Abstract
In Spain—Europe's leading chickpea producing country—chickpea (Cicer arietinum) is mainly cultivated on non-irrigated soils with low native fertility. This study was carried out from 2006 to 2008 in the province of León, Spain, under acid soil field conditions, with the aim of determining whether the application of zinc (Zn), boron (B) and molybdenum (Mo) improved chickpea growth and yield on acid soils. A split-split-plot design with three replications was used. Chickpea responded only to the Zn and Mo applications. At maturity, plants fertilized with Zn and with Mo had a greater total dry matter production and seed yield, mainly due to an increment in pod dry matter. For Zn, the highest yield was obtained with 2 mg Zn per plant (6.80 g plant−1), whereas for Mo the highest yield was obtained with 1 mg Mo per plant (6.73 g plant−1). Interaction was observed between B and Mo, interpreted as indicating that Mo can counteract the effect of B application.
Introduction
Spain is the main chickpea (Cicer arietinum L.) producing country in Europe, with 20,832 ha of land under chickpea cultivation in 2008 (Ministerio de Medio Ambiente y Medio Rural y Marino, Citation2010). Despite the importance of this crop, few studies have analysed the application of micronutrients to chickpea, and Knights et al. (Citation2007) have suggested that the lack of public investment in research, breeding and technology transfer may have contributed to the significant decline in chickpea cultivation in Europe. Chickpea is mainly cultivated on non-irrigated soils, and water stress often affects both productivity and yield stability (Kurdali Citation1996). Non-irrigated soils are generally impoverished, with low native fertility. Although chickpea is a rustic plant, a limited moisture supply together with mineral nutrient deficiencies and/or imbalances in the soil are considered major environmental stresses leading to yield loss in chickpea (Khan Citation1998; Ali et al. Citation2000). Plant nutrient availability depends, among other factors, on texture, on organic matter content and especially on soil pH. Micronutrients contribute substantially to achieving higher production through their effects on the plant itself, their role in the symbiotic nitrogen-fixing process, where micronutrient deficiencies can limit nitrogen fixation by legume-rhizobium symbiosis, and their influence on effective uptake of the principal and secondary nutrients.
Zinc (Zn) is the main micronutrient limiting chickpea productivity, boron (B) may cause yield losses of up to 100% (Ahlawat et al. Citation2007) and molybdenum (Mo) presents low availability in acidic soils. With the exception of Mo, micronutrient availability is greatest in the very slightly-to-medium acid range. In general, each tonne of chickpea grain removes 38 g of Zn, and it has been estimated that 35 g of B and 1.5 g of Mo are also removed from the soil (Ahlawat et al. Citation2007).
Zn deficiency is common in the chickpea-growing regions of the world and is perhaps the most widespread of micronutrient deficiencies (Roy et al. Citation2006; Ahlawat et al. Citation2007). Chickpea is generally considered sensitive to Zn deficiency (Khan Citation1998); more so, for example, than cereal and oil seeds (Tiwari & Pathak Citation1982). The consequences of Zn deficiency include reduced yield and a delay in crop maturity. Furthermore, Zn deficiency reduces water use, water use efficiency (Khan et al. Citation2004), nodulation and nitrogen fixation (Shukla & Yadav Citation1982; Ahlawat et al. Citation2007), which further contributes to a decrease in crop yield. Critical Zn concentrations in soils vary from 0.48–2.5 mg kg−1, depending on soil type (Ahlawat et al., 2007), and according to Ankerman & Large (Citation1974), soils have low Zn availability when there is less than 1.1 mg kg− 1 of Zn (DTPA extraction). Zn solubility decreases markedly above pH 6.0–6.5 (Sims Citation2000) and thus Zn deficiencies can be encountered in neutral to alkaline soils (Roy et al. Citation2006). Zn uptake is positively correlated with the amount of organic matter in the soil and negatively correlated with phosphorus (P) concentration in the soil (Sillanpää Citation1972; Hamilton et al. Citation1993; Ahlawat et al. Citation2007). Soils with more sand and less organic matter produce lower yields due to poor utilization of Zn (Singh & Ram Citation1996).
Although B deficiency limits chickpea productivity less than Zn deficiency (Ahlawat et al. Citation2007), it has been shown to have a significant limiting effect on chickpea yield in some regions with acid soil conditions (Srivastava et al. Citation1997). B application is most important when B concentration in soil is below 0.3 mg kg−1 (Ahlawat et al. Citation2007), and crop response to B application is higher in chickpea than in some cereals (Wankhade et al. Citation1996). B deficiency causes flower drop and, consequently, poor podding in chickpeas (Srivastava et al. Citation1997) and poor yields. According to Ankerman & Large (Citation1974), soils have low B availability when B concentration is below 0.6 mg kg−1 (hot water extraction), whereas according to Sillanpää (Citation1972), the deficiency limit may be around 0.5 mg kg−1, depending on conditions, extraction time and the soil. B deficiency can be caused by high soil pH, and availability decreases at a pH above 6.5–7.0 (Sims Citation2000) on highly leached sandy soils or on low organic matter soils.
