ABSTRACT
We previously reported that the acid invertase gene PpVIN2 appears to be induced by chilling and may play an important role in sucrose metabolism in peach fruit subjected to cold storage. We also hypothesised that an invertase inhibitor (INH) affects vacuolar acid invertase (VIN) activity at the post-translational level. To determine which INH gene correlates with VIN activity, five INH genes (designated PpINH1–5) were identified and cloned from ‘Yulu’ peach. Relative expression for each gene was then measured in ‘Yulu’ peaches stored at 0, 5, 10, and 20°C and in ‘Hujingmilu’ peaches stored at 5°C. Each INH gene contains four conserved cysteine residues and four asymmetric alpha-helices characteristic of inhibitor protein. PpINH1–4 are members of the INH family while PpINH5 is classified as a pectin methylesterase inhibitor (PMEI). Transcript analyses showed that the PpINHs in ‘Yulu’ peaches have lower expression during storage at 5°C vs. 10°C, indicating that they are not low temperature-induced genes. However, PpINH1 expression is negatively correlated with VIN activity in both ‘Yulu’ and ‘Hujingmilu’ peaches stored at 5°C, suggesting PpINH1 is involved in the post-translational regulation of VIN under chilling conditions.
Introduction
Invertase (β-fructofuranosidase; EC 3.2.1.26), the key enzyme in sucrose metabolism, irreversibly hydrolyses sucrose into glucose and fructose, and plays a major role in plant development and in responses to biotic and abiotic stresses (Jutta et al. Citation2008). Three distinct invertases are found in higher plants. Two are classified as acid invertases (AI) and are linked to the cell wall (CWIN) or are localised in the vacuole (VIN). AI has several important functions, such as regulating phloem sugar unloading, controlling sugar composition in storage organs, and modulating early plant growth and signal transduction (Dickinson et al. Citation1991; Stitt et al. Citation1991; Roitsch et al. Citation2003; Weber et al. Citation1996). Strong correlations are found between AI activity and the hexose:sucrose ratio in cold-stored potatoes from a range of cultivars (Mckenzie et al. Citation2005; Ye et al. Citation2010; Wu et al. Citation2011). In Japanese pears, PpAIV2 expression affects sucrose degradation during cold storage (Itai et al. Citation2015). Regulation of AI occurs at the transcriptional and post-translational levels following various stimuli such as changes in hexose concentration or in the environment (Yun et al. Citation2002; Roitsch et al. Citation2003; Huang et al. Citation2007). Since apoplasmic and vacuolar invertases are intrinsically stable enzymes due to their glycan decoration, control of their activity may be highly dependent on post-translational mechanisms (Greiner et al. Citation2000; Rausch and Greiner Citation2004; Tauzin et al. Citation2014). Recent studies suggest that invertase activity is subject to post-translational suppression by endogenous proteinaceous inhibitors (INHs) (Greiner et al. Citation1999; Hothorn et al. Citation2004; Paynel et al. Citation2014). The formation of the invertase inhibitor complex may be an important mechanism for the control of invertase activity in vivo, subsequently affecting carbon partitioning and fruit development (Fridman et al. Citation2004; Hothorn et al. Citation2010). Cold-induced sweetening resistance is partly due to post-translational inhibition of AI activity by the VIN inhibitor INH2 (Mckenzie et al. Citation2013). In tomatoes, overexpression of the invertase inhibitor Sly-INH reduces CWIN activity, suggesting that Sly-INH regulates CWIN activity at the posttranslational level (Zhang et al. Citation2015) and plays an important role in chilling tolerance (Xu et al. Citation2017).
