitrogen levels. This phenomenon occurs primarily due to decomposition of straw and partly due to co-occurring of the barley harvesting and rice transplantation period in the South Korean cropping systems (Kang et al. Citation2016). Hence, the application of biochar derived from barley straw could be among portfolio of options available for recycling of resources, environmental quality, and soil health in agricultural systems. Here, the objective of this study was to compare the effects of biochar and straw application on rice yield, soil properties, and fluxes of greenhouse gas during a 3-year period. The results from this study will be beneficial in developing sustainable strategies for improving soil and environmental quality and crop productivity in rice-based cropping systems.
2. Materials and methods
2.1. Raw materials
Here, soil was classified as sandy loam with a bulk density of 1.31 Mg m−3, porosity of 56.3%, pH of 5.87, and cation exchange capacity (CEC) of 6.64 cmolc kg−1. Barley Straw (BS) feedstock was collected from local farm in Gwangyang City, South Korea, and biochar was produced at the Sunchon National University. The organic matter (OM), TN, P2O5, and K2O contents of the BS feedstock were 93.2%, 0.27%, 0.11%, and 2.34%, respectively. The straw was placed in a covered stainless-steel container and subjected to pyrolysis in a furnace [DK-1015(E), STI tech, Gumi, Republic of Korea] under oxygen-limited conditions (Kang et al. Citation2016). N2 gas was used to purge the stainless-steel container to allow limited oxygen, and the temperature in the internal biomass chamber was ramped to 400°C at 3°C min−1. The temperature was maintained at this peak temperature for nearly 1 hour. The barley straw biochar (BC) obtained was ground and filtered through a 2 mm sieve for use in the field experiment. The pH, total nitrogen (TN), P2O5, K2O, and CEC of the BC were 7.72, 0.42%, 0.30%, 1.41%, and 21.7 cmolc kg−1, respectively. The C content in the BC was >70%.
2.2. Experimental design
The field experiment was carried out to evaluate and compare the effects of BC and BS applications on rice yield, soil properties, and GWP at Sepung-ri (34º 56ʹ 33ʺ N, 127º 33ʹ 56ʺ E), Gwangyang-eup, Gwangyang-si, Jeollanam-do, South Korea. This study is a continuation of study examining the effect of biochar and straw treatments by Kang et al. (Citation2021). The temperatures and precipitation recorded during the experimental period are illustrated in . The experimental area had a mild oceanic climate with mean annual temperature of 15.2ºC (range of 14.8 ~ 15.5ºC) and mean annual precipitation of 1212 mm (range of 824 ~ 1607 mm).
The field experiment was conducted from 2015 to 2017 and consisted of four treatments: BC (barley straw biochar 2,000 kg ha−1), BS (barley straw 2,000 kg ha−1), BC+BS (barley straw biochar 1,000 kg ha−1 + barley straw 1,000 kg ha−1, respectively), and an untreated control (CN) plot to separate each treatment. Each treatment area was 100 m2 (10 × 10 m) in size. The experiment was replicated 3 times, thus producing a total of 12 plots. BC, BS, or BC+BS were evenly spread in the respective treatments 14 days prior rice transplanting and incorporated to a depth of 15 cm. BC was applied once in 2015 only. However, BS was applied every year (). Although BC was applied only once, the effects on yield are expected to remain for several years (Dumortier et al. Citation2020). No inorganic fertilizers were used during rice cultivation. Rice plots were irrigated after transplantation, and drainage was performed 3 weeks before harvesting.
Table 1. Field management for rice cultivation.
2.3. Sampling and soil analysis
After crop harvesting in 2017, soil samples were collected from the near-surface layer (15 cm) using a soil auger. Randomized soil samples were obtained from each plot, and a total of 14 soil samples were collected from each treatment. Soils were air-dried and sieved through a 2 mm sieve. Subsequently, all soil analyses were performed using the methods described in NIAST (Citation2000). The soil pH and electrical conductivity (EC) were measured in a soil–water ratio of 1:5 after shaking the mixture for 30 min. Soil organic carbon (SOC) was measured using a total organic carbon analyzer (Shimadzu TOC-VCPN) (Bhattacharjya et al., Citation2016), whereas the total nitrogen (TN) and available P2O5 (Avail. P2O5) analyses were performed using the Kjeldahl and Lancaster methods, respectively. Soil exchangeable cations were extracted by 1N-NH4OAc. At the end of the experiment, soil bulk density was measured at random locations in each treatment using the core method (Grossman and Reinsch Citation2002).
2.4. Quantitative real-time PCR analyses
Approximately 1.0 g of soil from different treatment locations was used to extract total DNA using the FastDNA® SPIN Kit (MP-Biomedicals, U.S.A.). The extracted DNA was resuspended in 50 μL DES (DNase/Pyrogen-Free Water), and DNA concentration and quality were measured using an Optizen NanoQ spectrophotometer (Optizen, Korea). The total bacterial content was quantified using SYBR Green-based quantitative real-time PCR (qPCR). The primers used were 1114-F (5ʹ-CGGCAACGAGCGCAACCC-3ʹ) and 1275-R (5ʹ-CCATTGTAGCACGTGTGTAGCC-3ʹ) (Denman and McSweeney Citation2006). Quantitative PCR was performed in a 20 µL reaction mixture consisting of 10 µL of 2× QuantiSpeed SYBR mix (PhileKorea, Korea), 0.8 µL of each 10 µM primer, and 50 ng of template DNA using an Eco™ Real-Time PCR (Illumina, San Diego, CA, U.S.A.). The qPCR reactions were performed in triplicate under thermal cycler conditions of one cycle at 50°C for 2 min, and 95°C for 2 min, followed by 40 cycles at 95°C for 15 s, 60°C for 1 min and 72°C for 30 s.