Mo deficiency is common in acidic soils. Total Mo content varies from 0.2–5.0 mg kg−1 (Sims Citation2000) but Mo in soil is largely unavailable, with less than 0.2 mg kg−1 generally being reported as soluble Mo (Sillanpää Citation1972). According to Ankerman & Large (Citation1974), soils have low Mo availability when Mo concentration in the soil is below 0.11 mg kg−1 (ammonium acid oxalate). In Mo-deficient chickpea, the flowers produced are fewer in number, smaller in size and many of them fail to open or to mature, leading to lower seed yield (Ahlawat et al. Citation2007). Mo is directly related to N fixation by legumes (Roy et al. Citation2006) and Mo availability increases as the soil pH approaches neutrality (pH 7.0) or goes higher (Sims Citation2000); it is lower in the very slightly-to-medium acid range. Mo deficiency is common on very acidic soils, especially with crops that are very sensitive to low concentrations of Mo, such as legumes (Sims Citation2000). High phosphate levels are positively correlated with Mo deficiency.
Foliar and soil application of micronutrients is effective in some cases (Ali et al. Citation2000; Roy et al. Citation2006). However, Zn, B and Mo application results are controversial according to reports in the literature (Yanni Citation1992; Bhuiyan et al. Citation1997; Braga & Vieira Citation1998; Ali et al. Citation2000; Johnson et al. Citation2005; Johansen et al. Citation2007; Shil et al. Citation2007). Under pot conditions with acidic soils at high moisture availability, Zn and B soil and Mo foliar applications increased chickpea total dry matter and improved seed yield (Valenciano et al. Citation2009; 2010). However, nutrient interactions in annual crops affect yield, and these interactions can be positive, negative or neutral (Fageria et al. Citation1997). Soil, plant and climatic factors can influence interaction; under pot conditions with acidic soils at high moisture availability, Zn application was more efficient when it was applied in conjunction with B and Mo (Valenciano et al. Citation2010).
This study was conducted to determine the effect of Zn and B soil applications and Mo foliar application on growth and seed yield of a Kabuli chickpea under field conditions on acidic soils. The possible interactions of these micronutrients were also studied.
Material and methods
Site characteristics
Three experimental fields were established in the province of León (Spain), between 2006 and 2008, using a Kabuli chickpea ecotype (cv. Pedrosillano) and different micronutrient applications. The seed of this variety is small (1000-seed weight, 340 g) cream, rounded and smooth.
The plots were located in Ribas de la Valduerna (León, Spain) (42°18.5′N, 5°57.1′W). Wheat had been the previous crop on all plots. The experimental fields were not fertilized for the present experiment, and the main physical and chemical properties of the soils used are listed in . The experiments were conducted using acidic soils, which presented a medium availability of Zn and a very low to high availability of B according to Ankerman & Large (Citation1974), and high total Mo availability according to Gupta (Citation1997). Weather-related parameters for this area during the experimental period are shown in .
Figure 1 Climatic conditions at Ribas de la Valduerna (León, Spain) during the experimental period (Source: Meteorological Station, Sugar Refinery, La Bañeza, Spain).
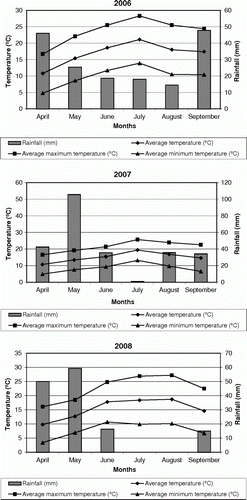
Table 1 Main physical and chemical characteristics of soils used in the experiments, with local names.
Experimental design
The experiment was carried out following a statistical pattern of split-split-plots according to a randomized complete block design, with three replications. The main factor was Mo application, with two levels of Mo, i.e. 0 and 241 g Mo ha−1 (corresponding to 0 and 1 mg plant−1 at establishment), for which the treatment codes were Mo0 and Mo1, respectively. Mo was applied to the leaves as Mo solution(ammonium molybdate and sodium molybdate) using a backpack sprayer, applying 0.4 m3 of spraying solution/ha. The secondary factor was B application, with two levels of B, i.e. 0 and 241 g B ha−1 (corresponding to 0 and 1 mg plant−1 at establishment), for which the treatment codes were B0 and B1, respectively. B was applied to the soil in plant rows as B solution (boric acid). The tertiary factor was Zn application, with five levels of Zn, i.e. 0, 120.5, 241.0, 482.0 and 964.0 g Zn ha−1 (corresponding to 0, 0.5, 1, 2 and 4 mg plant−1 at establishment), for which the treatment codes were Zn0, Zn0.5, Zn1, Zn2 and Zn4, respectively. Zn was applied to the soil in plant rows as Zn chelate (chelating agents: DTPA, EDTA and HEDTA).