The first plant INH was identified in potatoes (Schwimmer et al. Citation1961). Homologs were subsequently identified in red beets, sugar beets, sweet potatoes and maize (Jaynes and Nelson Citation1971; Kursanov et al. Citation1971). Ectopic expression of the tobacco VIN inhibitor in potatoes also reduced VIN activity and blocked hexose accumulation (Greiner et al. Citation1999). INHs are classified as pectin methylesterase inhibitors (PMEIs) (Hothorn et al. Citation2004) and Kunitz-type protease inhibitor (Glaczinski et al. Citation2002). A potato Kunitz-type protease inhibitor gene, St-inh, inhibits invertase and impacts potato cold-induced sweetening (Cheng et al. Citation2004). INHs are classified by subcellular localisation into cell wall inhibitors (CIFs) and vacuolar inhibitors (VIFs) (Rausch and Greiner Citation2004). INHs are low molecular weight proteins (17–23 kDa) that bind to invertase to form inactive complexes. For example, StInvInh2 interacts with StInv1 and inhibits its activities, thereby contributing to the accumulation of reducing sugars in cold-stored potato tubers (Liu et al. Citation2010; Lin et al. Citation2013). SlVIF and SlVI have been investigated in tomatoes (Tauzin et al. Citation2014) and are now known to physically interact with each other to control sucrose metabolism (Qin et al. Citation2016).
Peaches are climacteric fruit and have a short shelf life when stored at ambient temperature. Cold storage is a commercial method to slow ripening (Colaric et al. Citation2005), but peaches exposed to low but nonfreezing temperatures are prone to chilling injury (CI) and loss of food value (Valero et al. Citation1997). Severe CI occurs during storage at 5°C for 3 weeks, but is absent in peaches stored at 0°C or 10°C (Wang et al. Citation2013; Yu et al. Citation2015). As the predominant sugar in the ripening process, sucrose is not only closely correlated with overall taste and aroma (Colaric et al. Citation2005), but also affects the quality and chilling tolerance of postharvest peach fruit (Wang et al. Citation2013; Yu et al. Citation2016). When severe CI occurs in peaches stored at 5°C, it is accompanied by decreased sucrose levels. We recently demonstrated that VIN activity rises rapidly in peaches after 14 days of storage, and found a significant negative correlation with sucrose content during cold storage at 5°C (He et al. Citation2018). In addition, among seven AI genes detected in peaches, the VIN gene (PpVIN2) had the highest transcript level and only its expression was strongly elevated by low-temperature stress, suggesting that the gene is induced by chilling and may play an important role in sucrose metabolism in fruit subjected to cold storage.
In this work, we cloned the invertase inhibitor genes of peach, subjected them to DNA sequencing, and predicted their physicochemical properties. Expression of the INH genes was examined in ‘Yulu’ peaches stored at 0°C, 5°C, 10°C, and 20°C and in ‘Hujingmilu’ peaches stored at 5°C. The relationship between VIN activity and PpVIN2 expression in ‘Yulu’ and ‘Hujingmilu’ peaches stored at 5°C was also established. The results of this study provide a foundation for further research on the mechanism by which these genes products inhibit AI and affect sucrose accumulation.
Materials and methods
Fruit and storage
The experimental materials were the commercial mature peaches (Prunus persica L. Batsch cv. ‘Yulu’ and ‘Hujingmilu’) fruits harvested from an orchard in Fenghua, Zhejiang Province, China in 2016 and 2018, which are transported to our laboratory immediately after picking. Selected fruit were of similar size and maturity, had a uniform appearance, and were free of defects. ‘Yulu’ fruits (collected in 2016) were randomly divided into four groups, stored at 0°C, 5°C, 10°C, and 20°C, with constant relative humidity from 92% to 95%. Fruits in the 0°C, 5°C, and 10°C groups were stored for 4 weeks and sampled weekly. However, Fruits in the 20°C group were stored for 12 days and were sampled every 2 days. Besides, ‘Hujingmilu’ fruits (collected in 2018) were stored at 5°C for 4 weeks and sampled weekly.
The mesocarp (about 1 cm thick) was sectioned and frozen in liquid nitrogen, and stored at –80°C for later analysis. Three replicates, each consisting of fifteen fruits at each time point, were used for biochemical and molecular analysis.
