ABSTRACT
The increasing use of digital technologies can provide significant benefits to the excavation and interpretative process in archaeology. Yet, despite major developments in the last two decades, digital recording can still be seen as part of the realm of tech savvy archaeologists, well-funded projects, or larger commercial units who can develop their own recording systems or deploy a pre-existing application. The latter are often expensive and can be technologically challenging to implement, and few of these focus on both context recording and feature drawing. In response, this paper presents an accessible and flexible low-cost DIY digital workflow developed by the Comparative Kingship Project, Scotland, allowing field practitioners to benefit from some of the key advantages of “going digital” without the associated costs or complexities of some of the other systems.
Introduction
Archaeological excavation is a destructive process, making on-site recording paramount for documenting archaeological features and allowing the preservation of a site by record (Barker Citation1993). Textual and photographic records, drawings, material culture, environmental remains, and soil samples often constitute the only remaining evidence of excavated sites (Barker Citation1993, 162). Given the quantity of data produced during the excavation process, post-excavation work can be laborious, with certain tasks, such as digitally entering paper records or digitizing on-site plan and section (profile) drawings, particularly time-consuming. This can have significant cost-related implications, either regarding the number of staff or time needed on-site, a problem especially pertinent in an era of greater budgetary constraints in archaeology (Hamilakis Citation2015; Sayer Citation2014; The British Academy Citation2016).
The process of recording excavations in archaeology has gradually begun to take advantage of some of the major developments in digital technology (we include here digital photography and portable devices such as tablets, drones, dGPS, etc.), which has seen the emergence of oriented recording methods and the notion of “paperless archaeology” (Ballsun-Stanton et al. Citation2018; Eve and Hunt Citation2008; Jackson, Motz, and Brown Citation2016; Motz Citation2016; Roosevelt et al. Citation2015; Uildriks Citation2016; Wallrodt Citation2016). It is beyond the scope of this paper to debate in detail on the pros and cons of digital recording, and the benefits of digitally recording excavations have been well reported in the literature, the most significant of which include: a greater efficiency in the overall recording process and greater data quality and more accurate illustrations, as well as improved intellectual engagement with the archaeology in the field as digital recording liberates us from necessary but time-consuming tedious tasks (Austin Citation2014; Ellis Citation2016, 66–67; Morgan and Wright Citation2018; Olson et al. Citation2013; Roosevelt et al. Citation2015). Critics of digital recording and proponents of “slow archaeology” in particular (Caraher Citation2016) have, on the other hand, questioned whether improved efficiency has resulted in a greater understanding of the excavated site or how digital recording may lead to the “de-skilling” of archaeologists or impede interpretative and reflective processes due to the use of “rigid databases” or digital technologies replacing the “craft” of traditional illustration (for a more in-depth discussion on this debate, see Caraher Citation2016, Citation2019; Ellis Citation2016). Just as Ellis (Citation2016, 69) before us, here we firmly believe that these concerns are more associated with “good archaeology” than with a particular type of recording system (i.e. paper-based vs. paperless).
However, besides the ubiquitous use of digital photography and survey equipment such as total station or dGPS, it still appears that a significant proportion of field practitioners have yet to adopt digital recording methods for excavation (Boyd et al. Citation2021, 62). Most still rely on paper records and hand drawings, though digital recording is gradually being integrated into the excavation process, either directly in the field on a mobile device or during the post-excavation phase (Morgan and Eve Citation2012, 526). The main reasons for this slow adoption seem to include a lack of awareness of existing digital recording solutions available for archaeological excavations, their capabilities and applicability, their adaptability, their cost, and the perceived or real complexity to implement them (Austin Citation2014, 14). It is rarely highlighted how relatively easy it can be to implement project-specific digital recording using off-the shelf software, basic equipment (such as a handheld camera or a basic drone), or existing digital capture equipment already available on most excavations (Bobowski Citation2012; Waagen Citation2019; Wilhelmson and Dell’Unto Citation2015). Likewise, the ease of use and reliability of new digital technologies such as photogrammetry and drones for plan and section drawings does not appear to be widely deployed. In general, there is a lack of published workflows and guidelines for implementing a low-cost, digital recording system, especially for non-IT specialist field practitioners. Digital recording still appears to be largely the domain of tech-savvy archaeologists or well-funded projects with significant IT support (Ballsun-Stanton et al. Citation2018; Morgan and Eve Citation2012). In the British commercial sector, the larger companies have now developed their own digital recording systems, but, with the exception of LP Archaeology (ARK), these systems are not destined for distribution nor have they been widely published.
Furthermore, the majority of published digital recording systems originate from major, well-funded excavation projects at sites of international importance in particular (usually favorable) climates with significant infrastructure in place and reasonably easy site access. Such a physical and financial setting is in stark contrast to many commercial and smaller-scale academic excavations, where inclement weather conditions, difficulties in accessing sites, short-term targeted excavations, and small excavation teams are the norm. This is particularly the case in Scotland and for the Comparative Kingship Project at the University of Aberdeen. It is in this setting that we developed a simple, low-cost, and flexible DIY digital recording workflow which we mainly use on small-scale excavations at remote sites. In this paper, we present a digital approach developed for the project team and discuss how some of the major benefits of digital recording can still be attained with a low-cost DIY system which in turn could be adopted (or at least some elements) by practitioners across the sector.
The Comparative Kingship Project
The Comparative Kingship project at the University of Aberdeen is examining the nature of the societies and social, ideological, and political frameworks that filled the chasm left by the demise of the Roman Empire in the 5th century a.d. The project is undertaking detailed study of three major polities—Pictland, Scotland; Dál Riata, Northern Ireland; and Munster, Ireland—and their development during the course of the first millennium a.d. There are major disparities in the dataset, with early medieval Ireland having tens of thousands of settlements from this period (O’Sullivan et al. Citation2008), whereas in Scotland, the sites are much fewer (Noble and O’Driscoll Citation2019). Hence, in Scotland, a major focus of the project has been undertaking numerous evaluative excavations to try and identify sites of this period along with larger open area excavations of previously identified early medieval power centers at sites such as Rhynie, Aberdeenshire (Noble et al. Citation2013, Citation2019).
Since late 2017, the project has investigated approximately 35 sites, including hillforts, promontory forts, ecclesiastic enclosures, cemeteries, and the location of in situ carved stone sites, with a further 15–20 sites to be investigated (). The sites are varied in geographical and topographical settings, many of which are difficult to access, such as Mither Tap hillfort (Site Number NJ62SE 1, CANMORE). From this broad field-based approach with multiple research interventions, and the apparent lack of viable options available elsewhere, came the need to develop a digital recording workflow that followed discipline standards and allowed quick, but efficient and detailed, field recording and reporting. The system had to be simple enough for the multiple student and community volunteers who are involved in our fieldwork initiatives, robust enough to withstand the vagaries of the Scottish climate, portable enough to carry equipment to difficult to access sites, and low-cost enough to achieve with limited budgets for IT and equipment.
What are the Options? Digital Recording Approaches for Archaeological Excavation
The main reasons for excavations “to go digital” often reside in the desire to ensure greater efficiency and consistency in the recording process and increase data quality, as well as reduce the time necessary to perform certain tasks in and out of the field (entering and managing on-site records, drawing of plans and sections, checking and exporting records, and producing excavation reports). This usually entails entering stratigraphic information and other site records directly into a database or application on a tablet in lieu of paper records (Cianciarulo and Guerra Citation2007; Fee, Pettegrew, and Caraher Citation2013; Motz Citation2016; Roosevelt et al. Citation2015; Uildriks Citation2016) and the use of digital technologies such as survey equipment, orthophotography, or photogrammetry for plan and section drawings (Berggren et al. Citation2015; Kimball Citation2016; Morgan and Wright Citation2018; Waagen Citation2019). Practitioners wishing to switch from an analog to a digital record have the choice between either adopting an existing solution or developing and implementing their own DIY solution.
Existing alternatives
Existing solutions are either bespoke using proprietary software or purpose-built applications (for discussion, see Austin Citation2014, 14–15; Sobotkova et al. Citation2016) and are often for large excavation projects. Their primary objective is to provide a digital platform for collecting stratigraphic information and managing site records. These solutions are effectively enhanced databases integrating features such as the photographic record, GIS capabilities, survey equipment for spatially recording features, or 3D visualization abilities (Taylor et al. Citation2018).
Bespoke solutions such as iDig (Uildriks Citation2016), KAP (Roosevelt et al. Citation2015), or Imilké (López et al. Citation2016) are built from off the shelf, often proprietary software such as Filemaker Pro, Microsoft Access, or ArcGIS and were developed and deployed for specific projects on large multi-season excavations that often have considerable funds and resources. Though innovative in terms of digital recording, these solutions were not originally intended for wider distribution, which often limits their use for other projects. They are also often complex and difficult to replicate without hiring IT specialists, which is often beyond the means of most archaeological projects (Austin Citation2014). For example, while iDig is freely downloadable, easy to install, and has the advantage of running on the iPad, its vocabulary and structure is specific to the Agora project for which it was developed, limiting its portability to other projects (Uildriks Citation2016), though Boyd and colleagues (Citation2021) have adapted iDig for their excavations at Dhaskalio, Greece. Similarly, while the authors of a system designed for the Kaymakçi Archaeological Project in Turkey encourage its adoption at other sites (Roosevelt et al. Citation2015, 329), its setup and implementation appear technologically challenging (Roosevelt et al. Citation2015, 328) and would only be accessible to institutions with significant resources (in terms of personnel and funds).