Conventional tillage was used for land preparation, including mouldboard ploughing and vibrating tine cultivation to prepare a suitable seedbed. The experimental plot was 82.5 m2, with rows 0.55 m apart (5 rows per plot), plot length of 30 m and a space between seeds of 0.068 m. Sowing was carried out with a single-seed pneumatic precision planter on 20 April 2006, 22 April 2007 and 30 April 2008. Plant population density was 24.1 plants/m2.
Three weeks after emergence (6 May in 2006, 4 May in 2007 and 17 May in 2008), the five Zn concentrations and the two B concentrations were applied separately to the soil of each experimental plot. Mo applications were carried out by spraying each experimental plot at 30 days after emergence (Bhanavase & Patil Citation1994).
The trial plots were irrigated twice, 1.5–2 weeks before and 1.5–2 weeks after flowering, using a furrow irrigation system (approximately 30 L m−2 of water was applied each time). Chlorothalonil (tetrachloroisophthalonitrile) and quinosol (8-hydroxyquinoline sulphate) plus thiram (tetramethyl-thiuram sulfide) were used to reduce the incidence of disease (Ascochyta blight and root rots) and for chickpea plant protection (Ondategui Citation1996) throughout the crop cycle. No pests or disease were observed during the experimental period.
Data collection
Eighty plants were collected from each plot. Eight effective areas in each plot were randomly selected and then, from each of these areas, 10 consecutive plants bearing productive pods were hand-harvested at seed maturity (10 August in 2006, 4 August in 2007 and 11 August in 2008). Each plant was extracted manually with an agricultural tool. Plants were cut at ground level to separate the roots from the stems. Roots, stems with leaves (leaf-stems) and pods including seeds were separated, oven-dried at 80 °C to a constant weight to allow correction of data to absolute dry weight, and weighed. Data were recorded for the following characteristics:
• | Dry weight (DW) data: DW data were recorded for the 80 harvested plants and this was used to calculate indices of DW partitioning: root weight ratio (RWR = root DW/total DW); leaf-stem weight ratio (LSWR = leaf-stem DW/total DW); pod weight ratio (PWR = pod DW/total DW); and harvest index (HI = seed DW/total DW). | ||||
• | Number of pods per plant: this was determined from the 80 harvested plants. | ||||
• | Number of seeds per pod: this was determined from the 80 harvested plants. | ||||
• | Dry seed weight (g): this was determined from a sample of 1000 chickpea seeds. | ||||
• | Grain yield (g plant−1) was calculated from the yield components. |
Data analyses
Combined analysis of variance was performed using the routines of SPSS version 15.0.1. Mo application, B application, Zn application and environment were considered to be fixed effects. Means comparison was based on the Tukey test (P < 0.01 and P < 0.05) (Steel & Torrie Citation1986). Different correlations were also calculated and a regression analysis was performed.
Results and discussion
The symptoms of Zn, B and Mo deficiencies (Roy et al. Citation2006) were not observed in any of the sub-subplots; however, there were significant differences between the treatments for Zn soil application and for Mo foliar application.
Table 2 Effects of concentration of Zn, B and Mo applications on dry matter production of chickpea plants at maturity, indicating significance of analysis of variance and coefficient of variation (CV).
There were highly significant differences between environments for dry matter (DM) production (), for yield and for yield components (). This indicates the strong influence of the environment on chickpea performance, which has also been reported by other authors (Singh & Sandhu Citation2006). Environmental conditions during experiments affected plant response differently. At maturity, DM production was highest in Era-2008 (18.46 g plant−1) and all yield components also improved in Era-2008; therefore the highest yield was obtained from Era-2008 (7.19 g plant−1) (). Valenciano et al. (Citation2010) reported similar results in pot assays. These differences could mainly be explained by the different soil pH values. In Era-2008, the pH value was higher than 6 and according to Ondategui (Citation1996) and Ahlawat et al. (Citation2007), chickpea grows better in pH conditions ranging from 6 to 9. In addition, the high level of phosphorus in the Sotico-2006 and Housa-2007 soils may have limited the yields due to its antagonistic effect on other nutrients, according to Sillanpää (Citation1972), Ankerman & Large (Citation1974) and Hamilton et al. (Citation1993). Temperature had less influence since it was not critical (Nielsen Citation2001), and was similar over the 3 years. In contrast, Sotico-2006 produced the highest HI; in Era-2008, total DW increased more than yield, which resulted in a lower HI (), although DW in Era-2008 increased mostly in pod DW. Although drought reduces chickpea growth (Gunes et al. Citation2007), soil moisture contributed little to differences between years in the present study. During the sowing-establishment period, soil moisture was not critical as rainfall was satisfactory. The greatest differences in rainfall occurred during the flowering-maturity period, but two supplementary irrigations (Pacucci et al. 2006) were applied during this period to compensate for moisture differences.