2.5. Monitoring of methane (CH4) and nitrous oxide (N2O)
CH4 and N2O fluxes were measured using a static chamber with 0.02 m2 area and 0.01 m3 volume. Gas sampling was performed weekly between 1 and 2 p.m. local time. The chamber was inserted to a soil depth of 20 cm between rice plants. CH4 and N2O measurements were made at 0, 10, 20, and 30 min after chamber closure using a 25 mL gas-tight syringe and samples were instantly transferred into gas-evacuated 10 mL soda glass vials. Samples were simultaneously analyzed on a gas chromatograph (GC-2014, Shimadzu) equipped with a flame ionization detector (FID) and an electron capture detector (ECD) for CH4 and N2O measurements, respectively. In FID, we maintained temperatures at 55ºC for the column, 100ºC for the injector, and 230ºC for the detector, whereas temperatures were maintained at 50ºC for the column and 310ºC for the detector in the ECD. Carrier gases were used in the experiment, which included a gas mixture of argon and methane for CH4 and nitrogen gas for N2O. Both CH4 and N2O fluxes were estimated using the Rolston equation (Rolston Citation1986; Kang et al. Citation2016; Yun et al. Citation2022).
F = ρ × (V/A) × (Δc/Δt) × (273/T)
where F is either CH4 or N2O flux, ρ is the density of CH4 or N2O density, V is the chamber volume (m3), A is the chamber area (m2), Δc/Δt is the increase in the rate of gas concentration per 10 min (Δc is concentration difference before and after sample collection; Δt is sampling time) and T is 273 + mean temperature in the chamber (ºC).
The total CH4 and N2O fluxes for the entire rice cultivation period were computed using Kang et al. (Citation2016).
Total CH4 and N2O flux =
where n is the number of sampling intervals, Ri is the rate of CH4 or N2O emission from rice plots during the sampling interval, and Di is the number of days in the sampling interval
In addition, the effect of BC on GWP was estimated from the total CH4 and N2O fluxes (TF) over the rice cultivation period using the IPCC method (Citation2007) below. This approach has been utilized in many other studies (Kang et al. Citation2016):
Total GWP (g CO2 m−2) = 25 × TF – CH4+298 × TF – N2O
Rice yield (Y) and GWP were used to calculate Greenhouse Gas Intensity (GHGI) as described by Zhang et al. (Citation2012).
GHGI = GWP/Y
2.6. Statistical analysis
Data analyses were conducted using JMP statistical analysis software from SAS 14.2 (SAS Institute Inc.). The mean values were computed as an average of three replicates. Each mean value was subjected to one-way analysis of variance (ANOVA), and a comparison of the treatments was performed using Tukey’s test. Two-way ANOVA was used to determine the differences between years, treatment conditions, and their interaction on CH4 and N2O fluxes, GWP, and GHGI.
3. Results
3.1. Effect of biochar and straw application on rice yield
The rice yields during the 3-year study were significantly affected by treatment types (). Significant yield difference was particularly evident in Year 2017. Rice yields for CN, BC, BS, and BC+BS treatments ranged on average from 473 to 515, 497 to 532, 516 to 528, and 583 to 602 g m−2, respectively. Among the treatments, the BC+BS treatment produced the highest average rice yield. There was no significant difference in rice yield under BC+BS treatment within three different years. Under the BS treatment condition, rice yield tended to increase year by year, with the final yield (year 3) showing an increase of 2.4% from that in year 1. On the other hand, under the BC treatment condition, the rice yield tended to decrease gradually, and in 2017, the rice yield decreased by about 6.7% compared with Year 2015. The rice yield under BC treatment decreased in the third rice harvest cycle and was lower than that in the BS treatment. However, in the first and second rice harvest cycles, the rice yield under BC treatment was 3.22% and 2.41% higher than that of BS treatment. On an average, rice yield under combined treatment (i.e., BC+BS) was significantly higher than remaining single treatments. Average rice yield under BS, BC, and CN treatments largely remained similar.
Table 2. Rice yield (mean ± SD) under different biochar and straw treatments during the 3 years of study.
3.2. Effect of biochar and straw application on soil properties
shows concentration of select soil physicochemical properties under different treatments after the final rice harvesting. Briefly, soil bulk density ranged between 1.25 and 1.33 Mg m−3, pH between 5.78 and 5.96, EC between 0.18 and 0.20 dS m−1, SOC content between 9.04 and 10.4 g kg−1, TN content between 1.39 and 1.69 g kg−1, Avail. P2O5 content between 55.5 and 60.0 mg kg−1, and CEC between 6.25 and 6.80 cmolc kg−1. Soil bulk density and pH were improved in all BC, BS, BC +BS treatments compared with the CN treatment. The SOC and TN content after BC application increased by 0.56 and 0.08 g kg−1, respectively, compared to control. Further, SOC and TN content increased by 0.89–1.36 and 0.16–0.3 g kg−1 after BS and BC+BS application, respectively. The soil CEC values after BC, BS, and BC+BS treatment were 0.55, 0.37, and 0.49 cmolc kg−1 greater than under CN plot, respectively. The total bacteria 16S rRNA gene components under the BC, BS, and BC+BS treatments were similar but significantly higher than under the CN treatment ().