Applications purposely built for archaeological recording, with development involving archaeologists and an IT team, ensure the software is fit for purpose and guarantees support and maintenance (Sobotkova et al. Citation2016, 338). The advantage of such applications is that they are intended for distribution and developed for use on multiple excavations, as opposed to having been designed for a particular site, and are therefore more flexible and adaptable than bespoke systems. They are often built using web-based technologies such as the Archaeological Recording Kit (ARK), the Federated Archaeological Information Management System (FAIMS; Ballsun-Stanton et al. Citation2018), or OpenDig (Vincent, Kuester, and Levy Citation2014a, Citation2014b) and can be, to some extent, freely available to use under open-source licenses. However, knowledge of web technologies and access to a server is needed to install and customize files for specific project needs (Austin Citation2014, 15).
Another issue is the lack of dedicated long-term development and software support (Austin Citation2014; Roosevelt et al. Citation2015), something encountered for recording apps developed on Google-based software such as Fusion Tables (Cascalheira, Bicho, and Gonçalves Citation2017), which is no longer supported since 2019. Currently, only FAIMS (Ballsun-Stanton et al. Citation2018), ARK (Eve and Hunt Citation2008), and Intrasis (https://www.intrasis.com), a GIS-based recording system (Williams and Brown Citation2017), seemingly provide continuous support. Solutions such as ARK and FAIMS operate on an open-source business model where users pay for installation and/or customization and support costs, providing the funds for continued support, whereas Intransis is a licensed program. The costs for ARK and FAIMS can vary depending on the needs for customization and support, but at a minimum, it is several hundred British Pounds per season (Sobotkova et al. Citation2016, 345–346). Though these costs are likely to decrease as the number of users increases (Sobotkova et al. Citation2016, 348), they are still usually beyond the means of less well-funded archaeological projects whose spending priorities may lie elsewhere.
The majority of existing digital recording systems have been developed as all-in-one packages that amalgamate site records and some planning or drawing capabilities and sometimes include integration with survey equipment and 3D visualization tools. These programs were primarily designed for large-scale ongoing excavations with complex stratigraphy and large artifact assemblages and have been particularly effective in visualizing and managing large sets of excavation data. They can also incorporate real-time or scheduled synchronization of data between devices via a central database hosted on a server, which can be beneficial for projects with large excavation teams, allowing all team members, including post-excavation specialists, access to excavation data in near real-time (Taylor et al. Citation2018).
However, the necessity of such applications, and their associated costs, is questionable for smaller-scale excavations with limited funds. This is particularly true for relatively non-complex sites with shallow stratigraphy, such as in rural environments in Scotland, which can be limited to shallow deposits dominated by cut features such as ditches, postholes, or pits. This is in stark contrast to the internationally recognized and deeply stratified sites such as Pompeii or Çatalhöyük for which most of these programs were developed.
Digital recording of excavations now extends far beyond centralized databases and can include an array of exciting low-cost possibilities for other fundamental tasks, such as planning and section drawing (Morgan and Wright Citation2018). Therefore, in order to benefit from digital recording, practitioners may prioritize the acquisition of equipment (such as drones or tablets) or photogrammetry software rather than bear the cost of implementing an all-in-one recording package. For the Comparative Kingship Project, the systems noted above were too elaborate and costly for the needs of the project and the nature of the excavations. Our motivation here was the belief that it was possible to benefit from “going digital” while adopting a “simple” low-cost DIY digital recording workflow.
DIY approaches
There has been an increasing number of alternatives to purposely-built programs for digitally recording excavations, many of which consider the funding and technological limitations that can face projects (Perfetti, Fassi, and Rossi Citation2020). These have experimented with, and successfully developed, methodologies and tools to digitally undertake some of the key steps of the recording process. Even in its simplest form, entering site records directly into a database is already an improvement on analog recording, with greater data consistency during input, alleviation from cumbersome paper records, and an increase in efficiency during post-excavation, as the records already exist in digital format. Several well-known database software, such as Filemaker Pro, Microsoft Access, or LibreOffice Base, can easily be used to generate data entry forms. Filemaker Pro has been particularly popular among archaeologists developing recording apps suited to the need of their projects (Jackson, Motz, and Brown Citation2016), as it is particularly well suited for the iPad environment and can also be used on PC tablets. Web-based options are also available, which have the advantage of being accessed via a web-browser and therefore are not constrained by a particular operating system. For instance, Zoho and Open Data Kit have both been successfully used by archaeologists to generate field recording forms for use on tablets (Austin Citation2014; Bobowski Citation2012).
Drawings are a critical component of recording, usually forming the only scaled visual record of excavated features which are inevitably destroyed by the excavation process (Morgan and Wright Citation2018). Site plans provide the location and character of features and allow the site to be “reconstructed” once excavation has been completed, while section drawings are a valuable aid to understanding stratigraphic sequences. Just as the use of digital photography is ubiquitous on most archaeological excavations, the use of digital survey equipment (Total Station or DGPS) is relatively common for recording the spatial location of features across a site and their broader outlines. However, when dealing with the detailed recording of a particular feature, measured plans and sections are necessary and are still largely done by hand (Morgan and Wright Citation2018, 1).
Several methods have been explored in order to utilize aerial photography to produce detailed images of an excavation to complement hand-produced field drawings or to be directly digitized to produce a scaled plan. Kites, balloons, and helikites, for example, have been used, and though they do have some advantages, they also have significant drawbacks (Verhoeven Citation2009). The use of georectified oblique photos have also been used to produce vertical images of sites which can then be digitized to produce a plan. Recent advances in technology have also seen the use of 3D laser-scanning to produce accurate representations of the archaeological record. However, the post-processing requires significant skill and is extremely time-consuming, while the weight, bulkiness, and high cost of such equipment limits the use of this technology (Kimball Citation2016, 10, 14).
The benefits of drawing directly from 2D orthophotographs and 3D models (Brandolini and Patrucco Citation2019; Kimball Citation2016) have been clearly demonstrated in terms of time gained in-field and accuracy and precision of the illustrations produced when compared to analog plan drawings. Recent developments in drone technology and photogrammetry appear well-suited to resolve some of the drawbacks of other methods used for capturing vertical imagery (Waagen Citation2019). Furthermore, most drones can now produce high-resolution imagery that is georeferenced via onboard GPS technology, making it an instrument of choice for planning purposes. While the absolute accuracy of non-professional drones can vary considerably and can sometimes be in the range of + or – 50 m or more (this can be corrected using a variety of techniques, like setting up Control Points manually or with a DGPS or georeferencing the collected data in GIS), the relative accuracy of the data is usually between 1 and 2 cm, which is more than accurate enough to produce a highly detailed and accurate model.
Drawing sections is probably the aspect of recording that has received the least “digital attention.” Traditionally, sections are drawn by hand on paper in the field and, once in the office, scanned and then digitized using a CAD software such as Inkscape, Illustrator, or Affinity Designer. However, drawing sections can be time consuming, sometimes subjective, and their accuracy is highly dependent on the illustrator's experience and abilities. Furthermore, drawing sections can be challenging in high winds or heavy rain, or in the case of wide and deep sections. Sections have also been reconstructed in open-plan excavations from the detailed recording of contexts in GIS software (such as ArcGIS or QGIS) using section tools allowing the vertical visualization of stratigraphic sequences (Losier, Pouliot, and Fortin Citation2007). Just as with plans, field practitioners have been experimenting with alternative methods to record sections. An initial approach has been to draw sections digitally on a tablet using drawing software such as iDraw instead of paper (Wallrodt Citation2016, 43). The advantage of this is to directly produce a vector drawing, removing the time needed to scan and digitize paper sections in post-excavation. However, it does not eliminate the time spent drawing in-field, nor does it reduce issues of accuracy. A far more promising approach has been the use of photogrammetry to capture excavated sections as 3D models from which section drawings can be generated during post-excavation, representing an improvement in time efficiency and accuracy over hand-drawn sections in the field (Boyd et al. Citation2021; De Reu et al. Citation2014; Kimball Citation2016).
As government agencies such as Historic Environment Scotland still require the production of vector-scaled section drawings in reports and archaeologists do not yet appear to be willing to abandon this type of output, considering the clear benefits of generating section drawings from 3D photogrammetry models of sections, efforts should be made to democratize the photogrammetry approach in order to allow more field practitioners to digitally enhance the production of sections in a simpler and cost-effective manner.