Figure 2 Effect of environment on yield components (P/P = pods plant−1; S/P = seeds pod−1; 1000-W = 1000-seed weight in g), yield (Y = g plant−1), total dry weight (total DW = g plant−1) and harvest index (HI).
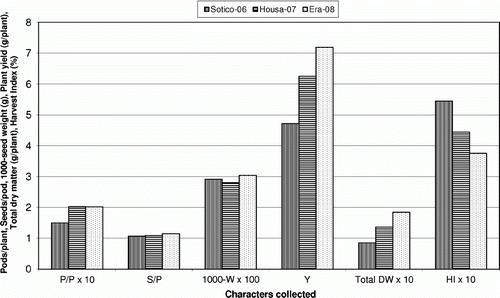
Table 3 Mean yield components and seed yield of the main treatment, indicating significance of analysis of variance and coefficient of variation (CV).
Chickpea responded to the Zn soil applications even though Zn availability is higher at this pH range (Ankerman & Large Citation1974; Royet al. Citation2006); however, a high P soil concentration can reduce Zn uptake (Sillanpää Citation1972; Hamilton et al. Citation1993; Ahlawat et al. Citation2007). Brennan et al. (Citation2001) reported that the relative response of chickpea to Zn application is greater than that of other crops. As with other leguminous crops, Zn application resulted in more vegetative growth on acid soil (Singh et al. Citation1992), leading to higher DM production and greater yield ( and ). Zn soil application increased chickpea growth (Khan et al. Citation2000) and thus at maturity plants fertilized with Zn had a greater total DW (Brennan et al. Citation2001), mainly due to increased pod DW per plant. In addition, Zn application increased root DW (Ahlawat et al. Citation2007) and leaf-stem DW, although in pot assays these characteristics were not affected (Valenciano et al. 2009; Citation2010), indicating that pot cultivation may limit growth, especially root growth. Treatments influenced DW partitioning between plant organs. A relationship between Zn soil application and relative DW of different chickpea plant organs was established (); LSWR decreased to 2.4 g plant−1, whereas PWR increased to 2.4 g plant−1. The least number of pods per plant (14.29 pods plant−1) and the lowest yield were obtained with Zn0 (4.69 g plant−1). Zn soil applications increased chickpea yield (Brennan et al. Citation2001), but this increase only occurred up to Zn2 (6.80 g plant−1), whereas the Zn4 treatments reduced maximum yield (Tripathi et al. Citation1997). Valenciano et al. 2009; (Citation2010) obtained similar results in the same environments but under pot conditions at high moisture availability; they also recorded differences for 1000-seed weight. Zn soil application increased yield (Khan et al. Citation2000) and, as with the common bean (Valenciano et al. Citation2007), this increase was the result of an increase in the number of pods per plant (Khan Citation1998; Valenciano et al. 2009; Citation2010). Although the response to Zn soil application varied with environment, as Loneragan & Webb (Citation1993) observed, variance analysis established that the environment×Zn interaction produced no significant differences in growth and yield. The response was similar between plots because all soils had medium Zn availability (Ankerman & Large Citation1974). However, the higher pH in Era-2008 may have reduced Zn availability, whereas high soil P levels in Sotico-2006 and Housa-2007 may have induced Zn deficiency by decreasing Zn uptake from soil (Sillanpää Citation1972; Hamilton et al. Citation1993; Ahlawat et al. Citation2007). HI decreased when 4 mg Zn per plant was applied and high Zn applications decreased the yield slightly (Tripathi et al. Citation1997). HI was higher at 1 g plant−1, and the highest yield was at 2 g plant−1; however, the highest total DW was also at 2 g plant−1.
Figure 3 Relationships between zinc soil application and relative dry matter production of different chickpea plant organs. RWR = root weight ratio, LSWR = leaf-stem weight ratio, PWR = pod weight ratio and HI = harvest index.