Figure 2. Quantification of total bacterial 16S rRNA gene components after final rice harvesting. Different letters indicate significant differences among treatments at a 5% probability level. Error bars represent standard deviations (n = 12).
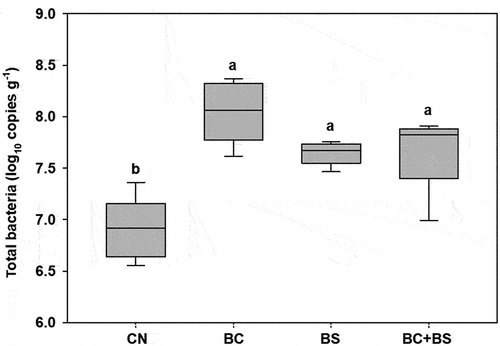
Table 3. Soil properties (mean ± SD) under different treatment sites after rice harvesting.
3.3. Effect of biochar and straw application on CH4 and N2O emission
The change in the CH4 emission rates during rice cultivation under different treatment conditions is shown in . Irrespective of the experimental years, the CH4 emission rate ranged from 3.31–42.10 mg m−2 hr−1 in CN, 1.32–28.01 mg m−2 hr−1 in BC, 0.56–44.90 mg m−2 hr−1 in BS, and 1.45 ~ 35.82 mg m−2 hr−1 in BC+BS treatment areas during the rice cultivation. CH4 emission patterns were similar in all treatments and years and peaked at 14–35 days after rice transplantation, gradually decreasing thereafter until rice harvesting. N2O emissions, on the other hand, varied greatly across treatments depending on the treatment conditions (irrigation and drainage) and sampling date (). It increased shortly after irrigation but decreased after drainage. The average rates of N2O emission were highest in 2016, with 73.3 µg m−2 hr−1 for CN, 93.5 µg m−2 hr−1 for BC, 155.8 µg m−2 hr−1 for BS, and 134.0 µg m−2 hr−1 for BC+BS. The N2O emission patterns were significantly different depending on the presence or absence of straw. Straw in general tended to increase both CH4 and N2O emission.
3.4. Effect of biochar and straw application on total flux
The total CH4 flux was significantly higher under straw-treated (BS and BC+BS) conditions. However, BC application produced a low total CH4 flux compared to that of the other treatments. In 2015, the CH4 flux of BC+BS and BC treatment areas was not significantly different, but in 2016 and 2017, the CH4 flux of the BC+BS treatment area increased by 24.8% and 16.2% over that of the BC treatment area. Meanwhile, total CH4 flux in the BC treatment area decreased by 2.9–40% compared to the CN area during the 3 year’s period ().
Table 4. Total CH4 and N2O fluxes, GWP, and GHGI (mean ± SD) during three rice-growing seasons.
The total N2O flux during the rice-growing period occurred in the order: BS ≥ BC+BS > BC > CN (). Similarly, the GWP over the 3 years occurred in the order: BS ≥ CN > BC+BS > BC treatment.
4. Discussion
Biochar and straw application to agricultural fields could potentially improve crop yield by improving soil properties; however, the efficacy may vary with extent, rate and type of biochar, pyrolysis conditions, soil, climate, and management practices (Burrell et al. Citation2016; Bonin et al. Citation2018). Yield effects under biochar are mostly apparent in nutrient-poor and marginal soils (Laird et al. Citation2017). In this study, we observed positive effects of the BC and BS application in select soil chemical properties such as pH, SOC, TN, and CEC after final rice harvesting in 2017. Also, a decrease in the soil bulk density was observed. Microbial activity appeared to be maintained at a higher level following biochar and straw application compared with the control treatment. Indeed, biochar could lower soil bulk density through either dilution effects or improved soil porosity. Biochar and straw application could increase exchangeable cations in soils by improving soil organic matter content and therefore surface area for cation adsorption (Blanco-Canqui Citation2017). BC treatment increased rice yield by 3.30–5.51% compared to control in each of the 3 years. The BS treatment, encompassing straw application every year, showed a steady increase in rice yield and produced a slightly different trend from that of the BC treatments. These observations agree with the report by Zhang et al. (Citation2012), Liu et al. (Citation2016) and Hu et al. (Citation2016) that showed significant positive effect of biochar and straw amendment on rice yield. Indeed, rice yield may differ with soil type, nutrient status, physical properties, climate, and management, with the straw application showing a significant positive effect.
Yield gains with BC and BS applications are governed by a number of mechanisms. Jeffery et al. (Citation2011) highlighted the benefits of improved water-holding capacity and liming effects in crop yields. Others have correlated yield with increase in water holding and plant available water (Zhou et al. Citation2019). Indeed, biochar application to soil improves soil bulk density, aeration, water retention, and SOC content and, therefore, soil health and productivity (Purakayastha, Kumari, and Pathak Citation2015; Fahad et al. Citation2016; Liu et al. Citation2016; Mohammadi et al. Citation2016). Straw, on the other hand, may have been mineralized during the 3-year study, resulting in an increase in nutrient use efficiency, and influencing rice yields (Thammasom et al. Citation2016; Zhang et al. Citation2017).