Cloning of putative INH genes and bioinformatic analyses
RNA extraction and cDNA synthesis
Total RNA was extracted from frozen tissue samples using the TIANGEN RNAprep Pure Plant Kit (Polysaccharides and Polyphenolics-rich) (TIANGEN, Beijing, China) according to the manufacturer's instructions. RNA integrity was confirmed by 1% agarose gel electrophoresis and ethidium bromide staining. RNA was quantified by measuring absorbance at 260 nm using a spectrophotometer (Nanodrop 2000, Thermo Scientific, USA), and purity was assessed by calculating the ratio of absorbance at 260/280 nm. cDNA for real-time RT–PCR was synthesised using HiScript® Q RT SuperMix containing gDNA wiper (Vazyme, Nanjing, China). cDNA samples were stored at −40°C.
Identification and cloning of INH genes
To identify members of the INH gene family in peaches, the keywords ‘acid invertase inhibitor' and ‘prunus perscia' were used as queries against the NCBI database collection, and five genes were identified. Gene specific primers () were designed using NCBI-PRIMER (http://www.ncbi.nlm.nih.gov/tools/primer-blast). cDNA synthesis and QPCR implementation referred to He et al. (Citation2018).
Table 1. INH Primers for PCR amplification.
Bioinformatic analyses
Protein molecular weights and isoelectric points were predicted using Compute pI/Mw (http://www.expasy.org/tools/pi_tool.html). The signal peptide, putative cleavage site, and putative glycosylation sites were predicted using the Signal P 3.0 Server (www.cbs.dtu.dk/services/SignalP-3.0), NetNGlyc 1.0 server, (http://www.cbs.dtu.dk/services/NetNGlyc), and YinOYang server (http://www.cbs.dtu.dk/services/YinOYang/). TMHMM (http://www.cbs.dtu.dk/services/THMHM) was used to identify putative transmembrane regions. Multi-sequence alignment was conducted using TCOFFEE-Expresso (http://tcoffee.crg.cat) and the results were refined with Jalview (version 2.10.5). Conserved domains were predicted using MEME (http://meme-suite.org/tools/meme). Jpred4 (www.compbio.dundee.ac.uk/jpred4/) and GOR IV (http://npsa-prabi.ibcp.fr/cgi-bin/npsa_automat.pl?page=npsa_gor4.html) were used together to predict protein secondary structure. Phylogenetic trees were constructed using the neighbor-joining method with MEGA X. The fraction (percentage) of replicate trees in which associated taxa were clustered in the bootstrap test (for 1000 replicates) is shown at the branch points (Felsenstein Citation1985). The tree was visualised using TREEVIEW (version 1.6.6).
Quantitative real-time PCR (qRT-PCR) analysis of INH gene expression
Relative quantitative real-time RT–PCR was performed in triplicate with the Roche LightCycler®96 Real Time PCR System and the LightCycler®96 SW1.1 analysis software. To normalise gene expression levels (to eliminate differences in the efficiency of cDNA synthesis), translation elongation factor 2 (TEF2, JQ732180.1) was chosen as the reference gene (Wang et al. Citation2013). Reactions were prepared in a total volume of 20 μL containing 10 μL 2×ChamQ Universal SYBR qPCR Master Mix (Vazyme, Nanjing, China), 2 μL template cDNA, 0.4 μL of each primer (10 mM), and 7.2 μL of RNase free water. Blank controls were run in triplicate for each master mix. The cycling conditions were as follows: initial denaturation at 95°C for 30 s, followed by 40 cycles at 95°C for 10 s and 60°C for 30 s. Baseline and Cycle threshold were automatically determined using the LightCycler®96S W1.1 software. The amplification primers were designed using the NCBI-PRIMER (https://www.ncbi.nlm.nih.gov/tools/primer-blast/) as shown in . Primer specificity was verified by melting curve analysis and gel electrophoresis. Primer amplification efficiency, calculated using serial dilutions of cDNA, was between 95% and 105%. At least three biological replicates were analysed for each cDNA. Relative gene expression was calculated using the comparative 2–ΔΔCt method (Livak and Schmittgen Citation2001; Kou et al. Citation2018).