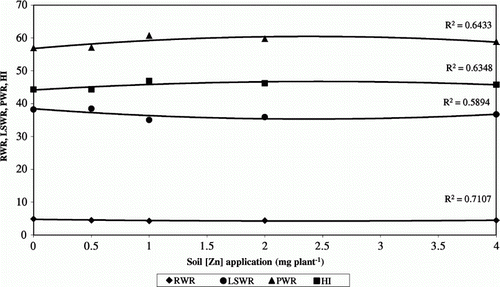
Chickpea did not respond to B soil application, although in similar environments, Valenciano et al. (Citation2010) found a significant response to B soil application in pot assays. This could be explained by soil moisture status; in pot assays, the soil moisture was maintained near field capacity, whereas in field conditions, limited irrigation was used. This may indicate that chickpea response to nutrient application varies according to soil moisture content (Sekhon & Singh Citation2007) or that chickpea plants in field conditions have access to higher soil volume, enabling them to meet their moisture needs. According to the literature, chickpea responds to B soil application in B deficient soils (Wankhade et al. Citation1996; Roy et al. Citation2006; Ahlawat et al. Citation2007; Shil et al. Citation2007). Consequently, significant interaction between soils and B application was expected due to the difference in B availability in the soils, which was low in some of them, such as Housa-2007 (Ankerman & Large Citation1974). However, no response was observed in any of the environments. B soil application did not cause either toxicity or a reduction in yield, perhaps because the doses used were not very high (Panwar et al. Citation1998), since high rates can cause a reduction in yield, especially in dry conditions (Ahlawat et al. Citation2007). Although Ali & Mishra (Citation2001) did find a significant response, they carried out foliar rather than soil applications.
Chickpea responded to Mo foliar application (Ali & Mishra Citation2001), which might be explained by a decrease in availability due to reduced soil pH (Ankerman & Large Citation1974). As with other leguminous crops, Mo application resulted in greater DM production on acidic soil (Singh et al. Citation1992). Mo foliar application affected chickpea growth (), and at maturity plants fertilized with Mo had a greater total DM production (Bhanavase & Patil Citation1994); according to Johansen et al. (Citation2007), lack of Mo causes a reduction in growth. An increased Mo supply led to a corresponding increase in DM production, primarily in the number of pods (including seeds), and Ahlawat et al. (2007) have indicated that Mo application increases the number of flowers. Leaf-stem DW was not affected by Mo application. Treatments influenced DW partitioning between plant organs, except for RWR, and LSWR was highest at Mo0 (38.77), whereas PWR was highest at Mo1 (60.72). In addition, chickpea yield characteristics were affected by Mo application (), a finding that has also been reported by Shil et al. (Citation2007). There were highly significant differences for Mo foliar application with regard to the number of pods per plant and yield but, contrary to pot assays (Valenciano et al. Citation2010), the number of seeds per pod was not affected. The least number of pods per plant (16.44 pods plant−1) was obtained without Mo treatment. Bozoglu et al. (Citation2007) reported contrary results, obtaining a decrease in pods per plant with Mo application, but their experiments were carried out with neutral pH soil where Mo availability is higher. As with the pot assays (Valenciano et al. Citation2010), the highest yield was obtained when Mo application was carried out (6.73 g plant−1), and this may be due to an increase in availability and/or possibly an increase in N2 fixation (Deo & Kothari Citation2002), since nodulation improved (Yanni Citation1992; Bhanavase & Patil Citation1994). Singh & Singh (Citation1994) also recorded yield increases with Mo application. However, Braga & Vieira (Citation1998) did not find an increment in yield, but they carried out Mo application later (57–60 days after emergence). Although total Mo was high, according to Gupta (Citation1997), the application of Mo still increased chickpea yield, probably due to low Mo availability resulting from low soil pH (Sillanpää Citation1972; Sims Citation2000; Ahlawat et al. Citation2007); total Mo soil content apparently does not always represent the amount available to plants. As Mo application increased seed production, its application resulted in an increased HI (6.8%). Variance analysis established that the environment×Mo interaction produced significant differences in total DW, in the number of pods per plant and in yield. The highest total DW was obtained when Mo was applied in Era-2008 (19.26 g plant−1), whereas the highest number of pods per plant and the highest yield were obtained when Mo was applied in Housa-2007, with 26.06 pod plant−1 and 8.10 g plant−1, respectively. Different soil pH levels could explain this interaction since, according to Ankerman & Large (Citation1974), soil pH is the most influential factor affecting Mo availability, whereas differences in phosphate levels have less influence. Although organic matter also influences Mo availability because it helps prevent leaching (Wichard et al. Citation2009), organic matter levels were very similar in all three soils.
These interaction results are contrary to those reported by Valenciano et al. (Citation2010) for pot assays with acidic soils. The only significant interactions found in the present study were between B and Mo applications, an effect that was not reported by Valenciano et al. (Citation2010). For total DW there was a highly significant interaction between B and Mo; the highest total DW was obtained with B1 and Mo1 (16.20 g plant−1) and the lowest with B1 and Mo0 (11.81 g plant−1). Shil et al. (Citation2007) also reported finding an interaction between B and Mo, for plant height. There was also a highly significant interaction between B and Mo for pod DW, where the highest pod DW was obtained with B1 and Mo1 (9.91 g plant−1), and the lowest with B1 and Mo0 (6.44 g plant−1). This finding can be interpreted as indicating that Mo can counteract the effect of B application. There was no interaction between Zn×B or between Zn×Mo, as reported by Valenciano et al. (Citation2010) for pot assays. There was a highly significant B×Mo interaction as regards the number of pods per plant and seed yield. The highest number of pods per plant (22.73 pods plant−1) and the highest seed yield (7.61 g plant−1) were obtained with B1 and Mo1 treatments. The lowest number of pods per plant (15.30 pods plant−1) and the lowest seed yield (4.99 g plant−1) were obtained with B1 and Mo0 treatments. Shil et al. (Citation2007) also detected an interaction between B and Mo, and in their study they found that the combined application of B and Mo produced better results than single applications. There was no interaction between Zn×B, between Zn×Mo, or between the three micronutrients, as reported by Valenciano et al. (Citation2010) for pot assays.
