Abstract
Elevated atmospheric carbon dioxide (CO2) increases the instantaneous rate of photosynthesis in C3 plants. With ample nutrients and water, this so-called ‘CO2 fertilization effect’ generally leads to improved growth and production of many plant species that humans use as food. To determine whether increasing CO2 could be beneficial to growth and yield of leafy Brassica vegetables, trials were established in a Free Air CO2 Enrichment facility at Horsham, Australia using projected atmospheric CO2 concentrations for the year 2050. The experiment measured the interacting effects of CO2 (ambient CO2 366 µmol/mol, elevated CO2 562 µmol/mol), nitrogen (low/high) and Brassica rapa cultivars (× 2) on shoot growth under south-eastern Australian conditions. For cultivars ‘Karate’ and ‘Chop Suey’, significant interaction between elevated CO2 and a high nitrogen treatment (196 kg/ha) increased the fresh weights of shoots.
Introduction
Global atmospheric CO2 concentration (a[CO2]) has increased from about 280 µmol/mol in pre-industrial times (AD 1750) (IPCC Citation2007) to a current international level of about 370 µmol/mol. The Intergovernmental Panel on Climate Change (IPCC) Citation2007 emissions scenario A1B (‘mid-range’ of greenhouse gas emissions) indicates that a[CO2] will reach 550 µmol/mol by 2050 (Carter et al. Citation2007; Meehl et al. Citation2007).
Future levels of CO2 and a hotter climate could significantly impact on the Australian Brassica vegetable industry worth approximately AU$350 million (ABS Citation2009). Production is centred in Victoria in the south-east of Australia. Australian farmers grow broccoli, cabbage, cauliflower, Brussels sprouts and a range of Asian bred cultivars e.g. Chinese cabbage and bok choy. Brassica vegetables are important horticultural crops worldwide and across Asia many cultivars are grown for consumption of their leaves.
Potential effects of elevated CO2 on plants include changes in growth, photosynthesis, source-sink ratio, partitioning and translocation of metabolites, photosynthetic enzymes, respiratory rate, leaf area index, stomatal conductance, transpiration rate, biomass production and water use efficiency (Saralabai et al. Citation1997). Carbon dioxide is the direct substrate of photosynthesis and increasing concentrations typically stimulate plant growth. The process is often referred to as the ‘CO2 fertilization effect’. In particular, C3 plants can benefit from elevated [CO2] since C3 photosynthesis is not substrate saturated at current [CO2] (Bowes Citation1993; Long et al. Citation2004).
Glasshouse production of many ornamental and vegetable crops commonly uses increased CO2 to achieve higher yields (Wittwer Citation1992). The literature describes a range in the magnitude of the benefit. The IPCC (Citation1997) has suggested that the effect on a wide range of plants of doubling in [CO2] varies from a 10% increase to almost 300% increase in biomass. A review of hundreds of studies concluded that the response of food and flower crops to a doubling of [CO2] was an average improvement in productivity of around 30% depending on nutrient and water supply (Wittwer Citation1992; Ainsworth & Long Citation2005).
While there is an instantaneous positive leaf response to increased CO2 in C3 plants, the net effect on whole plants depends on the interaction of temperature, moisture supply, nutrients, plant-plant competition, pests and diseases, acclimation, within-plant regulation and management practices (Steffen & Canadell Citation2005). Depending on these interactions, CO2 fertilization could lead to a net positive effect, little or no effect or in some cases even a negative effect (Steffen & Canadell Citation2005). Low nutrient concentrations limit the response of most crops to CO2 (Cure & Acock Citation1986). One of the key environmental factors that can influence rates of plant growth in conjunction with e[CO2] is soil nitrogen (N). Nitrogen is a basic constituent of macromolecules such as proteins and is an important element of chlorophylls, coenzymes and some plant hormones (Weier et al. Citation1974).
In spite of increased plant growth under e[CO2] (Bazzaz Citation1990; Amthor Citation2001), aerial plant parts generally accumulate less N, and C/N ratios increase (Baxter et al. Citation1994; Epron et al. Citation1996; Karowe et al. Citation1997). Reductions in foliar concentrations of proteins then become evident following increased C/N ratios (Cotrufo et al. Citation1998; Jablonski et al. Citation2002). With higher C/N ratios, plant foods tend to be of less nutritional value, and because Australian soils are generally nutrient poor, this degrading effect could be exacerbated.