Table 2. Primers of peach invertase gene and its inhibitor genes used for real-time fluorescence quantitative PCR.
VIN extraction and measurement of activity
VIN was extracted as described by Schaffer et al. (Citation1989) with modifications. Frozen peach tissue (1 g) was homogenised in 5 mL of extraction buffer including 100 mM phosphate buffered saline, 5 mM MgCl2, 2.5 mM DL-dithiothreitol, 1 mM ethylenediaminetetraacetic acid, and 0.1% (v/v) Tritonx-100. The homogenates were centrifuged at 10,000 × g for 20 min at 4°C and supernatants were dialysed in 0.1× extraction buffer (without Tritonx-100) to remove soluble sugars. VIN activity was assayed in a reaction containing 100 mM sodium citrate buffer (pH 4.5), 1% (m/v) sucrose, and crude enzyme extract. The mixture was incubated at 37°C for 30 min, and then placed in a boiling water bath for 5 min to stop the reaction. The activities were determined by measuring the amount of reducing sugar produced from sucrose. One unit of VIN activity was defined as the amount of enzyme required to produce 1 μmol glucose per hour, expressed as U/g fresh weight (FW). The glucose content was determined using 3,5-dinitrosalicylic acid.
Statistical analysis
Experiments were conducted using a completely randomised design. Statistical analyses were performed using SAS (version 8.2, SAS Institute, Cary, NC, USA). Pearson's correlation was used to determine the relationship between expression of INH genes and VIN activity. Differences between means were evaluated using ANOVA analysis. Differences for which p < 0.05 were considered significant. Figures were composed using OriginPro 2017C (Microcal Software Inc., Northampton, MA, USA). The standard errors (SEs) of the means are presented in the graphs as error bars.
Results
Cloning and characterisation of INH genes
Five INH genes (GenBank Accession No. XM_007208838, XM_007217302, XM_007223513, XM_007223389, XM_007209598) were isolated from peach and designated PpINH1–5. The genes are listed in , along with several predicted physical characteristics for the proteins they encode, including lengths, isoelectric points, and molecular weights. Two potential O-glycosylation sites were identified in PpINH1. PpINH3 also appears to contain a signal peptide at the N-terminus, with a putative cleavage site between Cys25 and Ser26 and the cleavage site is between the two N-glycosylation sites. Similarly, PpINH5 contains an N-terminal signal peptide whose putative cleavage site is local between Gly24 and Ser25 and the cleavage site is between the two N-glycosylation sites. Finally, PpINH4 has a transmembrane structure at the N-terminus and four N-glycosylation sites.
Table 3. Invertase inhibitor genes and their deduced amino acid in peaches.
An amino acid alignment for the five proteins is shown in . Although the proteins exhibit many differences, they each contain four conserved cysteine residues that form disulphide bridges in all functional INHs. MEME analysis suggests that PpINH5 is most likely a pectin methylesterases inhibitor (PMEI), while the other four proteins are invertase inhibitors that associate with cell walls (CIF).
Figure 1. Alignment of deduced amino acid sequences of inhibitor genes in peaches. Regions with amino acid identity are marked with different colours. The four conserved cysteine residues are indicated by crosses at the top of the alignment.
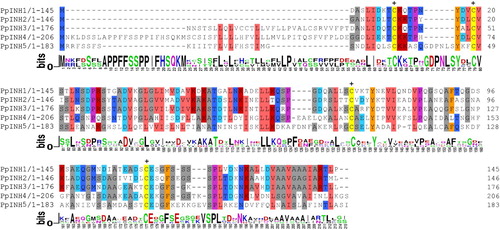
A phylogenetic tree was constructed using the five peach sequences, as well as functionally characterised INHs and PMEIs from other species (). The proteins form two high-level groups that can each be divided into two sub-groups. Subgroup I is composed of seven proteins from the Solanaceae (SlInvInh1, CAA09420; StInvInh1, ADM49012; NtInvInh1, CAA73333; StInvInh3, ADM49013; NtInvInh2, CAA73334; StInvInh2A, ADM49015; and StInvInh2B, ADM49016). Functional invertase inhibitors from A. thaliana and Z. mays cluster into subgroups II and III. PpINH1–4 are found in subgroup II, which also contains AtVIF1. Within subgroup II, PpINH1–3 are more closely related to each other than to PpINH4. Finally, PpINH5 occupies subgroup IV, accompanied by other PEI proteins such as AtPMEI1, AtPMEI2, and AdPMEI1.