Photogrammetry: current applications and unused potential
Recent developments in photogrammetry have shown considerable potential to further streamline the in-field recording process. Terrestrial photogrammetry has been used by archaeologists since the 1980s (Fussell Citation1982; Georgiadis et al. Citation2000). However, the prohibitive expense of hardware and processing software, as well as concerns with the reliability of the results due to issues with keypoint identification (which are integral to create an accurate and dense three-dimensional model), meant that this was not a viable technique until quite recently (see Dellepiane et al. Citation2013; Doneus et al. Citation2011, 82; Jeffrey Citation2003, 112). The last ten years has seen a distinct shift in its use and application (Roosevelt et al. Citation2015, 327), as evidenced by a series of studies testing its accuracy and usefulness (Dellepiane et al. Citation2013; De Reu et al. Citation2013; Lerma and Muir Citation2014; Remondino Citation2014; Roosevelt et al. Citation2015, 327). Most of these focus on methodological work-flows (Dellepiane et al. Citation2013) or best practice case studies (Sapirstein and Murray Citation2017), though a few examples, such as Roosevelt and colleagues (Citation2015), McCarthy (Citation2014), De Reu and colleagues (Citation2014), O’Driscoll (Citation2018, Citation2019), or Boyd and colleagues (Citation2021), have highlighted the wider potential of this technique in excavation and surveying contexts. Dellepiane and colleagues (Citation2013) and Doneus and colleagues (Citation2011), for example, use photogrammetry to document each stratigraphic level in a trench but do not discuss its potential application in plan and section recording. Conversely, De Reu and colleagues (Citation2014) have successfully used this technique to record plans and sections, while also highlighting its applicability to record complex deposits such as human and animal remains.
Roosevelt and colleagues (Citation2015, 340) have shown that photogrammetry can reduce the average 0.08–0.1 m error margins of traditional drawings to 0.01–0.03 m. Such methods also improve standardization, consistency, and problems with subjectivity, particularly when applied to sites where a team of archaeologists may have varying levels of competency, though as Sapirstein and Murray (Citation2017, 340) have summarized, the greatest benefit is the significant reduction in time needed for in-field recording. Photogrammetry does also provide a more permanent record, with the original layers, spatial data, and appearance of a given feature able to be revisited more easily than a simple line drawing and photograph.
Of course, terrestrial photogrammetry is not without its problems. For example, in all of the published cases above, a hand-held DSLR was used for photo capture. While this allows quick and ad hoc capture of data, without additional time-consuming mapping of control points, the data can lack important detail, such as a scale. While Sapirstein and Murray (Citation2017, 341) dismiss drone-derived photogrammetry for excavation recording, as they see it as being too low resolution (which is a questionable point, considering the potential resolution achievable with modern drone cameras), we would argue that this is a viable solution and through particular techniques and processing can provide critical detail, including an accurate scale. In this regard, the additional implementation of drone-derived photogrammetry of an excavation site, integrated with hand-held derived data, provides an innovative and comprehensive approach to accurate and detailed recording of excavations.
Developing a Low-Cost DIY Digital Recording Approach
Designing the application for recording stratigraphic information
Four objectives were considered prior to the development of a digital recording application for the Comparative Kingship Project. First, to eliminate cumbersome paper records, binders, and other associated materials, replacing them with far more transportable tablets, a critical consideration given the remote location of some of the sites to be excavated during the project. Second, to ensure consistency among team members when filling in site records. Third, to eliminate the time-consuming task during post-excavation of entering data from paper records (including deciphering handwriting and manually verifying consistency) into a database or spreadsheet for the production of excavation reports. Finally, the design of digital site recording forms had to remain relatively simple so that hiring a programmer was unnecessary, staff time for development and deployment was kept to a minimum, and the resulting application was easy to use by staff, students, or volunteers and therefore would remain a close mimic of the traditional paper record (Austin Citation2014).
The application was developed using Filemaker Pro. The main reason behind the choice of software was the ability to easily deploy an application for iPad, already a device of choice among archaeologists (Ellis Citation2016; Fee, Pettegrew, and Caraher Citation2013) and available in our department. Filemaker operates on both Windows and Mac, and with Filemaker Pro Advanced, it is possible to create standalone versions, which is useful for distribution. However, we did not have the IT skills to develop a separate Android version. The example of OsteoSurvey (Austin Citation2014) has also highlighted the use of Open Data Kit as a promising open-source alternative to Filemaker. Filemaker Pro is not without issues on Mac computers, as previous versions are not supported by the developer, whose commercial strategy instead is to make new versions regularly available on the market. Furthermore, operating system upgrades may render Filemaker Pro unstable. There are now a number of alternatives for developing applications, and a comprehensive review addressing costs, implementation issues, and technological challenges or the IT skills required would be valuable for the archaeological community, though it is beyond the scope of this paper.
For ease of use and development, it was decided that a master database would not be created to store synchronized data from multiple devices on the same site. Given the relatively small teams on most of our excavations, the cost of Filemaker Server, necessary to implement synchronization, the technological challenges (Houk Citation2012, 80), and the necessary time expenditure (Austin Citation2014, 15), it was deemed unnecessary. The lack of synchronization was surmounted by assigning iPads to specific trenches in the case of larger excavations, in the same way that several context registers may be used for a large site. At the end of each excavation, the data was exported then merged in an Excel spreadsheet if multiple tablets were used for recording. Photographs taken with the iPad were also exported.
The recording application was developed based on the Museum of London Archaeology Service (MoLAS) recording protocols (London Archaeology Service Citation1994; Spence Citation1993) and is text-based, offering a digital equivalent of traditional paper records, integrating iPad camera capabilities easily achievable in Filemaker. While iPad camera resolutions are high enough for the photographic record, publication, and reporting purposes, we still take a DSLR camera on each site for photogrammetry purposes (see below), working shots, and as backup. Wherever possible, the forms designed use value lists and check boxes to reduce the amount of writing and ensure data consistency. Free note taking was also possible on most forms. Help prompts were implemented on the forms for training purposes, an approach that Bria (Bria and DeTore Citation2016) has demonstrated to be highly effective.
The database is site-based and structured around the concept of excavation “Areas” (i.e. trenches, test pits, or specific areas), which constitute the parent table to which all other records are related (). The main form is the “excavation area” where the different records are easily accessible via menu tabs such as “Contexts,” “Samples” (following Dobney et al. Citation1992), “Photos,” “Finds,” and “Drawings” (). Contexts (i.e. stratigraphic units) are visible as an editable list (see ) with specific forms for detailed recording (i.e. deposit/fill or cut; ). The other site records are entered in a list style form, which was preferred in terms of rapid overview and ease of data entry. The “Photo” register () logs entries for photos taken with a tablet, automatically imported into the database, or a DSLR camera or drone. The “Drawings” register can be used to log hand-drawn sketches, scaled site plans, and section drawings or digitally annotated photographs.
Figure 3. Excavation “Area” data page showing the “Context” list tab. Green numbers indicate the context data entry has been approved by a supervisor. The pencil button takes the user to the relevant recording form type (deposit/fill or cut).
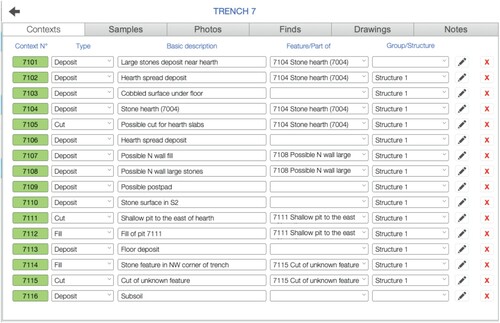
Integrating digital technologies into the drawing process
In our workflow (), some elements of hand drawing are retained, though the drawing process relies heavily on digital imagery. No hand-drawn plans are undertaken on-site, with this step of the recording process being abandoned altogether. Site plans and section drawings are produced after the excavation, back at the office, by digitizing a vertical image or a 3D photogrammetry model. The digitizing process is aided by an annotated hand-drawn sketch (on paper or tablet) or an annotated photograph on a tablet using a vector drawing software (). This step is done in the field and is an important element of the recording and interpretative processes as contexts and their stratigraphic relationships are identified.
Figure 7. Site plan generated from single vertical drone shot. A) Aerial photograph of Lower Citadel trench, Burghead; B) digitally annotated photograph during excavation on tablet; C) final site plan.