According to Bhatia et al. (Citation1993) and Omar & Singh (Citation1997), total DW is highly correlated with seed yield (0.892). HI showed a low significant positive correlation with the number of pods per plant (0.210), although this was the yield component that exhibited the highest positive correlation with HI, a finding that has also been reported by Bhatia et al. (Citation1993). The number of pods per plant was the most influential yield component, and the one most closely correlated with seed yield (0.969) (Maiti & Wesche-Ebeling Citation2001), but was also the most variable component (Bhatia et al. Citation1993). The number of seeds per pod was the least variable yield component, and the average for seeds per pod obtained in this study fell within the mean range reported in the literature (Khanna-Chopra & Sinha Citation1987).
Conclusions
This study shows that Zn soil and Mo foliar applications in acidic soils increase total DM and seed yield, primarily due to an increase in the number of pods per plant. High levels of Zn can cause a reduction in yield. Mo foliar applications improve the harvest index. Finally, the number of pods per plant is the most influential yield component, and the one that is most closely correlated with seed yield. Zn soil and Mo foliar applications can increase the yield of Kabuli chickpea cultivated in medium-acid soils with limited irrigation.
References
- Ahlawat , IPS , Gangaiah , B and Ashraf Zadid , M . 2007 . “ Nutrient management in chickpea ” . In Chickpea breeding and management , Edited by: Yadav , SS , Redden , R , Chen , W and Sharma , B . 213 – 232 . Wallingford, Oxon, , UK : CAB International .
- Ali , M , Dahan , R , Mishra , JP and Saxena , NP . 2000 . “ Towards the more efficient use of water and nutrients in food ” . In Linking research and marketing opportunities for pulses in the 21st century. Proceedings of the Third International Food Legumes Research Conference. Adelaide, Australia, 22–26 September , Edited by: Knight , R ed. 355 – 368 . Dordrecht, The Netherlands, Kluwer Academic Publishers .
- Ali , M and Mishra , JP . 2001 . Effect of foliar nutrition of boron and molybdenum on chickpea . Indian Journal of Pulses Research , 14 ( 1 ) : 41 – 43 .
- Ankerman , D and Large , R . 1974 . Soil and plant analysis , New York, , USA : A&L Agricultural Laboratories, Inc .
- Bhanavase , DB and Patil , PL . 1994 . Effects of molybdenum on nodulation in gram . Journal of Maharashtra Agricultural Universities , 19 ( 1 ) : 127 – 129 .
- Bhatia , VS , Singh , BN and Lal , S . 1993 . Variability and interrelationship of yield and its attributes in chickpea . Indian Journal of Pulses Research , 6 ( 1 ) : 1 – 5 .
- Braga , NR and Vieira , C . 1998 . Efeito da inoculação com Bradyrhizobium sp., nitrogênio e micronutrientes no rendimento do grão-de-bico . Bragantia , 57 ( 2 ) : 349 – 353 .
- Brennan , RF , Bolland , MDA and Siddique , KHM . 2001 . Responses of cool-season grain legumes and wheat to soil-applied zinc . Journal of Plant Nutrition , 24 ( 4–5 ) : 727 – 741 .
- Bhuiyan , MAH , Khanam , D , Rahman , MHH and Hossain , AKM . 1997 . Influence of Rhizobium inoculum, molybdenum and boron on chickpea (Cicer arietinum L.) in grey terrace soil of Bangladesh . Annals of Bangladesh Agriculture , 7 ( 2 ) : 119 – 126 .
- Bozoglu , H , Ozcelik , H , Mut , Z and Pesken , E . 2007 . Response of chickpea (Cicer arietinum L.) to zinc and molybdenum fertilization . Bangladesh Journal of Botany , 36 ( 2 ) : 145 – 149 .
- Deo , C and Kothari , ML . 2002 . Effect of modes and levels of molybdenum application on grain yield protein content and nodulation of chickpea grown on loamy sand soil . Communications in Soil Science and Plant Analysis , 33 ( 15–18 ) : 2905 – 2915 .
- Fageria , NK , Baligar , VC and Jones , CA . 1997 . Growth and mineral nutrition of field crops , New York, , USA : M Deker .