Figure 2. Evolutionary relationship between 18 genes from the PMEI-RP family. The tree was constructed using sequences from 7 different species, including peach. The two main groups are indicated using a dashed line, and the subgroups are identified using Roman numerals. Asterisks (*) indicate invertase inhibitors that have been characterised experimentally. The genes from peach (PpINH1–5) are shown in boxes. The other InvInh gene sequences are cDNAs from potato (StInvInh1, ADM49012; StInvInh2A, ADM49015; StInvInh2B, ADM49016; StInvInh3, ADM49013), tobacco (NtInvInh1, CAA73333; NtInvInh2, CAA73334), tomato (SlInvInh1, CAA09420), Arabidopsis thaliana (AtInvInh1, NP_564516; AtInvInh2, NP_201267; AtPMEI1, NP_175236; AtPMEI2, NP_188348), Zea mays (ZmINH1, ATT24363), and Kiwi (AdPMEI1, BAC54964).
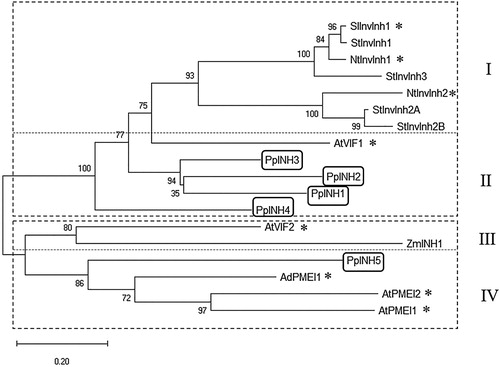
Prediction of the secondary structure of invertase inhibitor proteins
Predictive analysis of protein secondary structure indicated that PpINH1 alpha helix contains 52 amino acids, accounting for 35.86%; extended strand contains 11 amino acids, accounting for 7.59%; random coil contains 82 amino acids, accounting for 56.55%. PpINH2 alpha helix contains 64 amino acids, accounting for 43.84%; extended strand contains 20 amino acids, accounting for 13.70%; random coil contains 62 amino acids, accounting for 42.47%. Four conserved Cys are located on the 1, 2, 4 and 6 α-helices (PpINH1 and PpINH2). PpINH3 alpha helix contains 50 amino acids, accounting for 28.41%; extended strand contains 24 amino acids, accounting for 13.64%; random coil contains 102 amino acids, accounting for 57.95%. PpINH4 alpha helix contains 68 amino acids, accounting for 33.01%; extended strand contains 27 amino acids, accounting for 13.11%; random coil contains 111 amino acids, accounting for 53.88%. PpINH5 alpha helix contains 84 amino acids, accounting for 45.90%; extended strand contains 22 amino acids, accounting for 12.02%; random coil contains 77 amino acids, accounting for 42.08%. Four conserved Cys are located on the 2, 3, 5 and 7 α-helices (PpINH3-5). The secondary structure of INH proteins shows that PpINH1 and PpINH2 have a similar secondary structure while PpINH3, PpINH4 and PpINH5 possess the analogous structure.