There are many technical difficulties in conducting crop response studies for e[CO2] (Uprety Citation1998). Older approaches used greenhouses, leaf cuvettes, phytotrons, air exclusion systems and open-top chambers. However, environmental control in these systems induces some uncertainty in the extrapolation of results to variable natural environments. Erickson (Citation1993) warned that any positive effects on yields from CO2 fertilization, demonstrated in controlled experiments, would be weak in farm conditions given water and nutrient limits.
Free Air CO2 Enrichment (FACE) is a field-based technique used to investigate how crop, forest and natural plant communities respond to e[CO2] (Allen et al. Citation1993; Xu et al. Citation1997; Hendrey et al. Citation1999; Miglietta et al. Citation2001; Okada et al. Citation2001; Hovenden et al. Citation2006). Target plants are grown within rings open to the environment that have a monitored and regulated supply of CO2 to raise a[CO2] concentration to the desired target. FACE systems enable plants to be grown under natural conditions for long periods. While all e[CO2] systems have artefacts, FACE systems produce an environment more similar to field conditions than most other e[CO2] technologies available (Ainsworth et al. Citation2008), with crop yield responses generally considered consistent with other research methodologies (Tubiello et al. Citation2007).
To successfully adapt crop production practices and agro-ecosystem management in response to increasing a[CO2], plant production must be studied in a range of environments under field conditions. In this paper we report on results of the first FACE experiment to study e[CO2] and N effects on crop growth of two leafy Brassica rapa vegetables (C3 plants).
Materials and methods
Overview of AGFACE system
Experimentation took place at the Australian Grains Free Air CO2 Enrichment (AGFACE) facility. AGFACE is located on 7.5 ha in the grain-growing region of south-eastern Australia at Horsham (36°45′S, 142°06′E; 127 m elevation), Victoria. Vegetables were grown within eight, 4 m diameter AGFACE rings providing an e[CO2] atmosphere and in eight experimental control plots of a[CO2]. Carbon dioxide concentrations were monitored at the centre of each ring by infra-red gas analysers. Feedback to a controller was used to regulate the injection of CO2 to maintain a target concentration of 550 µmol/mol () (Mollah et al. Citation2009). The concentration achieved at ring centre was 562 µmol/mol (median). The median daytime concentration of ambient CO2 was 366 µmol/mol.
Figure 1 Major components of the AGFACE carbon dioxide delivery system at Horsham (SE Australia) (courtesy: Mollah et al. Citation2009).
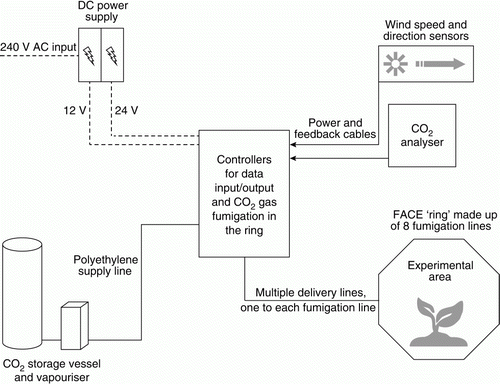
The octagon's fumigation tubes were positioned approximately 150 mm above the crop canopy and parallel to the ground. The 150 mm gap was maintained through the experimental period by raising the tubes at intervals following crop growth. Delivery of CO2 was suspended at night when plants were not photosynthesizing and if the wind speed exceeded 8 m/s during the day.
Brassica trial
Seed of leafy, Asian Brassica cultivars ‘Karate’ (choy sum, Brassica rapa subsp. chinensis) and ‘Chop Suey’ (choy sum, B. rapa subsp. chinensis×tatsoi, B. rapa subsp. narinosa) was sown on 2 March 2010. Seed-lines were sourced from South Pacific Seeds Pty (Griffith, NSW 2680).