- Gunes , A , Inal , A , Adak , MS , Alpaslan , M , Bagci , EG , Erol , T and Pilbeam , DJ . 2007 . Mineral nutrition of wheat, chickpea and lentil as affected by mixed cropping and soil moisture . Nutrient Cycling in Agroecosystems , 78 ( 1 ) : 83 – 96 .
- Gupta , UC . 1997 . Molybdenum in agriculture , Cambridge, , UK : Cambridge University Press .
- Hamilton , MA , Westermann , DT and James , DW . 1993 . Factors affecting zinc uptake in cropping systems . Soil Science Society of America Journal , 57 ( 5 ) : 1310 – 1315 .
- Johansen , C , Musa , AM , Kumar Rao , JVDK , Harris , D , Ali , MY , Shahidullah , AKM and Lauren , JG . 2007 . Correcting molybdenum deficiency of chickpea in the High Barind Tract of Bangladesh . Journal of Plant Nutrition and Soil Science , 170 ( 6 ) : 752 – 761 .
- Johnson , SE , Lauren , JG , Welch , RM and Duxbury , JM . 2005 . A comparison of the effects of micronutrient seed priming and soil fertilization on the mineral nutrition of chickpea (Cicer arietinum), lentil (Lens culinaris) rice (Oriza sativa) and wheat (Triticum aestivum) in Nepal . Experimental Agriculture , 41 ( 4 ) : 427 – 448 .
- Khan , HR . 1998 . Response of chickpea (Cicer arietinum) to zinc supply and water deficits. PhD thesis, Department of Plant Science , Glen Osmond, , Australia : University of Adelaide .
- Khan , HR , McDonald , GK and Rengel , Z . 2000 . Response of chickpea genotypes to zinc fertilization under filed conditions in South Australia and Pakistan . Journal of Plant Nutrition , 23 ( 10 ) : 1517 – 1531 .
- Khan , HR , McDonald , GK and Rengel , Z . 2004 . Zinc fertilization and water stress affects plant water relations, stomatal conductance and osmotic adjustment in chickpea (Cicer arietinum) . Plant and Soil , 267 ( 1–2 ) : 271 – 284 .
- Khanna-Chopra , R and Sinha , SK . 1987 . “ Chickpea: physiological aspect of growth and yield ” . In The chickpea , Edited by: Saxena , MC and Singh , KB . 163 – 189 . Wallingford, Oxon, , UK : CAB International .
- Knights , EJ , Açikgöz , N , Warkentin , T , Bejiga , G , Yadav , SS and Sandhu , JS . 2007 . “ Area, production and distribution ” . In Chickpea breeding and management , Edited by: Yadav , SS , Redden , R , Chen , W and Sharma , B . 167 – 178 . Wallingford, Oxon, , UK : CAB International .
- Kurdali , F . 1996 . Nitrogen and phosphorus assimilation, mobilization and partitioning in rainfed chickpea (Cicer arietinum L.) . Field Crops Research. , 47 ( 2–3 ) : 81 – 92 .
- Loneragan , JF and Webb , MJ . 1993 . “ Interactions between zinc and other nutrients affecting the growth of plants ” . In Zinc in soils and plants , Edited by: Robson , AD . 45 – 57 . Dordrecht, , The Netherlands : Kluwer Academic Publishers .
- Maiti , RK and Wesche-Ebeling , P . 2001 . “ Vegetative and reproductive growth and productivity ” . In Advances in chickpea science , Edited by: Maiti , R and Wesche-Ebeling , P . 67 – 104 . Enfield, NH, , USA : Science Publishers .
- Ministerio de Medio Ambiente y Medio Rural y Marino 2010 . Anuario de estadística 2009. Madrid, Ministerio de Medio Ambiente y Medio Rural y Marino .
- Nielsen , DC . 2001 . Production functions for chickpea, field pea and lentil in the Central Great Plains . Agronomy Journal , 93 ( 3 ) : 563 – 569 .
- Omar , M and Singh , KB . 1997 . Increasing seed yield in chickpea by increased biomass yield . International Chickpea and Pigeonpea Newsletter , 4 : 14
- Ondategui , J . 1996 . “ El garbanzo In ” . In El cultivo de las leguminosas de grano en Castilla y León , 357 – 398 . Valladolid, , Spain : Junta de Castilla y León .
- Pacucci , G , Troccoli , C and Leoni , B . 2006 . Effect of supplementary irrigation on yield of chickpea genotypes in a Mediterranean climate . Agricultural Engineering International 8: Manuscript LW 04: 005 ,
- Panwar , BS , Gupta , SP and Kala , R . 1998 . Responses to boron in pearl millet and chickpea in a pot experiment with a non-calcareous soil in India . Acta Agronomica Hungarica , 46 ( 4 ) : 335 – 340 .