The application of BC+BS mixture appears to maintain stable rice production and soil quality. While BS can compensate for insufficient nutrients in BC, BC will retain nutrient elution by BS. Many previous studies reported higher nutrient availability to crops when BC was combined with additional nutrients in agricultural soils (Chan et al. Citation2007; Agegnehu, Nelson, and Bird Citation2016). Thus, the combination of BC and BS is likely to improve yield by potentially improving nutrient availability compared to sole application. We observed a lower GWP with BC application compared with other treatments, which is in par with several previous studies (Liu et al. Citation2014; Shen et al. Citation2014). Interestingly, BC was applied only once at the beginning of the study, but the GWP was significantly lower in all biochar-amended soils both alone or combined in all 3 years. On an average, BC had the lowest GWP compared with other treatments – In a global meta-analysis, Xu et al. (Citation2021) reported 27% reduction in GWP with biochar application. The effect of biochar on GWP is largely dependent on biochar’s CN ratio. An increase in biochar CN ratio is likely to decrease soil N mineralization intensity. Further, biochar increases cation exchange capacity through increased surface area for cation adsorption, thus adsorbs NH4+ (adsorbed on negatively charged COOH and OH groups) and NO3- (retained in intrapores of biochar or adsorbed by positively charged base functional groups) to reduce N2O emission (Xu et al. Citation2021)
GWP is highly dependent on CH4 emission in the paddy environment. The anaerobic decomposition of organic materials and the processes of nitrification and denitrification can affect CH4 and N2O emissions. Biochar may, however, increase N2O due to improved soil water content and thus denitrification. CH4 is likely to increase due to inhibition of soil methanotrophs (He et al., 2016) significant tradeoff between CH4 and N2O may exist with shifts from continuous flooding to midseason drainage management in rice paddies (Zou et al. Citation2005). Indeed, CH4 and N2O fluxes depend on soil texture, soil temperature, organic matter, organic amendments, soil microorganisms, and water management, among other factors (Minami Citation1994; Ruser et al. Citation2006; Sander, Samson, and Buresh Citation2014; Hu et al. Citation2016). In this study, the BS application acted as a source of decomposable carbon and nitrogen, increasing CH4 and N2O emissions and producing a relatively high GWP. Similar increase in GWP following straw application has been reported by other researchers. Thammasom et al. (Citation2016) reported higher CH4 emissions and GWP from straw application than those from biochar treatments. Zhang et al. (Citation2012) reported that straw application generates more CH4 emissions because it promotes methanogenesis with abundant methane-producing substrates. Also, compared to N2O emissions, CH4 emissions from rice fields have been reported to contribute significantly more to GWP increases (Cai, Shan, and Xu Citation2007; Bridgham et al. Citation2013; Pandey et al. Citation2014; Wang et al. Citation2018, Citation2019). We also observed consistently lower GHGI under BC+ BS treatment due largely to consistent rice yield and lower GWP. Because straw application (BS) produced a higher GWP than the other treatments, our results suggest on combining BS with biochar (BC+ BS) for reducing CH4 and N2O fluxes and GWP. However, long-term biochar studies under different soil amendments, nutrients, and water management are necessary to better understand GWP and minimize CH4 and N2O emissions in paddy fields.
5. Conclusion
This study comparing biochar and straw application effects on rice yield, select soil properties, and GWP indicates that BC and BS have potential to maintain or improve soil quality and rice yields, and lower greenhouse gas emission. However, BC alone resulted in inconsistent rice production, and BS-alone increased greenhouse gas emissions. Thus, our results suggest for a combination of a single BC treatment and annual BS supplementation to increase rice yield and lower GWP. Our results also suggest the benefits of using BC and BS as soil amendment to reduce dependency on inorganic fertilization and to improve agricultural sustainability.
Disclosure statement
No potential conflict of interest was reported by the author(s).
Additional information
Funding
References
- Acharya, B. S., J. Rasmussen, and J. Eriksen. 2012. “Grassland Carbon Sequestration and Emissions Following Cultivation in a Mixed Crop Rotation.” Agriculture, Ecosystems & Environment 153: 33–39. doi:10.1016/j.agee.2012.03.001.
- Agegnehu, G., P. N. Nelson, and M. I. Bird. 2016. “The Effects of Biochar, Compost, and Their Mixture and Nitrogen Fertilizer on Yield and Nitrogen Use Efficiency of Barley Grown on a Nitisol in the Highlands of Ethiopia.” The Science of the Total Environment 569-570: 869–879. doi:10.1016/j.scitotenv.2016.05.033.
- Bhattacharjya, S., R. Chandra, N. Pareek, and K. P. Raverkar. 2016. “Biochar and Crop Residue Application to Soil: Effect on Soil Biochemical Properties, Nutrient Availability and Yield of Rice (Oryza Sativa L.) and Wheat (Triticum Aestivum L.).” Archives of Agronomy and Soil Science 62 (8): 1095–1108. doi:10.1080/03650340.2015.1118760.
- Blanco‐canqui, H., D. A. Laird, E. A. Heaton, S. Rathke, and B. S. Acharya. “2020. Soil Carbon Increased by Twice the Amount of Biochar Carbon Applied After 6 Years: Field Evidence of Negative Priming.” GCB Bioenergy 12 (4): 240–251. doi:10.1111/gcbb.12665.
- Blanco-Canqui, H. 2017. “Biochar and Soil Physical Properties.” Soil Science Society of America Journal 81: 687–711. doi:10.2136/sssaj2017.01.0017.