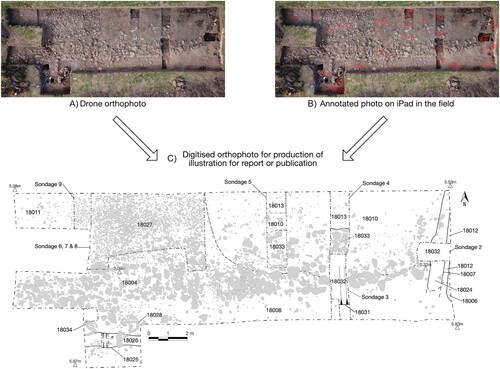
Depending on the context, there are a number of potential methodologies we can apply when creating plan drawings. The simplest is taking a vertical photograph using a drone (see Verhoeven Citation2009, 236–237), though for larger excavations, depending on the camera, this may lack quality and definition. In this regard, using a drone to create a photogrammetric three-dimensional model and orthomosaic of the excavated area provides more accuracy and also allows the user to extract accurate height data () (for a more comprehensive step by step guide to this process, see O’Driscoll Citation2018; Waagen Citation2019). We use a DJI Mavic Pro with 12 megapixel camera or DJI Phantom 4 Pro with 20 megapixel camera. Both are relatively light-weight and have built-in GPS (most low-cost drones have in-built GPS). This imprints each photograph with a geotag that enables the subsequent addition of a precise scale. However, accurate plans can be created with any type of camera drone, regardless of the presence of GPS or geotagged images. Instead, the core principle of any photogrammetric survey, either ground or drone based, is overlap of images. These overlapping images show features from slightly different positions, giving each photograph a unique angle of the selected area/object while also providing it with keypoints (reference points) visible on multiple photographs. An overlap of between 70 and 80 percent is needed to create an accurate three-dimensional model (O’Driscoll Citation2018). For larger landscape surveys using a drone, an automated flight-path created using freely available software, such as Drone Deploy or Pix4D Capture, is generally applied (for a more comprehensive step by step guide to using this software, see O’Driscoll Citation2018, 33). While this is a good way of ensuring consistent, evenly spaced photographs with sufficient overlap, it is not wholly necessary for planning an excavation trench. For the surveys described below, the drone was flown manually at a height of between 4 and 6 m, the height depending on the size of the trench and type of archaeology to be recorded. The height of the drone also determines the resolution of the model and number of data points per cubic meter. Once the drone has reached the desired height, the camera needs to be focused before image capture begins (on pre-planned flights, this is automated).
There are no special trench preparations needed for the survey, other than cleaning that would be undertaken for general context photographs. In some instances, where a context or deposit is poorly defined, we recommend lightly marking the outline with a trowel. Once the survey has started, it is good practice to keep the height consistent and photographs evenly spaced, though this is not a stringent requirement as long as there is enough photographic overlap.
There are some instances where flying a drone is not possible or necessary, such as in wet or windy conditions, in areas where flight restrictions occur, or simply where the size of an excavation trench is too small or narrow. While a 2 × 2 m trench could easily be surveyed with a drone, using a handheld digital camera offers more flexibility. In this instance, the same principles apply, with photographic overlap the main necessary component for a successful survey. Vertical photographs should be taken in a systematic manner using the same zig-zag traverse pattern employed by drone survey, making sure that the survey area incorporates at least 1 m of ground beyond the edge of the excavation trench. This allows the edges of the trench to have enough overlapping photographs to be included in the final three-dimensional model. When using a handheld camera, it is also good practice to take a series of oblique photographs from the edge of the excavation trench. Unlike most drone surveys, surveys employing handheld cameras will not automatically produce a scale (though the model will be proportionally correct) or orientate the resulting model. This has to be applied manually by incorporating a ranging-rod and north arrow into the surveyed area. Using a bubble-level to level the ranging-rod provides further accuracy. Conversely, the corners of the trench can be mapped with a Total Station or GPS and the photogrammetric model corrected in a GIS software such as ArcGIS or QGIS (for a more comprehensive step by step guide to employing corrections, i.e. “Ground Control Points,” see Waagen Citation2019, 14–17).
Regardless of the methodology employed (drone or handheld), the same techniques are used to process, visualize, and manipulate the data. Firstly, the photographs need to be processed. While this may seem like the most intimidating part of photogrammetry, nearly all modern software is automated (we use Pix4D Cloud; however, other software platforms, such as Autodesk ReMake or Agisoft Metashape, are also automated). Although the underlying algorithms and processes are complex and continually developing (O’Driscoll Citation2018, 33), all that is required of the user is to drag and drop the captured photographs into the program (this has been tested on Pix4D Cloud, Autodesk ReMake, and Agisoft Metashape). The software then knits the computed keypoints together to form an orthomosaic, DSM (Digital Surface Model), mesh, and point cloud, as well as producing a quality report that gives key information like the exact resolution of the model. We use Pix4D Cloud, which allows us to process our data online, without the need for high-spec computers. While this is a paid service, as is Agisoft Metashape, other freeware alternatives, such as Autodesk ReMake, exist, though these are not connected to the cloud and require longer processing times or better quality hardware for computation.
Once the data has been processed, we can download the output files and input into a GIS software to visualize and manipulate (while we use ArcGIS, this has also been tested in QGIS). Again, this software may seem like an impenetrable black box. However, all of the downloaded files can simply be dragged and dropped into the GIS, the most important of which are the orthomosaic and DSM. The orthomosaic provides a vertical, geo-rectified image with an accurate scale, and this can be used to create a trench plan by tracing contexts either in GIS or other illustration programs. We can also use the DSM to extract feature profiles, levels, and other height data. Profiles are created in GIS using the 3D Analyst tool (for ArcGIS), where the line of the profile is marked out with the Interpolate Line function, and then the Profile Graph function visualizes the selected height data. In the open-source GIS package QGIS, this can be done using the Profile Tool plugin. Levels can be taken by selecting any point with the Identify tool.
It is also possible to use these techniques on excavation sections (for a more comprehensive step by step guide to recording sections using photogrammetry, see De Reu et al. Citation2014). While it is possible to use photogrammetric modeling for simple and clearly defined stratigraphic sections, we advocate a more integrated approach for complex sequences, where a traditional paper record is taken of the layers and any detailed, time consuming features to draw, such as stone inclusions, are modeled. The position of the section is either located using GPS or Total Station survey or potentially a large, site wide photogrammetric drone survey (see O’Driscoll Citation2018). The section should be cleaned as normal, with the layers lightly marked with a trowel after general record photographs are taken. As above, a leveled ranging rod should be placed at the edge of the balk to allow the user to correctly level the section. The same processing methodology described above is applied here. In some instances, it might be necessary to delete data points which have been generated beyond the section face. This needs to be done manually. Usually, this requires the user to drag the point cloud file over the photogrammetry program window (we use Pix4DMapper, which is a companion program to Pix4DCloud), which loads the data. Then the user manually selects the unwanted data using a polygon and simply deletes this data. Once completed, the software will have to partially re-process the data to create an updated orthomosaic and DSM. In some cases, the resulting models will need to be displayed in a dedicated three-dimensional visualization program like SketchFab. Here again, this simply entails dragging the generated mesh file into the program, which automatically creates a textured model. The ranging rod (which has been leveled) and north arrow can be used to both scale and orientate the model. This can then be used to create a section by tracing contexts using an illustration program and combining them with the digitized section drawing.
The Comparative Kingship Project data is stored on the University of Aberdeen’s servers and, upon completion, the project’s digital archive will be stored with Historic Environment Scotland Archives Digital Repository (Historic Environment Scotland Citation2019).
The DIY Workflow Put into Practice: Excavations at Burghead and Other Sites from the Comparative Kingship Project
Our recent excavations at Burghead provide an excellent case study for the diverse applications of digital technologies and test for our low-cost DIY approach. Burghead, in northeastern Scotland, is one of the largest early medieval forts known in northern Britain (). Prior to its partial destruction in the early 19th century, it comprised three banks with external ditches cutting off the southeastern approach to a distinct promontory projecting into the southern edge of the Moray Firth (Oram Citation2007). The interior, which largely survives intact, is divided into an upper and lower citadel and was protected in the east, north, and west by a massive bank that survives in places to a height of over 6 m. Antiquarian excavations revealed incredibly well-preserved timber-lacing with charred in situ wooden beams. More recent sample excavation by the Archaeology Department at the University of Aberdeen has shown dense activity within the interior. The 2019 excavation season saw a variety of targeted and large-scale excavations of both the bank and internal areas in which our digital recording workflow was used and compared with more traditional forms of recording.
There were four major excavation areas at Burghead: T18 in the lower citadel and T11, T13, and T14 in the upper citadel. The trench supervisors of T18 and T14 each had an iPad, while the proximity and the small scale of T13 and T11 made it possible to share one device between the two trenches. One of the major advantages in the field was the portability of the devices versus having binders of paper records. The application only had two context recording forms: deposits/fills and cuts. The current deposit/fill forms, supplemented by the ability to enter text freely, were sufficient to collect the necessary information on ramparts and other contexts that may traditionally have required a separate or bespoke recording template. If there was a need for recording in more detail, such as stone finishing and coursing, this could be achieved by “revisiting” the feature by relying on a 3D photogrammetry model (Kimball Citation2016). The ability to enter text freely in a “notes” field proved to be particularly useful at the context level (for interpretations) but also at the trench level, where a “notes” field was sometimes used as a trench diary where the main observations, thoughts, and interpretations could be recorded on a daily basis.
At Burghead, the lack of syncing between devices was not an issue, despite the fact that several trenches were opened over two seasons. Should the necessity of using multiple devices in the same trench arise, careful coordination between excavators would be necessary, but feasible, just as it would be if using paper records, referring to a master list of context numbers. One of the other clear benefits of the digital recording workflow was the ability to take photos with the iPad, which were then automatically integrated into the database. Staff and students adapted rapidly to the use of digital records, and no specific training was required. The ability to call directly on the recording form help features, such as soil description charts or icons for cut description, proved popular and useful among students. Finally, the major benefit of creating even just a simple database like the one developed here was the ability to easily export all records as Excel spreadsheets as well as photographs. It is this aspect which proved to save the most time during post-excavation work, as well as generating consistent data.