Eight plots under eCO2 treatment (i.e. eight, 4 m diameter CO2 delivery ‘rings’=octagons) were established along with eight control ‘plots’. The rows of plants in each ring/plot were orientated north-south with Karate planted in the hemi-ring/plot on the eastern side and Chop Suey in the western half. Direct seeding was deployed rather than use of plug seedlings because ‘plugs’ are already 2–3 weeks old and [CO2] can have a large impact on young plants. Mechanical seed planting delivered a row spacing of approximately 19.5 cm and following hand-thinning, plant spacings were approximately 15 cm; 35 to 40 plants/m2. This planting density was comparable to that used in local commercial agricultural practice.
Soil type at AGFACE is a self-mulching grey vertosol clay (Horsham clay pH 7) (Isbell Citation1966). Low (98 kg N/ha) and high (196 kg N/ha) nitrogen levels based on commercial rates for broccoli (Brassica oleracea) were soil incorporated for both CO2 treated plants and controls (i.e. eCO2: four rings low N and four rings high N; control: four plots low N and four plots high N). The first N application (using mono-ammonium phosphate) occurred pre-sowing on 26 February 2010 with direct drilling to a depth of 5 cm (). Subsequent N applications as urea occurred post-emergence and 16 days prior to harvest ().
Table 1 Schedule for application of low and high nitrogen rates in the Brassica FACE planting.
The 4 m diameter CO2 delivery rings were each positioned centrally within a 5×5 m irrigated zone. Water was supplied through sporadic rainfall and daily overhead irrigation from 90o sprinklers positioned on the four corners of plots. The level of irrigation was varied to take into account natural rainfall levels, ambient temperatures and varying water needs dependent on stage and maturity of plant growth. Irrigation pipes were buried to avoid solar irradiation and the delivery of potentially damaging hot water. Plants in the irrigated zone external (and peripheral) to CO2 delivery rings and equivalent areas in control plots, served to buffer CO2 movement. The supply of CO2 was started 9 days after sowing on 11 March 2010. Seedling emergence was observed on 14 March 2010. During the period 2 March 2010 to 28 April 2010, prevailing weather conditions were typical for the time of year (temperature: average minimum 10 oC, average maximum 25 oC).
Plants were harvested on 28 April 2010, 57 days after sowing. Twenty plants per ring/plot were taken for evaluation. Sampling took place from central parts of rings, at least 30 cm away from CO2 delivery pipes to avoid possible higher concentrations of gas at the outlet source.
In the field, harvested plants (i.e. heads=shoot only) were packed into semi-permeable polyethylene bags and stored in polystyrene containers partially filled with ice. These actions were taken to slow respiration rate and help prevent moisture loss from the leaves. Assessment was laboratory based with samples stored temporarily at 1 oC in the field-packed containers.
Harvested plants were assessed for shoot height above ground, shoot fresh-weight and leaf colour. A subjective scale for leaf colour was used, with rating ‘5’=‘bright green’ through to rating ‘1’=‘yellow’.
Statistics
Plant height and weight data were analysed using a restricted maximum likelihood (REML) algorithm, to determine the relationships between ‘fresh weight’ and ‘plant height’ for each of the other factors, i.e. concentration of CO2 and added N. The REML model had both fixed and random terms, which could be symbolically represented as follows:
All statistical analyses were performed using GenStat Thirteenth Edition (Payne et al. Citation2009).
Results
Harvested plants appeared healthy with an absence of leaf chlorosis, disease and pest infestation in all treatment plots. With respect to general appearance and quality, plants were indistinguishable from commercial crops grown in the main production areas in southern Victoria. The subjective assessment of leaf colour (data not presented) showed no variability within variety that could be attributed to e[CO2] or N levels. Leaves of Chop Suey plants were always darker than those of Karate, but this is a varietal trait that has been observed in commercial plantings.
The amount of N remaining in the soil at harvest was found to be consistent across low and high N treatment plots (0.11 to 0.12 g/100 g). The above ground height of Karate plants was significantly increased by higher N application (196 kg N/ha) (). At eCO2, plants fertilized with 196 kg N/ha were 34% taller than those fertilized with 98 kg N/ha. Similarly, the fresh weight of harvested aerial parts of Karate plants was significantly greater when soil N was higher. Effects of CO2 alone on Karate growth were not significant. However, increase in Karate shoot fresh weights could be attributed to an e[CO2] and high N interaction (P=0.034). Under growing conditions of 196 kg N/ha soil treatment with 562 µmol/mol CO2, Karate plants were produced with a mean fresh weight of 221.6 g; a 110% increase over e[CO2]+98 kg N/ha and a 37% increase over a[CO2]+196 kg N/ha ().