- Roy , RN , Finck , A , Blair , GJ and Tandon , HLS . 2006 . Plant nutrition for food security. A guide for integrated nutrient management. FAO Fertilizer and Plant Nutrition Bulletin 16 , Rome, , Italy : Food and Agriculture Organization of the United Nations .
- Sekhon , HS and Singh , G . 2007 . “ Irrigation management in chickpea ” . In Chickpea breeding and management , Edited by: Yadav , SS , Redden , R , Chen , W and Sharma , B . 246 – 267 . Wallingford, Oxon : UK, CAB International .
- Shil , NC , Noor , S and Hossain , MA . 2007 . Effects of boron and molybdenum on the yield of chickpea . Journal of Agriculture and Rural Development (Gazipur) , 5 ( 1–2 ) : 17 – 24 .
- Sillanpää , M . 1972 . Trace elements in soils and agriculture , Rome, , Italy : Food and Agriculture Organization of the United Nations .
- Sims , TT . 2000 . “ Soil fertility evaluation ” . In Handbook of soil science , Edited by: Summer , ME . 113 – 154 . Boca Raton, Florida, , USA : CRC Press .
- Singh , M and Ram , N . 1996 . Effect of soil enrichment with zinc on crop yields and its replenishment in Mollisols of northern India . Agrochimica , 40 ( 1 ) : 19 – 24 .
- Singh , A and Sandhu , JS . 2006 . Genotype×environment interaction in chickpea . Crop Improvement , 33 ( 1 ) : 67 – 69 .
- Singh , V and Singh , GP . 1994 . Effect of applied potassium and molybdenum on yield and composition of chickpea . Journal of Potassium Research , 10 ( 4 ) : 411 – 414 .
- Singh , A , Singh , BB and Patel , CS . 1992 . Response of vegetable pea (Pisum sativum) to zinc, boron and molybdenum in an acidi Alsifol of Meghalaya . Indian Journal of Agronomy , 37 ( 3 ) : 615 – 616 .
- Srivastava , SP , Yadav , CR , Rego , TJ , Johansen , C and Saxena , NP . 1997 . “ Diagnosis and alleviation of boron deficiency causing flower and pod abortion in chickpea (Cicer arietinum L.) in Nepal ” . In Boron in soils and plants, Developments in Plant and Soil Sciences, 76 , Edited by: Bell , RW and Rerkasem , B . 95 – 99 . Dordrecht, , The Netherlands : Kluwer Academic Publishers .
- Steel , RGD and Torrie , JH . 1986 . Bioestadística: Principios y procedimientos , México DF, México : McGraw Hill .
- Shukla , UC and Yadav , OP . 1982 . Effect of phosphorus and zinc on nodulation and nitrogen fixation in chickpea (Cicer arietinum L.) . Plant and Soil , 65 ( 2 ) : 239 – 248 .
- Tiwari , NK and Pathak , AN . 1982 . Studies of the zinc requirements of different crops . Experimental Agriculture , 18 ( 4 ) : 393 – 398 .
- Tripathi , HC , Singh , RS and Misra , VK . 1997 . Response of gram (Cicer arietinum) to sulphur and zinc fertilization . Indian Journal of Agricultural Sciences , 67 ( 11 ) : 541 – 542 .
- Valenciano , JB , Miguélez-Frade , MM , Marcelo , V and Reinoso , B . 2007 . Response of irrigated common bean (Phaseolus vulgaris) yield to foliar zinc application in Spain . New Zealand Journal of Crop and Horticultural Science , 35 ( 3 ) : 325 – 330 .
- Valenciano , JB , Miguélez-Frade , MM and Marcelo , V . 2009 . Response of chickpea (Cicer arietinum) to soil zinc application . Spanish Journal of Agricultural Research , 7 ( 4 ) : 952 – 956 .
- Valenciano , JB , Marcelo , V and Boto , JA . 2010 . Response of chickpea (Cicer arietinum) yield to micronutrient application under pot conditions in Spain . Spanish Journal of Agricultural Research , 8 ( 3 ) : 797 – 807 .
- Wankhade , SG , Dakhore , RC , Wanjari , SS , Patil , DB , Potdukhe , NR and Ingle , RW . 1996 . Response of crops to micronutrients . Indian Journal of Agricultural Research , 30 ( 3–4 ) : 164 – 168 .
- Wichard , T , Mishra , B , Myneni , SCB , Bellenger , JP and Kraepiel , AML . 2009 . Storage and bioavailability of molybdenum in soils increased by organic matter complexation . Nature Geoscience , 2 ( 9 ) : 625 – 629 .
- Yanni , YG . 1992 . Performance of chickpea, lentil and lupin nodulated with indigenous or inoculated rhizobia micropartners under nitrogen, boron, cobalt and molybdenum fertilization schedules . World Journal of Microbiology and Biotechnology , 8 ( 6 ) : 607 – 613 .