Relative expression of PpINH genes in ‘Yulu’ peaches stored at four different temperatures
Expression levels for the five PpINH genes were compared in ‘Yulu’ fruit stored at different temperatures for 28 days (). At 0°C, expression of PpINH1–4 was remarkably stable until day 14, and expression levels were lower than at any other temperature during this period. In contrast, the expression of these four genes was more variable at higher temperatures. For example, at 5°C expression of PpINH1, 2 and 4 increased modestly on days 7 and 14. At 10°C, expression of PpINH1–4 increased and then declined, with PpINH2–4 showing particularly dramatic shifts. PpINH1, 2 and 4 at 20°C also exhibited rapid increases followed by rapid declines. PpINH5 expression had a distinctly different profile in comparison with the patterns generated by the other genes. Although its expression levels were relatively stable at most temperatures, expression of PpINH5 increased sharply at 0°C, late in storage.
Figure 3. Relative expression of INH genes in peach fruit (‘Yulu’) stored at four different temperatures. (A: 0°C, B: 5°C, C: 10°C, D: 20°C). Values are the means ± SE of triplicate assays. Different letters indicate a significant difference (P < 0.05) between 0°C, 5°C, and 10°C using Duncan's multiple range test at each time point.
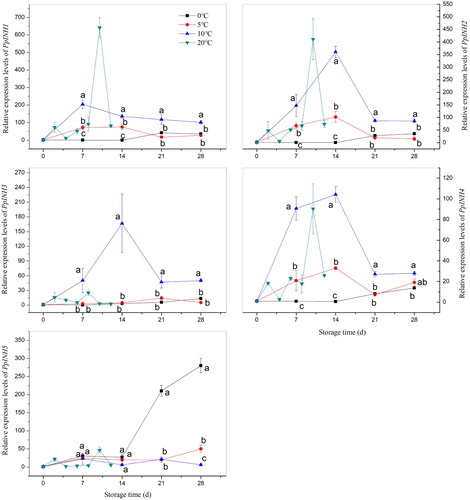
Relative expression of PpINH genes in ‘Yulu’ and ‘Hujingmilu’ peaches during storage at 5°C
As shown in , expression of PpINH1–4 was similar in both cultivars. The expression level of PpINH1 gene in ‘Yulu’ was significantly higher than that in ‘Hujingmilu’. In general, the expression for these genes first increased and then decreased during storage. In contrast, PpINH5 expression trended upward throughout storage. Among the five inhibitors, PpINH3 had the lowest expression levels. Compared with ‘Hujingmilu’, the PpINH1 expression of ‘Yulu’ were significantly higher except 21th day. However, for PpINH2-5 ‘Yulu’ only showed significantly higher value at 14th of PpINH2, 21th of PpINH3, 28th of PpINH3, 7th and 28th of PpINH5.
Figure 4. Relative expression of INH genes in peach fruit (‘Yulu’ and ‘Hujingmilu’) stored at 5°C. (A: PpINH1, B: PpINH2, C: PpINH3, D: PpINH4, E: PpINH5). Values are the means ± SE of triplicate assays. Different letters indicate a significant difference (P < 0.05) using Duncan's multiple range test.
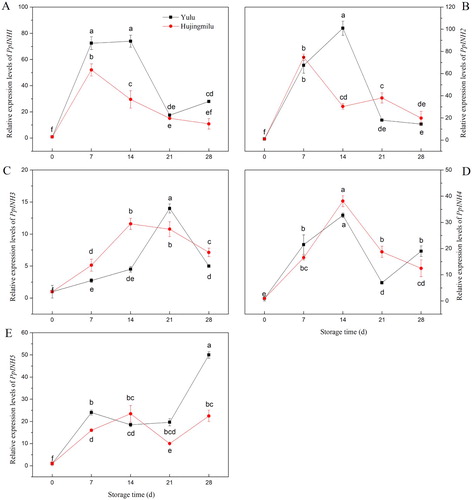
VIN activity and relative expression of PpVIN2 in ‘Yulu’ and ‘Hujingmilu’ peaches during storage at 5°C
‘Yulu’ and ‘Hujingmilu’ peaches were stored at 5°C for 28 days. Over this period, VIN activity increased approximately two-fold in ‘Yulu’ fruit, while no significant change was observed in ‘Hujingmilu’ fruit (A). At all times, VIN activity was lower in ‘Hujingmilu’. In contrast, expression of PpVIN2 increased markedly in both cultivars over the same period, reaching levels approximately 250-fold higher than initial values on day 21 (B).