Table 2 Mean shoot system heights and fresh weights of ‘Karate’ and ‘Chop Suey’ plants grown with high or low soil nitrogen under atmospheric (air) or CO2 enriched (FACE) conditions.
Elevated CO2 did not affect the height of Chop Suey plants (). However, Chop Suey fresh weights were significantly increased by growing under e[CO2]. There was also a significant interaction of increased soil N and e[CO2] in promoting Chop Suey fresh weight increase (P=0.087). At 562 µmol/mol e[CO2] and 196 N kg/ha the average fresh weight of Chop Suey heads was 120.9 g. In comparison, when grown under 98 kg N/ha and 366 µmol/mol a[CO2], average fresh weights were 58.2 g, approximately 50% lower.
Discussion
The trend for negative stimulation of fresh weight due to CO2 under the low N treatment (98 kg N/ha) indicates there was insufficient N available for optimal plant growth. Low soil N has been noted as a factor limiting the response of plants to increased CO2 (Ainsworth & Long Citation2005). Different responses from Karate and Chop Suey suggest that there may be a range of genetic variation in N use efficiency that could be selected to improve future response to eCO2.
As in our own work with B. rapa, Uprety & Mahalaxmi (Citation2000) found an interactive effect of CO2 and N nutrition indicating that with the addition of more N, plants sustained the positive effect of CO2 enrichment by utilizing additional carbohydrates. Although we found no effect of CO2 alone on plant height, Uprety & Mahalaxmi (Citation2000) observed that CO2 enrichment not only increased the plant height of B. juncea, but also the number of leaves and leaf area. Nitrogen fertilization increased B. juncea height in both elevated CO2 and ambient atmospheres. Carbon dioxide enrichment increased the dry weights of B. juncea leaves, stems and roots, as did the addition of soil N under 600 ±15 µmol/mol CO2 atmospheres. Uprety & Mahalaxmi (Citation2000) concluded that additional N availability is crucial for rapid growth under high CO2 conditions. Boomiraj et al. (Citation2010) also found that B. juncea was sensitive to changes in CO2 and temperature. In more recent work on Brassica vegetables, La et al. (Citation2009) examined the effects of CO2 enrichment on the growth of Chinese kale (B. alboglabra) treated with three N concentrations (5, 10 and 20 mmol/L). Height, stem thickness and dry weights of the total aerial parts significantly increased as [CO2] was elevated from 350 to 800 µmol/mol at each N concentration. For Chinese kale, results demonstrate that high N supply is also beneficial for growth under higher e[CO2] conditions (La et al. Citation2009).
To exploit projected future increased [CO2] for greater food production and quality, it seems likely that the N fertilizer requirement of Brassica vegetables could be higher than what is required now. Nitrogen recovery from the soil is generally high for Brassica vegetables (Everaarts Citation1993). Maximum N uptake by white cabbage (B. oleracea var. capitata) was around 400 kg/ha (Everaarts & Booij Citation2000). Fortier et al. (Citation2010) observed that yields of marketable broccoli (B. oleracea) heads increased in a curvilinear manner with N rate (0, 105, 135, 165, 195 or 225 kg N/ha), with a tendency to plateau at high rates.
In our trial with B. rapa cultivars, the higher rate at 196 kg N/ha gave greater fresh weights of aerial plant parts. Mean percentage increase in fresh weight across both cultivars at eCO2 under high N was 98% compared with the mean 28% increase under aCO2. This result suggests that to maximize the benefit from future eCO2, soil nitrogen levels will need to match, or possibly exceed, the highest rates currently used for commercial Brassica vegetable production in south-eastern Australia.