- Bohara, H., S. Dodla, J. J. Wang, M. Darapuneni, B. S. Acharya, S. Magdi, and K. Pavuluri. 2019. “Influence of Poultry Litter and Biochar on Soil Water Dynamics and Nutrient Leaching from a Very Fine Sandy Loam Soil.” Soil and Tillage Research 189: 44–51. doi:10.1016/j.still.2019.01.001.
- Bonin, C. L., R. B. Fidel, C. Banik, D. A. Laird, R. Mitchell, and E. A. Heaton. 2018. “Perennial Biomass Crop Establishment, Community Characteristics, and Productivity in the Upper US Midwest: Effects of Cropping Systems Seed Mixtures and Biochar Applications.” European Journal of Agronomy 101: 121–128. doi:10.1016/j.eja.2018.08.009.
- Bouwman, A. F., L. J. M. Boumans, and N. H. Batjes. 2002. “Emissions of N2O and NO from Fertilized Fields: Summary of Available Measurement Data.” Global Biogeochemical Cycles 16: 1–13. doi:10.1029/2001GB001811.
- Bridgham, S. D., H. Cadillo-Quiroz, J. K. Keller, and Q. Zhuang. 2013. “Methane Emissions from Wetlands: Biogeochemical, Microbial, and Modeling Perspectives from Local to Global Scales.” Global Change Biology 19: 1325–1346. doi:10.1111/gcb.12131.
- Burrell, L. D., F. Zehetner, N. Rampazzo, B. Wimmer, and G. Soja. 2016. “Long-Term Effects of Biochar on Soil Physical Properties.” Geoderma 282: 96–102. doi:10.1016/j.geoderma.2016.07.019.
- Cai, Z. C., Y. H. Shan, and H. Xu. 2007. “Effects of Nitrogen Fertilization on CH4 Emissions from Rice Fields.” Soil Science and Plant Nutrition 53: 353–361. doi:10.1111/j.1747-0765.2007.00153.x.
- Chan, K. Y., L. V. Zwieten, I. Meszaros, A. Downie, and S. Joseph. 2007. “Agronomic Values of Green Waste Biochar as a Soil Amendment.” Australian Journal of Soil Research 45: 629–634. doi:10.1071/SR07109.
- Cheng, W. 2020. “Soil Carbon and Nitrogen Dynamics by Land Use and Management Changes in East and Southeast Asian Countries (Soil C and N by LUMC).” Soil Science and Plant Nutrition 66: 34–36. doi:10.1080/00380768.2020.1718923.
- Denman, S. E., and C. S. McSweeney. 2006. “Development of a Real-Time PCR Assay for Monitoring Anaerobic Fungal and Cellulolytic Bacterial Populations Within the Rumen.” FEMS Microbial Ecology 58: 572–582. doi:10.1111/j.1574-6941.2006.00190.x.
- Dumortier, J., H. Dokoohaki, A. Elobeid, D. J. Hayes, D. Laird, and F. E. Miguez. 2020. “Global Land-Use and Carbon Emission Implications from Biochar Application to Cropland in the United States.” Journal of Cleaner Production 258: 120684. doi:10.1016/j.jclepro.2020.120684.
- Fahad, S., S. Hussain, S. Saud, S. Hassan, M. Tanveer, M. Z. Ihsan, A. N. Shah, et al. 2016. “A Combined Application of Biochar and Phosphorus Alleviates Heat-Induced Adversities on Physiological, Agronomical and Quality Attributes of Rice.” Plant Physiology and Biochemistry 103: 191–198. doi:10.1016/j.plaphy.2016.03.001.
- Ghimire, R., S. Lamichhane, B. S. Acharya, P. Bista, and U. M. Sainju. 2017. “Tillage, Crop Residue, and Nutrient Management Effects on Soil Organic Carbon in Rice-Based Cropping Systems: A Review.” Journal of Integrative Agriculture 16: 1–15. doi:10.1016/S2095-3119(16)61337-0.
- Grossman, R. B., and T. G. Reinsch. 2002. “Bulk Density and Linear Extensibility.” In Methods of Soil Analysis: Part 4, SSSA Book Series 5, edited by J. H. Dane and G. C. Topp, 201–225. Madison, WI: SSSA.
- Gutekunst, M. Y., R. Vargas, and A. L. Seyfferth. 2017. “Impacts of Soil Incorporation of Pre-Incubated Silica-Rich Rice Residue on Soil Biogeochemistry and Greenhouse Gas Fluxes Under Flooding and Drying.” The Science of the Total Environment 593-594: 134–143. doi:10.1016/j.scitotenv.2017.03.097.
- He, Y., X. Zhou, L. Jiang, M. Li, Z. Du, G. Zhou, J. Shao, et al. 2017. “Effects of Biochar Application on Soil Greenhouse Gas Fluxes: A Meta‐analysis.” GCB Bioenergy 9 (4): 743–755. doi:10.1111/gcbb.12376.
- Hussain, S., S. Peng, S. Fahad, A. Khaliq, J. Huang, K. Cui, and L. Nie. 2015. “Rice Management Interventions to Mitigate Greenhouse Gas Emissions: A Review.” Environmental Science and Pollution Research 22: 3342–3360. doi:10.1007/s11356-014-3760-4.