Relationship between INH gene expression and VIN activity
Inhibitor gene expression and VIN activity, measured in ‘Yulu’ and ‘Hujingmilu’ peaches during storage at 5°C, were compared using a Pearson correlation analysis. As shown in , PpINH1 expression correlated negatively with VIN activity in ‘Yulu’ (r = −0.9743, p = 0.0257) and ‘Hujingmilu’ (r = −0.8912, p = 0.0424) fruit. No significant correlation was observed between the expression of any other inhibitor gene and VIN activity.
Table 4. Pearson's Correlation between the PpINHs expression and VIN activity of ‘Yulu’ and ‘Hujingmilu’ peaches stored at 5°C.
Discussion
Previous research in our laboratory demonstrated that VIN activity is negatively correlated with sucrose content, suggesting that VIN activity plays an important role in sucrose metabolism and chilling injury in postharvest ‘Yulu’ and ‘Baifeng’ peach during cold storage at 5°C (He et al. Citation2018). Furthermore, PpVIN2 expression affects VIN activity and indirectly promotes sucrose degradation (He et al. Citation2018). Although PpVIN2 expression and VIN activity are correlated, the increase in PpVIN2 expression is disproportionate to the increase in VIN activity. The discrepancy implies that invertase activity is subject to posttranslational regulation, as well as by specific invertase inhibitors.
INH genes have been identified in numerous plant species (Weil et al. Citation1994; Greiner et al. Citation1999; Bate et al. Citation2004; Brummell et al. Citation2011; Qin et al. Citation2016; Shivalingamurthy et al. Citation2018). In this study, five putative invertase inhibitor genes were cloned from peach (cv. ‘Yulu’) and molecularly characterised. The encoded proteins contain two disulphide bridges and are mainly alpha-helical, which are characteristics seen in other invertase inhibitor proteins (Camardella et al. Citation2010). However, the primary amino acid sequences of INH proteins from different species are poorly conserved, even amongst proteins from the same species. For example, the two INH genes from tobacco are 47% similar (Greiner et al. Citation1998, Citation1999), the two genes from A. thaliana are 29% similar (Link et al. Citation2004), and similarities amongst members of the PMEI-RP family ranged from 20 to 35% (Rausch and Greiner Citation2004). In our study, the five INH genes exhibited similarities of 25% (PpInvInh1 vs. PpInvInh5) to 65% (PpInvInh1 vs. PpInvInh3). The substantial differences in structure suggest that each INH has a distinct role.
In our previous study, out of seven AI genes examined, only PpVIN2 was sensitive to chilling stress at 5°C. Expression of this gene sharply increased during the first 2 weeks of storage, and was significantly higher than in fruits stored at other temperatures (0°C, 10°C and 20°C) (He et al. Citation2018). However, in the present study, expression of INHs was lower at 0°C and 5°C than at 10°C and 20°C (). Although a sharp increase in PpINH5 expression occurred late in storage, it was observed only at 0°C, suggesting that PpINHs is not responsive to chilling stress.
Some reports suggest that INHs affect sugar metabolism by regulating the activity of AI. Overexpression of the StInvInh2 vacuolar invertase inhibitor gene in cold-induced sweetening-susceptible potato tubers reduces AI activity and the accumulation of reducing sugars (Mckenzie et al. Citation2013). Overexpression of SlVIF in tomato reduces VIN activity, whereas silencing its expression increases VIN activity, indicating that invertase activity is regulated by an endogenous inhibitor (Qin et al. Citation2016). In soybean, silencing of the GmCIF1 inhibitor increased CWIN activity and modifies sucrose metabolism (Tang et al. Citation2016). In this study, a Pearson correlation analysis indicated that PpINH1 expression correlates negatively with VIN activity in ‘Yulu’ and ‘Hujingmilu’ peaches during storage at 5°C, supporting the hypothesis that PpINH1 regulates VIN via a post-translational mechanism. Besides, we found that the expression of PpINH1 at 5°C in ‘Yulu’ was significantly higher than that in ‘Hujingmilu’ while the others did not. Therefore, we speculate that PpINH1 may regulate the activity of PpVIN2 at the post-translational level. The interaction between StInv1 and StInvInh2 may control the cold-induced sweetening through post-translational regulation of StInv1 by StInvInh2 in potato tubers (Liu et al. Citation2010). In Dendrobium officinale, expression of DoInvInh1 and DoInvInh3 correlated negatively with CWIN activity, suggesting that these genes regulate CWIN activity (Miao et al. Citation2018).