Leafy Brassica vegetables are relatively new crops to Victoria and optimum rates for N fertilizer use are not well established for commercial production. Prior to the trial reported here, the FACE site at Horsham had not been used for vegetable production, so that our experimental N rates were based on the site's existing soil N and industry knowledge of fertilizer rates from key commercial broccoli (B. oleracea) production sites in southern Victoria. It appears that we achieved appropriate rates of low N and high N to demonstrate commercial quality across a fertilizer range. However, more importantly, these rates used in conjunction with appropriate watering suitably demonstrated greater productivity (shoot fresh weights) in response to combined increases of N and [CO2] in the growth environment, under field conditions. The two cultivars we tested had differing growth responses to [CO2]. Both irrigation treatments and N fertilization rates were uniform. Thus, we attribute the difference to genetic variance at the sub-species level. Uprety et al. (Citation1998) noted varying photosynthetic and growth responses to CO2 enrichment of related Brassica hybrids. It may be possible to use genetic variation to breed cultivars for more efficient N use under e[CO2] and reduce the need for high rates of added fertilizers.
Conclusion
Future increased levels of atmospheric CO2 could be beneficial and increase the productivity of Brassica vegetable crops even with the high-end soil N levels currently used in commercial production. However, impact on foliar concentrations of proteins and eating quality from a consumer's perspective require evaluation. Long-term experiments under field conditions are also needed to predict the interactive effects of e[CO2] and other ecological variables. Concurrent rises in temperature and declines in water availability with climate change in south-eastern Australia are likely to impact significantly on any gains attributable to CO2 fertilization effects.
Acknowledgements
This research was funded by the Victorian Department of Primary Industries and used AGFACE facilities supported by the Australian Grains Research and Development Corporation.
References
- ABS 2009 . Value of Agricultural Commodities Produced, Australia . Canberra , Australian Bureau of Statistics .
- Ainsworth , EA , Leakey , ADB , Ort , DR and Long , SP . 2008 . FACE-ing the facts: inconsistencies and interdependence among field, chamber and modeling studies of elevated [CO2] impacts on crop yield and food supply . New Phytologist , 179 : 5 – 9 . doi: 10.1111/j.1469-8137.2008.02500.x
- Ainsworth , EA and Long , SP . 2005 . What have we learned from 15 years of free-air CO2 enrichment (FACE)? A meta-analytic review of the responses of photosynthesis, canopy properties and plant production to rising CO2 . New Phytologist , 165 : 351 – 372 . doi: 10.1111/j.1469-8137.2004.01224.x
- Allen , LH , Drake , BG , Rogers , HH and Shinn , JH . 1993 . “ Field techniques for exposure of plants to CO2 ” . In Free-air CO2 enrichment for plant research in the field , Edited by: Hendrey , GR . Boca Raton , Florida : CRC Press .
- Amthor , JS . 2001 . Effects of atmospheric CO2 concentration on wheat yield: review of results from experiments using various approaches to control CO2 concentration . Field Crops Research , 73 : 1 – 34 . doi: 10.1016/S0378-4290(01)00179-4
- Baxter , R , Ashenden , TW and Farrar , JF . 1994 . Effects of elevated carbon dioxide on three grass species from montane pasture. II. Nutrient uptake, allocation and efficiency of use . Journal of Experimental Botany , 45 : 1267 – 1278 . doi: 10.1093/jxb/45.9.1267
- Bazzaz , FA . 1990 . The response of natural ecosystems to the rising global CO2 levels . Annual Review of Ecology and Systematics , 21 : 167 – 196 . doi: 10.1146/annurev.es.21.110190.001123
- Boomiraj , K , Chakrabarti , B , Aggarwal , PK , Choudhary , R and Chander , S . 2010 . Assessing the vulnerability of Indian mustard to climate change . Agriculture, Ecosystems and Environment , 138 : 265 – 273 . doi: 10.1016/j.agee.2010.05.010
- Bowes , G . 1993 . Facing the inevitable: plants and increasing atmospheric CO2 . Annual Review of Plant Biology , 44 : 309 – 332 . doi: 10.1146/annurev.pp.44.060193.001521
- Carter TR , Jones RN , Lu X , Bhadwal S , Conde C , Mearns LO et al. 2007 . New assessment methods and the characterisation of future conditions . Climate Change 2007: Impacts, adaptation and vulnerability. Contribution of Working Group II to the Fourth Assessment Report of the Intergovernmental Panel on Climate Change . Parry ML , Canziani OF , Palutikof JP , van der Linden PJ , Hanson CE Cambridge University Press , Cambridge , , UK . Pp. 133 – 171 .