The large and complex stone features revealed within T18 (, ) highlight the significant advantages of using our digital workflow. Even with regard to ongoing interpretations of a trench during an excavation, using a drone to simply visualize the overall plan can help clarify feature shape, orientation, etc., and in our experience, this can lead to the identification of features earlier than if we were assessing the trench from the ground alone. In terms of recording, a trench like this would traditionally be frame planned, as the density and complexity of the stones could not be adequately mapped with Total Station or GPS. In large trenches, this might be undertaken by a number of people and, in research contexts, by students of significantly differing levels of experience and competency. While every practitioner should have the ability to do these types of drawings, and students need time and training with these techniques, photogrammetry allows us to create a more accurate, consistent, and less subjective plan at a much quicker pace. This in turn allows students more time, and less pressure, to learn traditional techniques and, in the commercial sector, significantly cuts down the amount of time and resources needed in the recording phase of an excavation.
Figure 10. A) Comparison of orthophoto and photogrammetry model and B) comparison of photogrammetric models.
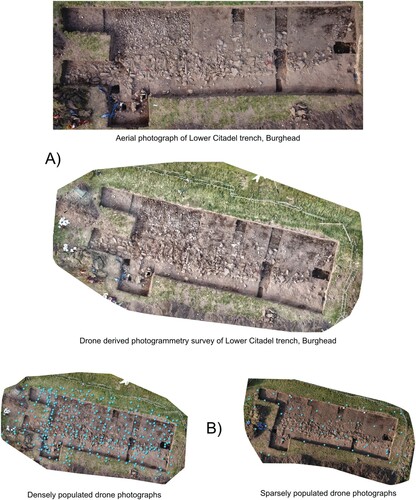
In this instance, a good quality vertical aerial photograph was used to plan the trench. Subsequently, both drone-derived and handheld photogrammetry was undertaken to compare the three methods (A). While there is no obvious difference in terms of the plans that are extracted, the photogrammetry techniques allow for a more extensive analysis, where feature profiles, levels, and other height-dependent data can be extracted and allows the original deposits to be more easily interrogated in the future through accurate photogrammetric models.
Two drone surveys were undertaken over the same area in order to compare the quality of models derived from a dataset with a low number of photographs and one with considerably more (B). Model 1 was created with 47 overlapping images taken at a height of approximately 6 m with a flight time of approximately 3 minutes. The flight-path of this model shows that exact and evenly spaced photographs are not necessary to create a good quality model, as long as there is sufficient overlap of images. A resolution/Ground Sample Distance of 104,339 points per meter cubed was attained using this data. Model 2 used 295 overlapping images taken from a similar height over 8 minutes to create a model with a resolution/Ground Sample Distance of 878,436 points per meter cubed. We might pursue such detail if we were attempting to model an artifact, for example, but in the present context, we see no advantage in this extra detail. In contrast, 648 photographs were taken with the handheld camera over 18 minutes, creating a model with a resolution/Ground Sample Distance of 1,847,572 points per meter cubed. While this is a good option in windy or wet weather, or for those who do not have access to a drone, with a trench of this size, there is more opportunity for user error (insufficient overlap being the most common mistake). We recommend, whenever possible, to only use a handheld camera for trenches less than 5 m wide.
In contrast, it took a relatively inexperienced student approximately 1 hour and 20 minutes to draw 2 m2 of the trench using a planning frame (it must be noted that the trench comprised a significant quantity of small to medium stone and cobbles, making plan drawing time consuming even for an experienced excavator). Extrapolating this, it would have taken this student approximately 66 hours to complete the entire trench. This is in addition to the time it would take to scan and digitize the resultant drawing. Of course, an experienced archaeologist could have recorded the trench much quicker, but even in this case, the methods described here saved significant quantities of time and produced better, more detailed records.
Excavation of the upper citadel rampart revealed an incredibly well-preserved wall face that survived to a height of nearly 3 m (A). Due to the depth of the trench and the need for substantial shoring, it proved difficult to complete an accurate section drawing. An experienced archaeologist set up and completed the section in approximately 3 hours and 22 minutes. The photogrammetric model was completed in approximately 7 minutes, with 260 overlapping images used to create an orthomosaic and DSM with a resolution/Ground Sample Distance of 189,538 points per meter cubed. Both the traditional hand drawing and photogrammetric model were digitized and compared in A. Immediately, we notice differences in terms of detail, not only regarding markings on the face of certain stones, but in the shape and outline. In most cases, the corner points of stones are measured when drawing by hand, and detail in shape can be missed. While such detail is not always necessary, there are certainly differences in the presence/absence of smaller stones. This may be a consequence of the subjectivity of the archaeologist drawing the section, who decides what to include in the drawing, which can be influenced by time and cost restraints, but also an archaeologists’ opinion of what is necessary to include. Many practitioners delimit an area of small cobbles or pebbles rather than drawing each stone individually, for example. Using photogrammetry substantially reduces this subjectivity, as there is no additional in-field time or costs associated with collecting this data. Using the three-dimensional models also allows users to reassess previous excavations without being influenced or biased by section drawings.
Figure 11. A) Comparison of hand-drawn vs. photogrammetry derived section drawings at Burghead. B) Section produced from combining photogrammetric model and hand-drawn outline of section at Balbinny, Angus.
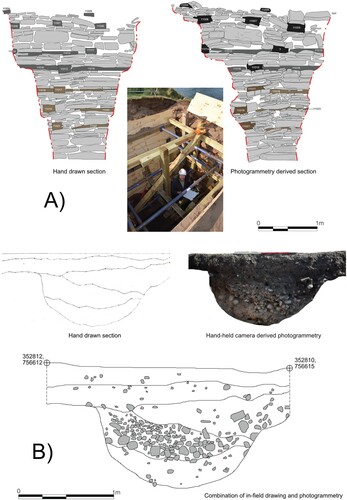
A more commonly encountered section was drawn at another Comparative Kingship site at Balbinny, Angus, Scotland (B). This leveled fort was discovered as a cropmark, and a section was excavated across its ditch on the eastern side in order to retrieve dating information on its construction and use. While it took a relatively inexperienced student approximately 47 minutes to set up and complete the drawing, no detail, such as the concentrated deposit of small rounded cobbles (deposit 104), was included. This is an excellent example of where digital and traditional recording techniques can be used in combination. In this instance, a photogrammetry model was used to add significant detail to the section drawing but also to check the quality of the students’ work.
Here again we stress the need for a hybrid approach, as in many instances, particularly where relying on compaction and composition as opposed to color, it is difficult to distinguish between contexts. At Mither Tap, Aberdeenshire, Scotland, for example, a deep balk was recorded using photogrammetry. While four contexts were identified in the field, it was impossible to distinguish between these in the photogrammetric model due to their similar color, composition, and inclusions. We attempted to lightly mark out these layers in-field using a trowel, which produced good results in particularly challenging weather conditions for drawing. Using such a method can also alleviate issues which arise when inaccuracies in hand drawn records lead to minor difficulties in combining traditional and digital methods. However, our approach may need to be adapted for very large sections with deep and complex stratigraphies, such as those encountered in urban settings.
We can also use digital recording in feature-specific contexts. T18 at Burghead revealed a series of evenly spaced post pads in the southwestern side of the excavated area. These stone features were used to support wooden posts in loose sand subsoils. While the broader trench level aerial photograph and photogrammetry surveys allow us to plan this feature and extract a profile, we could also use these methods for feature-specific contexts. Here, for example, 40 vertical and oblique photographs taken of one of these post pads with a handheld camera produced a model with a resolution/Ground Sample Distance of 189,472 points per meter cubed (A). A shows the dense point cloud, which can be generated from a feature-specific photogrammetric model, as well as some of the methods (hillshade and TIN models) which can be used to visualize the data. A model like this provides us with a comprehensive record of this feature, where we can extract an accurate plan or profiles from any orientation and view the feature in three dimensions. While a complete trench survey would provide similar results, creating feature specific models, particularly of important contexts, can be useful when other parts of the trench are not ready to be surveyed.
Figure 12. A) Photogrammetric models of a post pad at Burghead. B) Photogrammetry of the well at Tap O’Noth and generated profile.
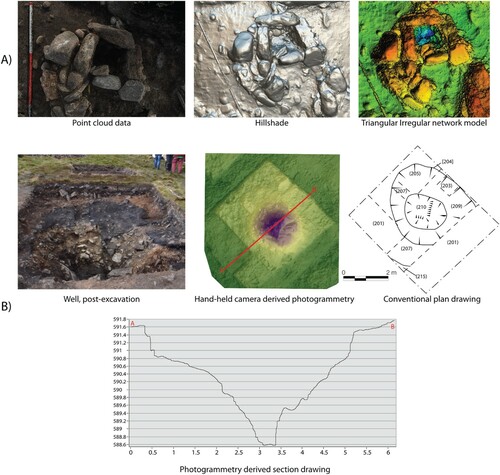
Photogrammetry can also mitigate health and safety issues. At our excavations at the Iron Age hillfort of Tap O’Noth in western Aberdeenshire, a rock-cut, partially clay-lined well was investigated within the interior. Instead of attempting to draw a profile using traditional methods, due to safety concerns, two photogrammetry surveys, one drone based and the other completed with a handheld camera, produced accurate three-dimensional models which were used to extract a profile and produce a plan (B).