- Hu, N., B. Wang, Z. Gu, B. Tao, Z. Zhang, S. Hu, L. Zhu, and Y. Meng. 2016. “Effects of Different Straw Returning Modes on Greenhouse Gas Emissions and Crop Yield in a Rice-Wheat Rotation System.” Agriculture, Ecosystems & Environment 233: 115–122. doi:10.1016/j.agee.2016.02.027.
- IPCC. 2007. Changes in Atmospheric Constituents and in Radiative Forcing. In Climate Change 2007: The Physical Science Basis. Contribution of Working Group I to the Fourth Assessment Report of the Intergovernmental Panel on Climate Change, edited by Solomon, S., D. Qin, M. Manning et al., Cambridge, UK: Cambridge University Press.
- IPCC. 2013. “Climate Change 2013: The Physical Science Basis.” In Contribution of Working Group I to the Fifth Assessment Report of the Intergovernmental Panel on Climate Change. Cambridge, United Kingdom and New York, USA: Cambridge University Press.
- Jeffery, S., F. G. Verheijen, M. van der Velde, and A. C. Bastos. 2011. “A Quantitative Review of the Effects of Biochar Application to Soils on Crop Productivity Using Meta-Analysis.” Agriculture, Ecosystems & Environment 144: 175–187. doi:10.1016/j.agee.2011.08.015.
- Jeong, S. T., G. W. Kim, H. Y. Hwang, P. J. Kim, and S. Y. Kim. 2017. “Beneficial Effect of Compost Utilization on Reducing Greenhouse Gas Emissions in a Rice Cultivation System Through the Overall Management Chain.” The Science of the Total Environment 115: 613–614. doi:10.1016/j.scitotenv.2017.09.001.
- Jeong, O. Y., H. S. Park, M. K. Baek, W. J. Kim, G. M. Lee, C. M. Lee, M. Bombay, M. B. Ancheta, and J. H. Lee. 2021. “Review of Rice in Korea: Current Status, Future Prospects, and Comparisons with Rice in Other Countries.” Journal of Crop Science and Biotechnology 24: 1–11. doi:10.1007/s12892-020-00053-6.
- Kang, S. W., D. C. Seo, Y. H. Cheong, J. W. Park, J. H. Park, H. W. Kang, K. Park, O. K. Ys, and J. S. Cho. 2016. “Effect of Barley Straw Biochar Application on Greenhouse Gas Emissions from Upland Soil for Chinese Cabbage Cultivation in Short-Term Laboratory Experiments.” Journal of Mountain Science 13 (4): 693–702. doi:10.1007/s11629-014-3428-z.
- Kang, S. W., J. J. Yun, J. H. Park, Y. H. Cheong, J. H. Park, D. C. Seo, and J. S. Cho. 2021. “Effects of Biochar and Barley Straw Application on the Rice Productivity and Greenhouse Gas Emissions of Paddy Field.” Applied Biological Chemistry 64 (1): 1–10. doi:10.1186/s13765-021-00666-7.
- Kul, R., T. Arjumend, M. Ekinci, E. Yildirim, M. Turan, and S. Argin. 2021. “Biochar as an Organic Soil Conditioner for Mitigating Salinity Stress in Tomato.” Soil Science and Plant Nutrition 67 (6): 693–706. doi:10.1080/00380768.2021.1998924.
- Laird, D. A., J. M. Novak, H. P. Collins, J. A. Ippolito, D. L. Karlen, R. D. Lentz, K. R. Sistani, K. Spokas, and R. S. Van Pelt. 2017. “Multi-Year and Multi-Location Soil Quality and Crop Biomass Yield Responses to Hardwood Fast Pyrolysis Biochar.” Geoderma 289: 46–53. doi:10.1016/j.geoderma.2016.11.025.
- Lehmann, J. 2007. “A Handful of Carbon.” Nature 447: 143–144. doi:10.1038/447143a.
- Liang, K., X. Zhong, N. Huang, R. M. Lampayan, Y. Liu, J. Pan, B. Peng, X. Hu, and Y. Fu. 2017. “Nitrogen Losses and Greenhouse Gas Emissions Under Different N and Water Management in a Subtropical Double-Season Rice Cropping System.” The Science of the Total Environment 609: 46–57. doi:10.1016/j.scitotenv.2017.07.118.
- Linquist, B., van Groenigen KJ, M. A. Adviento-Borbe, C. Pittelkow, van Kessel C, K. J. Groenigen, and C. Kessel. 2012. “An Agronomic Assessment of Greenhouse Gas Emissions from Major Cereal Crops.” Global Change Biology 18: 194–209. doi:10.1111/j.1365-2486.2011.02502.x.
- Liu, Y., H. Lu, S. Yang, and Y. Wang. 2016. “Impacts of Biochar Addition on Rice Yield and Soil Properties in a Cold Waterlogged Paddy for Two Crop Seasons.” Field Crops Research 191: 161–167. doi:10.1016/j.fcr.2016.03.003.
- Liu, J., J. Shen, Y. Li, Y. Su, T. Ge, D. L. Jones, and J. Wu. 2014. “Effects of Biochar Amendment on the Net Greenhouse Gas Emission and Greenhouse Gas Intensity in a Chinese Double Rice Cropping System.” European Journal of Soil Biology 65: 30–39. doi:10.1016/j.ejsobi.2014.09.001.
- Liu, C. W., and C. Y. Wu. 2004. “Evaluation of Methane Emissions from Taiwanese Paddies.” The Science of the Total Environment 333: 195–207. doi:10.1016/j.scitotenv.2004.05.013.