- Cotrufo , MF , Ineson , P and Scott , A . 1998 . Elevated CO2 reduces the nitrogen concentration in plant tissues . Global Change Biology , 4 : 43 – 54 . doi: 10.1046/j.1365-2486.1998.00101.x
- Cure , J and Acock , B . 1986 . Crop responses to carbon dioxide doubling: a literature survey . Agricultural and Forest Meteorology , 38 : 127 – 145 . doi: 10.1016/0168-1923(86)90054-7
- Epron , D , Liozon , R and Mousseau , M . 1996 . Effects of elevated CO2 concentration on leaf characteristics and photosynthetic capacity of beech (Fagus sylvatica) during the growing season . Tree Physiology , 16 : 425 – 432 . doi: 10.1093/treephys/16.4.425
- Erickson , J . 1993 . From ecology to economics: the case against CO2 fertilisation . Ecological Economics , 8 : 157 – 175 . doi: 10.1016/0921-8009(93)90042-5
- Everaarts , AP . 1993 . General and quantitative aspects of nitrogen fertiliser use in the cultivation of Brassica vegetables . Acta Horticulturae , 339 : 149 – 160 .
- Everaarts , AP and Booij , R . 2000 . The effect of nitrogen application on nitrogen utilisation by white cabbage (Brassica oleracea var. capitata) and on nitrogen in the soil at harvest . Journal of Horticultural Science and Biotechnology , 75 : 705 – 712 .
- Fortier , E , Desjardins , Y , Tremblay , NA , Bélec , C and Côté , M . 2010 . Influence of irrigation and nitrogen fertilisation on broccoli polyphenolics concentration . Acta Horticulturae (ISHS) , 856 : 55 – 62 .
- Hendrey , GR , Ellsworth , DS , Lewin , KF and Nagy , J . 1999 . A free-air enrichment system for exposing tall forest vegetation to elevated atmospheric CO2 . Global Change Biology , 5 : 293 – 309 . doi: 10.1046/j.1365-2486.1999.00228.x
- Hovenden , MJ , Miglietta , F , Zaldei , A , Vander Schoor , JK , Wills , KE and Newton , PCD . 2006 . The TasFACE climate-change impacts experiment: design and performance of combined elevated CO2 and temperature enhancement in a native Tasmanian grassland . Australian Journal of Botany , 54 : 1 – 10 . doi: 10.1071/BT04194
- IPCC (Intergovernmental Panel on Climate Change) 1997 . Special report: The regional impacts of climate change—an assessment of vulnerability . Watson R , Zinyowera M , Moss R eds. Cambridge University Press , Cambridge , , UK . Pp. 517 .
- IPCC (Intergovernmental Panel on Climate Change) 2007 . Climate change 2007: the physical science basis . Contribution of Working Group I to the Fourth Assessment Report of the Intergovernmental Panel on Climate Change . Solomon S , Qin D , Manning M , Chen Z , Marquis M , Averyt KB , Tignor M , Miller HL eds. Cambridge University Press . 996 p.
- Isbell , RF . 1966 . The Australian Soil Classification , Melbourne : CSIRO Publishing .