Discussion
The purpose of this paper was to highlight the viability of a low-cost digital DIY recording system with minimal development required and the ease of applying and integrating these tools, either in part or as a complete suite, in the recording process. We feel there is value in making digital workflows more available to non-IT specialist field practitioners and demystifying digital recording. Though there is a gradual increase in the adoption of digital tools for excavation, the uptake still appears slow among the community, based on discussions with colleagues and other field practitioners. Published workflows and practical working examples can help the uptake of digital recording methods in archaeology.
The authors have significant excavation experience in both research and commercial contexts and had previously relied upon paper-based recording. It was observed that the adoption of the digital workflow, despite being simple, improved recording effectiveness and data quality (accuracy and consistency) and provided greater opportunities for on-site and post-excavation reflection and interpretation, as tedious and menial tasks were reduced or removed, resulting in a greater understanding of sites and the production of higher quality outputs (reports, publications, and illustrations).
The workflow could be particularly beneficial to small/medium commercial units or projects with limited IT or financial resources and in projects where rapid recording and reporting is required. In our case, the development of a paperless record was particularly advantageous, as excavated sites were often located in remote areas (hilltops and remote rural) and in some cases with no vehicle access. At times, several sites were investigated in quick succession by undertaking short evaluations, where carrying binders, paper records, and site plans, including the necessary planning frames and supplementary equipment, would have been an extra burden on the necessary excavation and survey tools that were required on-site. A similar observation can be made for cameras, where we now just take one camera on-site primarily for photogrammetry purposes, as most excavation shots were taken with the tablet camera. At some smaller excavations, survey equipment was not taken on-site, with trench locations being recorded directly using the drone.
Considering the limited IT budget at our disposal, it was worthwhile to spread costs over all components of the workflow, such as the purchase of a drone and photogrammetry software, rather than concentrate our funds on an all-in-one existing recording package. Another advantage of developing a DIY workflow with multiple independent components was the possibility to use equipment available on most excavations and its greater flexibility to adjust in the light of new advances in digital recording practices. Contrary to integrated systems, it was not desirable and was technically too challenging to produce a single application or database that contained all the necessary functions for digital recording (Spigelman, Roberts, and Fehrenbach Citation2016, 416). The design of the recording app was the most time consuming and challenging aspect, and this would probably be the case for any given digital recording system. The features implemented will be limited to personal abilities and time available, including for self-teaching when necessary. Nevertheless, even a database in its simplest form is an improvement on paper context sheets, as the data is already in digital format (eliminating indecipherable hand-written records), is more consistent, and is easier and more convenient to back up (local copies and synchronization to cloud-based servers) than paper records, without limiting free note taking (Ellis Citation2016, 59–60). Furthermore, DGPS integration in the recording app was unnecessary for the type of excavations undertaken, considering DGPS data can be easily imported into GIS software, including open-source options, an important consideration for an accessible and low-cost digital workflow. Similarly, DSLR cameras and drone photos for photogrammetry were processed post-excavation in independent software and archived separately.
The most significant benefits to the in-field recording process we observed were the time and accuracy gained in producing site plans and sections. Elements of hand-drawing were retained in the form of sketches on paper, thus retaining the cognitive actions involved in paper sketching (see Morgan and Wright Citation2018, 11–12 for a review on structured sketching), to accompany orthophotos and photogrammetry plan and section models. This approach was preferred over directly annotating photographs on the tablet (which is a consideration for future improvements of our workflow), which can be technologically challenging (Waagen Citation2019, 18). Morgan and Eve (Citation2012, 12) have already argued the benefits of a hybrid methodology where archaeological craft is maintained and the recording system benefits from the introduction of digital technology, while maintaining elements of older traditions.
After adopting the digital workflow, we observed significant time savings in nearly all situations, which led to a better learning environment. We ensured that students continued receiving training in conventional methods, particularly drawing. More time could be spent with students, or volunteers, “at the trowel's edge,” thus helping them develop their excavation and interpretative skills, focusing on the identification and understanding of the stratigraphy. Less pressure was put on students to generate site plans and section drawings that would traditionally compose the site archive, with the responsibilities this entails. As more accurate and less time-consuming site plans and sections could be produced, students benefited from greater opportunities to hone their drawing skills and reflective engagement (Morgan and Wright Citation2018). Students were taught conventional drawing, albeit with fewer drawing hours than with the use of an analog recording system, and a greater emphasis could be placed on ensuring they were able to correctly identify the various contexts and understand the stratigraphic relationships being analyzed. This was done by asking students to “present their feature” in an informal manner to their trench supervisors. After completing at least one conventional drawing, students would then usually only draw scaled sketches (plans) or outlines (sections) to accompany drone shots or photogrammetry models. The completion of their illustration is then done during post-excavation using CAD software. Compared to using a conventional paper-based recording system, we found this hybrid approach more effective for teaching and training, resulting in an enriched experience for the students.
There has been a necessary reflective process within the discipline on the impact of digital recording on archaeological practices (Caraher Citation2016, Citation2019; Ellis Citation2016; Huggett Citation2015, Citation2017), and concerns have been raised among practitioners that digital paperless recording could lead to the deskilling of the field archaeologist and constrict the interpretative process, removing it from the field to the office (Caraher Citation2016, 435–436; Morgan and Wright Citation2018, 12). It has also been argued that neo-liberal pressures on academic and private excavations are forcing field archaeologists to neglect on-site training and interpretation (Caraher Citation2016). Digital recording should therefore be regarded as a solution rather than a problem, and developing guidelines and publishing easily implementable practical workflows, in the absence of a discipline-agreed system, should be a priority in order to make digital recording more available and perhaps help towards alleviating some of those pressures, particularly in the commercial sector (Spigelman, Roberts, and Fehrenbach Citation2016, 403). We found, as Roosevelt and colleagues (Citation2015, 339) and Ellis (Citation2016, 66–67), that the reduction or replacement of tedious time-consuming analog tasks by quicker, more accurate digital methods released extra time for reflection and interpretation of the stratigraphy in the trench. Based on our experience, digital recording did not result in de-skilling or lack of engagement with the archaeology, issues raised by the “slow” archaeology movement (see Caraher Citation2016, Citation2019; Ellis Citation2016). Quality recording and on-site intellectual reflection are also values shared with “good archaeology” (Ellis Citation2016, 69) to which digital recording can positively contribute. Could digital recording not also contribute to such movements, including “degrowth archaeology” (Flexner Citation2020), by potentially “slowing down” or “de-stressing” some aspects of the excavation process?
By removing tedious analog tasks (e.g. drawing countless stones), students and staff benefited from having more time available for intellectual engagement with the archaeology (analysis and interpretation of the stratigraphy) rather than spending those hours just recording it, increasing our interpretation and understanding of the site (see also Ellis Citation2016, 67). Not only do we continue to value training in conventional recording and excavation practices as an important formative element, these skills were deemed necessary to better integrate new digital tools. What appeared evident, however, is that more training in digital recording technologies (i.e. database design, drone technology, photogrammetry, etc.) at the undergraduate level will be required, and acquiring these new skill sets will be just as important as learning conventional recording methods. This will be an important step in students becoming digitally independent archaeologists in the future, avoiding technological elitism and poor practice (see Taylor et al. Citation2018), and contributing to the “distribution of digital know-how at relatively low-cost” (Roosevelt et al. Citation2015, 341) and the democratization of digital recording.
Conclusion
The aim of this paper is to demonstrate the ease and affordability of implementing digital recording on archaeological excavations using a DIY approach as an alternative to previously developed digital recording systems. Composed of independent elements, the workflow is flexible in terms of upgrades and improvement and can accommodate budgets of various sizes. The study also highlighted that even the incorporation of a relatively simple digital approach could be highly beneficial, due to the low expenditure in terms of development time and costs. This resulted in greater data quality and overall efficiency and generated further opportunities for reflective engagement and training on-site. Hiring an IT specialist or purchasing available packages may well be out of reach for most archaeological projects; thus, we believe low-cost and DIY approaches, despite their limitations, have an important role in improving archaeological excavation practice and can benefit all sectors involved in fieldwork, including community-led, academic, and commercial projects.
Geolocation Information
The paper’s study area is Scotland, United Kingdom and more particularly northeast mainland Scotland.