- Liu, Y., M. Yang, Y. Wu, H. Wang, Y. Chen, and W. Wu. 2011. “Reducing CH4 and CO2 Emissions from Waterlogged Paddy Soil with Biochar.” Journal of Soils and Sediments 11: 930–939. doi:10.1007/s11368-011-0376-x.
- Major, J. 2010. Guideline on Practical Aspects of Biochar Application to Field Soil in Various Soil Management Systems. International Biochar Initiative.
- Minami, K. 1994. “Methane from Rice Production.” Fertilizer Research 37: 167–179. doi:10.1007/BF00748935.
- Mohammadi, A., A. Cowie, T. L. A. Mai, R. Radl, P. Kristiansen, M. Brandão, and S. Joseph. 2016. “Biochar Use for Climate-Change Mitigation in Rice Cropping Systems.” Journal of Cleaner Production 116: 61–70. doi:10.1016/j.jclepro.2015.12.083.
- Muthayya, S., J. D. Sugimoto, S. Montgomery, and G. F. Maberly. 2014. “An Overview of Global Rice Production, Supply, Trade, and Consumption.” Annals of the New York Academy of Sciences 1324: 7–14. doi:10.1111/nyas.12540.
- NIAST. 2000. Methods of Soil and Plant Analysis. RDA, Suwon, Korea: National Institute of Agricultural Science and Technology.
- Pandey, A., V. T. Mai, D. Q. Vu, T. P. L. Bui, T. L. A. Mai, L. S. Jensen, and A. D. Neergaard. 2014. “Organic Matter and Water Management Strategies to Reduce Methane and Nitrous Oxide Emissions from Rice Paddies in Vietnam.” Agriculture, Ecosystems & Environment 196: 137–146. doi:10.1016/j.agee.2014.06.010.
- Purakayastha, T. J., S. Kumari, and H. Pathak. 2015. “Characterisation Stability, and Microbial Effects of Four Biochars Produced from Crop Residues.” Geoderma 239-240: 293–303. doi:10.1016/j.geoderma.2014.11.009.
- Qi, L., H. D. Niu, P. Zhou, R. J. Jia, and M. Gao. 2018. “Effects of Biochar on the Net Greenhouse Gas Emissions Under Continuous Flooding and Water-Saving Irrigation Conditions in Paddy Soils.” Sustainability 10: 1403. doi:10.3390/su10051403.
- Qin, X., Y. Li, H. Wang, C. Liu, J. Li, J. Wan, Q. Gao, F. Fan, and Y. Liao. 2016. “Long-Term Effect of Biochar Application on Yield-Scaled Greenhouse Gas Emissions in a Rice Paddy Cropping System: A Four-Year Case Study in South China.” The Science of the Total Environment 569-570: 1390–1401. doi:10.1016/j.scitotenv.2016.06.222.
- Rolston, D. E. 1986. Gas FluxMethod of soil analysis. Part 1. 2nd, edited by A. Klute, 1103–1119. Madison, WI: Agron. Monogr. 9. ASA and SSSA
- Ruser, R., H. Flessa, R. Russow, C. Schmidt, F. Buegger, and J. C. Munch. 2006. “Emission of N2O, N2, and CO2 from Soil Fertilized with Nitrate: Effect of Compaction, Soil Moisture and Rewetting.” Soil Biology & Biochemistry 38: 263–274. doi:10.1016/j.soilbio.2005.05.005.
- Sander, B. O., M. Samson, and R. J. Buresh. 2014. “Methane and Nitrous Oxide Emissions from Flooded Rice Fields as Affected by Water and Straw Management Between Rice Crops.” Geoderma 235-236: 355–362. doi:10.1016/j.geoderma.2014.07.020.
- Shen, J., H. Tang, J. Liu, C. Wang, Y. Li, T. Ge, D. L. Jones, and J. Wu. 2014. “Contrasting Effects of Straw and Straw-Derived Biochar Amendments on Greenhouse Gas Emissions Within Double Rice Cropping Systems.” Agriculture, Ecosystems & Environment 188: 264–274. doi:10.1016/j.agee.2014.03.002.
- Sriphirom, P., A. Chidthaisong, K. Yagi, S. Tripetchkul, and S. Towprayoon. 2020. “Evaluation of Biochar Applications Combined with Alternate Wetting and Drying (AWD) Water Management in Rice Field as a Methane Mitigation Option for farmers’ Adoption.” Soil Science and Plant Nutrition 66 (1): 235–246. doi:10.1080/00380768.2019.1706431.
- Sun, H., Y. Feng, Y. Ji, W. Shi, L. Yang, and B. Xiang. 2018. “N2O and CH4 Emissions from N-Fertilized Rice Paddy Soil Can Be Mitigated by Wood Vinegar Application at an Appropriate Rate.” Atmospheric Environment 185: 153–158. doi:10.1016/j.atmosenv.2018.05.015.
- Thammasom, N., P. Vityakon, P. Lawongsa, and P. Saenjan. 2016. “Biochar and Rice Straw Have Different Effects on Soil Productivity, Greenhouse Gas Emission and Carbon Sequestration in Northeast Thailand Paddy Soil.” Agriculture and Natural Resources 50: 192–198. doi:10.1016/j.anres.2016.01.003.
- Thangarajan, R., N. S. Bolan, G. Tian, R. Naidu, and A. Kunhikrishnan. 2013. “Role of Organic Amendment Application on Greenhouse Gas Emission from Soil.” The Science of the Total Environment 465: 72–96. doi:10.1016/j.scitotenv.2013.01.031.