- Jablonski , LM , Wang , X and Curtis , PS . 2002 . Plant reproduction under elevated CO2 conditions: ameta-analysis of reports on 79 crop and wild species . New Phytologist , 156 : 9 – 26 . doi: 10.1046/j.1469-8137.2002.00494.x
- Karowe , DN , Seimens , DH and Mitchell-Olds , T . 1997 . Species-specific response of glucosinolate content to elevated atmospheric CO2 . Journal of Chemical Ecology , 23 : 2569 – 2582 . doi: 10.1023/B:JOEC.0000006667.81616.18
- La , G , Fang , P , Teng , Y , Li , Y and Lin , X . 2009 . Effect of CO2 enrichment on the glucosinolate contents under different nitrogen levels in bolting stem of Chinese kale (Brassica alboglabra L.) . Journal of Zhejiang University: Science B , 10 : 454 – 464 . doi: 10.1631/jzus.B0820354
- Long , SP , Ainsworth , EA , Rogers , A and Ort , DR . 2004 . Rising atmospheric carbon dioxide: Plants FACE the future . Annual Review of Plant Biology , 55 : 591 – 628 . doi: 10.1146/annurev.arplant.55.031903.141610
- Meehl GA , Stocker TF , Collins WD , Friedlingstein P , Gaye AT , Gregory JM et al. 2007 . Global climate projections (Chapter 10) . In : Climate Change 2007: The Physical Science Basis . Contribution of Working Group I to the Fourth Assessment Report of the Intergovernmental Panel on Climate Change . Solomon S , Qin D , Manning M , Chen Z , Marquis M , Averyt KB , Tignor M , Miller HL eds. Cambridge, UK and New York, US , Cambridge University Press , Cambridge , , UK and New York, US . 747 – 845 .
- Miglietta , F , Peressotti , A , Primo Vacari , F , Zaldei , A , De Angelis , P and Scarscia Mugnozza , G . 2001 . Free-air CO2 enrichment of a poplar plantation: the POPFACE fumigation system . New Phytologist , 150 : 465 – 476 . doi: 10.1046/j.1469-8137.2001.00115.x
- Mollah , MR , Norton , RM and Huzzey , J . 2009 . Australian grains free air carbon dioxide enrichment (AGFACE) facility: design and performance . Crop and Pasture Science , 60 : 697 – 707 . doi: 10.1071/CP08354
- Okada , M , Lieffering , M , Nakamura , H , Yoshimoto , M , Kim , HY and Kobayashi , K . 2001 . Free-air enrichment (FACE) using pure CO2 injection: system description . New Phytologist , 150 : 251 – 260 . doi: 10.1046/j.1469-8137.2001.00097.x
- Payne RW , Murray DA , Harding SA , Baird DB , Soutar DM 2009 . GenStat for Windows Introduction , (13th edition) Hemel Hempstead , UK, VSN International .
- Saralabai , VC , Vivekanandan , M and Suresh Babu , R . 1997 . Plant responses to high CO2 concentration in the atmosphere . Photosynthetica , 33 : 7 – 37 . doi: 10.1023/A:1022118909774
- Steffen , W and Canadell , P . 2005 . Carbon dioxide fertilisation and climate change policy , Canberra : Australian Greenhouse Office, Australian Government Department of the Environment and Heritage. 33 p .
- Tubiello , F , Amthor , J , Boote , K , Donatelli , M , Easterling , W Fischer , G . 2007 . Crop response to elevated CO2 and world food supply . European Journal of Agronomy , 26 : 215 – 223 . doi: 10.1016/j.eja.2006.10.002
- Uprety , DC . 1998 . Carbon dioxide enrichment technology: Open top chambers a new tool for global climate research . Journal of Scientific and Industrial Research , 57 : 266 – 270 .
- Uprety , DC , Dwivedi , N and Mohan , R . 1998 . Characterisation of CO2 responsiveness in a Brassica oxycamp interspecific hybrid . Journal of Agronomy and Crop Science , 180 : 7 – 13 . doi: 10.1111/j.1439-037X.1998.tb00362.x
- Uprety , DC and Mahalaxmi , V . 2000 . Effect of elevated CO2 and nitrogen nutrition on photosynthesis, growth and carbon-nitrogen balance in Brassica juncea . Journal of Agronomy and Crop Science , 184 : 271 – 276 . doi: 10.1046/j.1439-037x.2000.00392.x
- Weier TE , Stocking CR , Barbour MG 1974 . New York : Wiley and Sons . Botany: an introduction to plant biology 5th edition. . 693 p.
- Wittwer S 1992 . Rising carbon dioxide is great for plants . Policy Review, Autumn 1992. www.purgit.com/co2ok.html (accessed March 2008) .
- Xu , ZG , Walklate , PJ and McLeod , AR . 1997 . Numerical study of a full-size free-air fumigation system . Agricultural and Forest Meteorology , 85 : 159 – 170 . doi: 10.1016/S0168-1923(96)02413-6