Supplemental Material
Download MP4 Video (77.5 MB)Acknowledgements
Edouard Masson-MacLean and James O’Driscoll contributed equally to this paper. We wish to thank three anonymous reviewers for their positive comments and constructive suggestions, which improved the original version of the paper. EMM also wishes to thank personally Dr. Joshua Wright (University of Aberdeen) for the informal but valuable and stimulating discussions which helped shape some of the original ideas for this paper. The Comparative Kingship project is funded by the Leverhulme Trust as part of a Research Leadership Award under Grant RL-2016-069. The original impetus for the digital recording was a Historic Environment Scotland grant RG14686 for work at Dunnicaer promontory fort developed by Cathy MacIver—this set the foundations for the workflow outlined above.
Additional information
Notes on contributors
Edouard Masson-MacLean
Edouard Masson-MacLean (Ph.D. 2018, University of Aberdeen, Scotland) is a research fellow at the University of Aberdeen. His research interests include archaeozoology, fieldwork practices and recording, Arctic archaeology, and animal husbandry in early medieval Scotland.
James O’Driscoll
James O’Driscoll (Ph.D. 2016, University College Cork, Ireland) is a research fellow at the University of Aberdeen. His research interests include remote sensing and GIS-based techniques in archaeology, prehistoric and early medieval archaeology in Europe, and landscape archaeology.
Cathy McIver
Cathy MacIver (MLitt. 2010, University of Glasgow) is a project manager at AOC Archaeology Group, a fellow of the Society of Antiquaries of Scotland, and a research affiliate of the University of Glasgow. Her research interests include prehistoric and early medieval Scotland, digital archaeology (databases and GIS), community archaeology, teaching, and training in fieldwork methods and techniques.
Gordon Noble
Gordon Noble (Ph. D. 2009, University of Reading) is a Professor at the University of Aberdeen. His research interests include the archaeology of Scotland from the Mesolithic to Medieval periods, early royal landscapes of Ireland and Scotland, fieldwork, landscape archaeology, and public engagement.
References
- Austin, A. 2014. “Mobilizing Archaeologists: Increasing the Quantity and Quality of Data Collected in the Field with Mobile Technology.” Advances in Archaeological Practice 2 (1): 13–23.
- Ballsun-Stanton, B., S. A. Ross, A. Sobotkova, and P. Crook. 2018. “FAIMS Mobile: Flexible, Open-Source Software for Field Research.” SoftwareX 7: 47–52.
- Barker, P. 1993. Techniques of Archaeological Excavation. London: Psychology Press.
- Berggren, Å, N. Dell’Unto, M. Forte, S. Haddow, I. Hodder, J. Issavi, N. Lercari, C. Mazzucato, A. Mickel, and J. S. Taylor. 2015. “Revisiting Reflexive Archaeology at Çatalhöyük: Integrating Digital and 3D Technologies at the Trowel’s Edge.” Antiquity 89 (344): 433–48.
- Bobowski, B. 2012. “Easy Recording System: Solutions Based on Web Free Apps Databases.” In Revive the Past. Computer Applications and Quantitative Methods in Archaeology (Caa). Proceedings of the 39th International Conference, Beijing, April 12-16, edited by M. Zhou, I. Romanowska, X. Wu, P. Xu, and P. Verhagen, 170–76. Pallas Publications: Amsterdam.
- Boyd, M. J., R. Campbell, R. C. P. Doonan, R. C. Doonan, C. Douglas, G. Gavalas, M. Gkouma, C. Halley, B. Hartzler, J. A. Herbst, H. R. Indgjerd, and A. Krijnen. 2021. “Open Area, Open Data: Advances in Reflexive Archaeological Practice.” Journal of Field Archaeology 46 (2): 62–80. https://doi.org/https://doi.org/10.1080/00934690.2020.1859780.
- Brandolini, F., and G. Patrucco. 2019. “Structure-From-Motion (Sfm) Photogrammetry as a Non-Invasive Methodology to Digitalize Historical Documents: A Highly Flexible and Low-Cost Approach.” Heritage 2 (3): 2124–36.
- Bria, R., and K. E. DeTore. 2016. “Enhancing Archaeological Data Collection and Student Learning With a Mobile Relational Database.” In Mobilizing the Past for a Digital Future: The Potential of Digital Archaeology, edited by E. W. Averett, J. M. Gordon, and D. B. Counts, 143–82. Grand Forks, ND: The Digital Press@ University of North Dakota.
- The British Academy. 2016. Reflections on Archaeology. https://www.thebritishacademy.ac.uk/documents/221/reflections-on-archaeology.pdf.
- Caraher, W. 2016. “Slow Archaeology: Technology, Efficiency, and Archaeological Work.” In Mobilizing the Past for a Digital Future: The Potential of Digital Archaeology, edited by E. W. Averett, J. M. Gordon, and D. B. Counts, 421–41. The Digital Press at the University of North Dakota.
- Caraher, W. 2019. “Slow Archaeology, Punk Archaeology, and the “Archaeology of Care”.” European Journal of Archaeology 22 (3): 372–85.
- Cascalheira, J., N. Bicho, and C. Gonçalves. 2017. “A Google-Based Freeware Solution for Archaeological Field Survey and Onsite Artifact Analysis.” Advances in Archaeological Practice 5 (4): 328–39.
- Cianciarulo, D., and F. Guerra. 2007. “Digital Recording in Archaeological Excavation Using Tablet Pc.” In Proceedings of the XXI International Symposium Cipa 2007 : AntiCIPAting the Future of the Cultural Past : Zappeion Megaron, Athens, Greece 01-06 October 2007, edited by A. Georgopoulos. Athenai: CIPA.
- De Reu, J., P. De Smedt, D. Herremans, M. Van Meirvenne, P. Laloo, and W. De Clercq. 2014. “On Introducing an Image-Based 3D Reconstruction Method in Archaeological Excavation Practice.” Journal of Archaeological Science 41: 251–62.
- De Reu, J., G. Plets, G. Verhoeven, P. De Smedt, M. Bats, B. Cherretté, W. De Maeyer, J. Deconynck, D. Herremans, P. Laloo, and M. Van Meirvenne. 2013. “Towards a Three-Dimensional Cost-Effective Registration of the Archaeological Heritage.” Journal of Archaeological Science 40 (2): 1108–21.
- Dellepiane, M., N. Dell’Unto, M. Callieri, S. Lindgren, and R. Scopigno. 2013. “Archeological Excavation Monitoring Using Dense Stereo Matching Techniques.” Journal of Cultural Heritage 14 (3): 201–10.
- Dobney, K., A. R. Hall, H. K. Kenward, and A. Milles. 1992. “A Working Classification of Sample Types for Environmental Archaeology.” Circaea 9 (1): 24–26.
- Doneus, M., G. Verhoeven, M. Fera, C. Briese, M. Kucera, and W. Neubauer. 2011. “From Deposit to Point Cloud – A Study of Low-Cost Computer Vision Approaches for the Straightforward Documentation of Archaeological Excavations.” Geoinformatics CTU FCE: Reviewed Papers from XXIIIrd International CIPA Symposium, Prague, Czech Republic, September 12-16, 2011 6: 81–88.
- Ellis, S. J. R. 2016. ““Are We Ready for New (Digital) Ways to Record Archaeological Fieldwork? A Case Study from Pompeii”.” In Mobilizing the Past for a Digital Future: The Potential of Digital Archaeology, edited by E. W. Averett, J. M. Gordon, and D. B. Counts, 51–75. Grand Forks, ND: The Digital Press @ The University of North Dakota.
- Eve, S., and G. Hunt. 2008. “ARK: A Developmental Framework for Archaeological Recording.” Proceedings of Computer Applications and Quantitative Methods 2007, Berlin, 2-6th April 2007.
- Fee, S. B., D. K. Pettegrew, and W. R. Caraher. 2013. “Taking Mobile Computing to the Field.” Near Eastern Archaeology 76 (1): 50–55.
- Flexner, J. L. 2020. “Degrowth and a Sustainable Future for Archaeology.” Archaeological Dialogues 27 (2): 159–71.
- Fussell, A. 1982. “Terrestrial Photogrammetry in Archaeology.” World Archaeology 14 (2): 157–72.
- Georgiadis, C., V. Tsioukas, L. Sechidis, E. Stylianidis, and P. Patias. 2000. “Fast and Accurate Documentation of Archaeological Sites Using in the Field Photogrammetric Techniques.” International Archives of Photogrammetry and Remote Sensing 33: 28–32.
- Hamilakis, Y. 2015. “Archaeology and the Logic of Capital: Pulling the Emergency Break.” International Journal of Historical Archaeology 19 (4): 721–35.
- Historic Environment Scotland. 2019. HES Archives: Digital Repository Management Policies. Historic Environment Scotland. https://www.historicenvironment.scot/archives-and-research/publications/publication/?publicationid=0eb12d93-32d0-4450-8ef7-ab7d00f85f54.
- Houk, B. A. 2012. “The Chan Chich Archaeological Project’s Digital Data Collection System.” In The 2012 Season of the Chan Chich Archaeological Project, edited by B. A. Houk, 73–82. Lubbock, Texas: Texas Tech University.
- Huggett, J. 2015. “Challenging Digital Archaeology.” Open Archaeology 1 (1).
- Huggett, J. 2017. “The Apparatus of Digital Archaeology.” Internet Archaeology 44.