- Wang, C., X. Li, Q. Min, W. Wang, J. Sardans, C. Zeng, C. Tong, and J. Peñuelas. 2019. “Responses of Greenhouse-Gas Emissions to Land-Use Change from Rice to Jasmine Production in Subtropical China.” Atmospheric Environment 201: 391–401. doi:10.1016/j.atmosenv.2018.12.032.
- Wang, W., S. Neogi, D. Y. F. Lai, C. Zeng, C. Wang, and D. Zeng. 2017. “Effects of Industrial and Agricultural Waste Amendment on Soil Greenhouse Gas Production in a Paddy Field in Southeastern China.” Atmospheric Environment 164: 239–249. doi:10.1016/j.atmosenv.2017.05.052.
- Wang, C., W. Wang, J. Sardans, W. An, C. Zeng, A. A. Abid, and J. Peñuelas. 2018. “Effect of Simulated Acid Rain on CO2, CH4, and N2O Fluxes and Rice Productivity in a Subtropical Chinese Paddy Field.” Environmental Pollution 243: 1196–1205. doi:10.1016/j.envpol.2018.08.103.
- Xu, H., A. Cai, D. Wu, G. Liang, J. Xiao, M. Xu, G. Colinet, and W. Zhang. 2021. “Effects of Biochar Application on Crop Productivity, Soil Carbon Sequestration, and Global Warming Potential Controlled by Biochar C: N Ratio and Soil pH: A Global Meta-Analysis.” Soil and Tillage Research 213: 105125. doi:10.1016/j.still.2021.105125.
- Yagi, K., P. Sriphirom, N. Cha-Un, K. Fusuwankaya, A. Chidthaisong, B. Damen, and S. Towprayoon. 2020. “Potential and Promisingness of Technical Options for Mitigating Greenhouse Gas Emissions from Rice Cultivation in Southeast Asian Contries.” Soil Science and Plant Nutrition 66: 37–49. doi:10.1080/00380768.2019.1683890.
- Yang, S., Y. Xiao, X. Sun, J. Ding, Z. Jiang, and J. Xu. 2019. “Biochar Improved Rice Yield and Mitigated CH4 and N2O Emissions from Paddy Field Under Controlled Irrigation in the Taihu Lake Region of China.” Atmospheric Environment 200: 69–77. doi:10.1016/j.atmosenv.2018.12.003.
- Yun, J. J., J. H. Park, B. S. Acharya, J. H. Park, J. S. Cho, and S. W. Kang. 2022. “Production of Pelleted Biochar and Its Application as an Amendment in Paddy Condition for Reducing Methane Fluxes.” Agriculture 12 (4): 470. doi:10.3390/agriculture12040470.
- Zhang, A., R. Bian, G. Pan, L. Cui, Q. Hussain, L. Li, J. Zheng, et al. 2012. “Effects of Biochar Amendment on Soil Quality, Crop Yield and Greenhouse Gas Emission in a Rice Paddy: A Field Study of 2 Consecutive Rice Growing Cycles.” Field Crops Research 127: 153–160. doi:10.1016/j.fcr.2011.11.020.
- Zhang, J., X. Hang, S. M. Lamine, Y. Jiang, D. Afreh, H. Qian, X. Feng, et al. 2017. “Interactive Effects of Straw Incorporation and Tillage on Crop Yield and Greenhouse Gas Emissions in Double Rice Cropping System.” Agriculture, Ecosystems & Environment 250: 37–43. doi:10.1016/j.agee.2017.07.034.
- Zhang, Y., Z. Li, J. Feng, X. Zhang, Y. Jinag, J. Chen, M. Zhang, A. Deng, and W. Zhang. 2014. “Differences in CH4 and N2O Emissions Between Rice Nurseries in Chinese Major Rice Cropping Areas.” Atmospheric Environment 96: 220–228. doi:10.1016/j.atmosenv.2014.07.038.
- Zhang, X. Y., G. B. Zhang, Y. Ji, J. Ma, H. Xu, and Z. C. Cai. 2012. “Straw Application Altered CH4 Emission, Concentration and 13C-isotopic Signature of Dissolved CH4 in a Rice Field.” Pedosphere 22: 13–21. doi:10.1016/S1002-0160(11)60187-2.
- Zhou, H., H. Fang, Q. Zhang, Q. Wang, C. Chen, S. J. Mooney, X. Peng, and Z. Du. 2019. “Biochar Enhances Soil Hydraulic Function but Not Soil Aggregation in a Sandy Loam.” European Journal of Soil Science 70: 291–300. doi:10.1111/ejss.12732.
- Zhou, B., Y. Feng, Y. Wang, L. Yang, L. Xue, and B. Xing. 2018. “Impact of Hydrochar on Rice Paddy CH4 and N2O Emissions: A Comparative Study with Pyrochar.” Chemosphere 204: 474–482. doi:10.1016/j.chemosphere.2018.04.056.
- Zou, J., Y. Huang, J. Jiang, X. Zheng, and R. L. Sass. 2005. “A 3‐year Field Measurement of Methane and Nitrous Oxide Emissions from Rice Paddies in China: Effects of Water Regime, Crop Residue, and Fertilizer Application.” Global Biogeochemical Cycles 19 (2). doi:10.1029/2004GB002401.