- Jackson, S. E., C. F. Motz, and L. A. Brown. 2016. “Pushing the Paperless Envelope: Digital Recording and Innovative Ways of Seeing at a Classic Maya Site.” Advances in Archaeological Practice 4 (2): 176–91.
- Jeffrey, S. 2003. “Three Dimensional Modelling of Scottish Early Medieval Sculpted Stones.” PhD thesis, University of Glasgow.
- Kimball, J. J. L. 2016. 3D Delineation: A Modernisation of Drawing Methodology for Field Archaeology. Oxford: Archaeopress Publishing Ltd.
- Lerma, J. L., and C. Muir. 2014. “Evaluating the 3D Documentation of an Early Christian Upright Stone With Carvings from Scotland with Multiples Images.” Journal of Archaeological Science 46: 311–18.
- London Archaeology Service, Museum of. 1994. Archaeological Site Manual. London, UK: Museum of London.
- Losier, L.-M., J. Pouliot, and M. Fortin. 2007. “3D Geometrical Modeling of Excavation Units at the Archaeological Site of Tell ‘Acharneh (Syria).” Journal of Archaeological Science 34 (2): 272–88.
- López, M. C., F. A. de Haro, L. S. Lara, A. L. Martínez Carrillo, M. Serrano Araque, and J. St P. Walsh. 2016. “Cástulo in the 21st Century: A Test Site for a New Digital Information System.” In Mobilizing the Past for a Digital Future: The Potential of Digital Archaeology, 319–35. The Digital Press @ University of North Dakota.
- McCarthy, J. 2014. “Multi-Image Photogrammetry as a Practical Tool for Cultural Heritage Survey and Community Engagement.” Journal of Archaeological Science 43: 175–85.
- Morgan, C., and S. Eve. 2012. “DIY and Digital Archaeology: What Are You Doing to Participate.” World Archaeology 44 (4): 521–37.
- Morgan, C., and H. Wright. 2018. “Pencils and Pixels: Drawing and Digital Media in Archaeological Field Recording.” Journal of Field Archaeology 43 (2): 136–51.
- Motz, C. F. 2016. “Sangro Valley and the Five (Paperless) Seasons: Lessons on Building Effective Digital Recording Workflows for Archaeological Fieldwork.” In Mobilizing the Past for a Digital Future: The Potential of Digital Archaeology, 77–109. Grand Forks, ND: The Digital Press @ University of North Dakota.
- Noble, G., M. Gondek, E. Campbell, and M. Cook. 2013. “Between Prehistory and History: The Archaeological Detection of Social Change Among the Picts.” Antiquity 87 (338): 1136–50.
- Noble, G., M. Gondek, E. Campbell, N. Evans, D. Hamilton, and S. Taylor. 2019. “A Powerful Place of Pictland: Interdisciplinary Perspectives on a Power Centre of the 4th to 6th Centuries Ad.” Medieval Archaeology 63 (1): 56–94.
- Noble, G., and J. O’Driscoll. 2019. “Fortified Settlement in Early Medieval Northern Britain and Ireland.” In Hillforts: Britain, Ireland and the Nearer Continent. Papers from the Atlas of Hillforts of Britain and Ireland Conference, June 2017, edited by G. Lock, and I. Ralston, 97–116. Oxford: Archaeopress.
- O’Driscoll, J. 2018. “Landscape Applications of Photogrammetry Using Unmanned Aerial Vehicles.” Journal of Archaeological Science: Reports 22: 32–44.
- O’Driscoll, J. 2019. “Picture Perfect: Using Drone Technology and Photogrammetry Techniques to Map the Western Stone Forts of Ireland.” Journal of Field Archaeology 44 (2): 126–46.
- Olson, B. R., R. A. Placchetti, J. Quartermaine, and A. E. Killebrew. 2013. “The Tel Akko Total Archaeology Project (Akko, Israel): Assessing the Suitability of Multi-Scale 3D Field Recording in Archaeology.” Journal of Field Archaeology 38 (3): 244–62.
- Oram, R. 2007. “Capital Tales or Burghead Bull.” In A Grey Eye Looks Back: A Festschrift in Honour of Colm ó Baoill, edited by S. Arbuthnot, and K. Hollo, 241–62. Ceann Drochaid, Perthshire: Clann Tuirc.
- O’Sullivan, A., F. McCormick, T. Kerr, and L. Harney. 2008. Early Medieval Ireland: Archaeological Excavations 1930-2004. Dublin: The Heritage Council.
- Perfetti, L., F. Fassi, and C. Rossi. 2020. “Low-Cost Digital Tools for Archaeology.” In Innovative Models for Sustainable Development in Emerging African Countries. Research for Development, edited by N. Aste, S. Della Torre, C. Talamo, R. Singh Adhikari, and C. Rossi, 137–48. Cham: Springer.
- Remondino, F. 2014. “UAV: Platforms, Regulations, Data Acquisition and Processing.” In 3D Recording and Modelling in Archaeology and Cultural Heritage: Theory and Best Practice, edited by F. Remondino, and S. Campana, 73–88. Oxford: BAR Publishing.
- Roosevelt, C. H., P. Cobb, E. Moss, B. R. Olson, and S. Ünlüsoy. 2015. “Excavation Is (Destruction) Digitization: Advances in Archaeological Practice.” Journal of Field Archaeology 40 (3): 325–46.
- Sapirstein, P., and S. Murray. 2017. “Establishing Best Practices for Photogrammetric Recording During Archaeological Fieldwork.” Journal of Field Archaeology 42 (4): 337–50.
- Sayer, F. 2014. “Politics and the Development of Community Archaeology in the UK.” The Historic Environment: Policy & Practice 5 (1): 55–73.
- Sobotkova, A., S. A. Ross, B. Ballsun-Stanton, A. Fairbairn, J. Thompson, and P. VanValkenburgh. 2016. “Measure Twice, Cut Once: Cooperative Deployment of a Generalized, Archaeology-Specific Field Data Collection System.” In Mobilizing the Past for a Digital Future. The Potential of Digital Archaeology, edited by E. W. Averett, J. M. Gordon, and D. B. Counts, 337–70. Grand Forks: The Digital Press @ The University of North Dakota.
- Spence, C. 1993. “Recording the Archaeology of London: The Development and Implementation of the DUA Recording System.” In Practices of Archaeological Stratigraphy, edited by E. C. Harris, M. R. Brown III, and G. J. Brown, 23–46. Academic Press.
- Spigelman, M., T. Roberts, and S. Fehrenbach. 2016. “The Development of the Paleoway: Digital Workflows in the Context of Archaeological Consulting.” In Mobilizing the Past for a Digital Future. The Potential of Digital Archaeology, edited by E. W. Averett, J. M. Gordon, and D. B. Counts, 337–70. Grand Forks: The Digital Press @ The University of North Dakota.
- Taylor, J., J. Issavi, Å Berggren, D. Lukas, C. Mazzucato, B. Tung, and N. Dell’Unto. 2018. ““The Rise of the Machine”: The Impact of Digital Tablet Recording in the Field at Çatalhöyük’.” Internet Archaeology 47.
- Uildriks, M. 2016. “IDig-Recording Archaeology: A Review.” Internet Archaeology 42.
- Verhoeven, G.t J. J. 2009. “Providing an Archaeological Bird’s-Eye View– An Overall Picture of Ground-Based Means to Execute Low-Altitude Aerial Photography (Laap) in Archaeology.” Archaeological Prospection 16 (4): 233–49.
- Vincent, M. L., F. Kuester, and T. E. Levy. 2014a. “OpenDig: Contextualizing the Past from the Field to the Web.” Mediterranean Archaeology and Archaeometry 14 (4): 109–16.
- Vincent, M. L., F. Kuester, and T. E. Levy. 2014b. “OpenDig: Digital Field Archeology, Curation, Publication, and Dissemination.” Near Eastern Archaeology 77 (3): 204–8.
- Waagen, J. 2019. “New Technology and Archaeological Practice. Improving the Primary Archaeological Recording Process in Excavation by Means of Uas Photogrammetry.” Journal of Archaeological Science 101: 11–20.
- Wallrodt, J. 2016. “Why Paperless: Technology and Changes in Archaeological Practice, 1996–2016.” In Mobilizing the Past for a Digital Future: The Potential of Digital Archaeology, edited by E. Walcek Averett, J. M. Gordon, and D. B. Counts, 33–50. Grand Forks, ND: The Digital Press at the University of North Dakota.
- Wilhelmson, H., and N. Dell’Unto. 2015. “Virtual Taphonomy: A New Method Integrating Excavation and Postprocessing in an Archaeological Context.” American Journal of Physical Anthropology 157: 305–21.
- Williams, G., and O. Brown. 2017. “Where the Long Shadows Fall: Urban Archaeology in the Early 20th Century–Standardizing Methodologies, Legislation, Finance and Publication.” In Architecture, Archaeology and Contemporary City Planning. Issues of Scales. Workshop Proceedings, London 22-25th September 2016, edited by J. Dixon, G. Verdiani, and P. Cornell.