INH proteins are hypothesised to regulate invertase activity at the post-translational level through protein–protein interactions (Rausch and Greiner Citation2004). In potato, Kunitz-type protein inhibitors were captured by StvacINV1 in a yeast two-hybrid screen of two cDNA libraries (Liu et al. Citation2011), and the interaction between them was confirmed using a bimolecular fluorescence complementation assay in tobacco cells (Lin et al. Citation2013). In tomato, a yeast two-hybrid assay demonstrated that SlVIF interacts with SlVI to suppress SlVI activity. Specific interactions between SolyVIF and TIV-1 were investigated by an enzymatic approach and surface plasmon resonance (Tauzin et al. Citation2014), and other studies have also shown that the identified interaction between the serine protease inhibitor KTI4 and the vacuolar protease SlVPE3 mediates the maturation and disease resistance in tomato fruit (Wang et al. Citation2017). Additionally, the same methods were also used to probe the interaction between a CIF from N. tabacum and a CWIN from A. thaliana (Hothorn et al. Citation2010).
Conclusions
In this study, five INH genes (PpINH1–5) were identified in peaches and cloned from the ‘Yulu’ cultivar. PpINH1–4 are INH proteins, while PpINH5 is classified as a PMEI. None of the five INH genes are low-temperature induced. In contrast to the other four inhibitor genes, expression of PpINH1 is negatively correlated with VIN activity in ‘Yulu’ and ‘Hujingmilu’ peaches stored at 5°C, suggesting that PpINH1 is involved in the post-translational regulation of VIN. Our results provide a foundation for exploring interactions between PpINH1 and VIN in future experiments.
Disclosure statement
No potential conflict of interest was reported by the authors.
ORCID
Xingfeng Shao http://orcid.org/0000-0002-0724-8186
Additional information
Funding
References
- Bate NJ, Niu XP, Wang YW, Reimann KS, Helentjaris TG. 2004. An invertase inhibitor from maize localizes to the embryo surrounding region during early kernel development. Plant Physiology. 134:246–254. doi: 10.1104/pp.103.027466
- Brummell DA, Chen RK, Harris JC, Zhang HB, Hamiaux C, Kralicek AV, Mckenzie MJ. 2011. Induction of vacuolar invertase inhibitor mRNA in potato tubers contributes to cold-induced sweetening resistance and includes spliced hybrid mRNA variants. Journal of Experimental Botany. 62:3519–3534. doi: 10.1093/jxb/err043
- Camardella L, Carratore V, Ciardiello MA, Servillo L, Balestrieri C, Giovane A. 2010. Kiwi protein inhibitor of pectin methylesterase amino-acid sequence and structural importance of two disulfide bridges. The FEBS Journal. 267:4561–4565.
- Cheng SH, Liu J, Song BT, Xie CH. 2004. Cloning of potato invertase inhibitor St-inh cDNA and its expression in E. coli and functional analysis. Acta Biologiae Experimentalis Sinica. 37:269–275.
- Colaric M, Veberic R, Stampar F, Hudina M. 2005. Evaluation of peach and nectarine fruit quality and correlations between sensory and chemical attributes. Journal of the Science of Food and Agriculture. 85:2611–2616. doi: 10.1002/jsfa.2316
- Colaric M, Veberic R, Stampar F, Hudina M. 2005. Evaluation of peach and nectarine fruit quality and correlations between sensory and chemical attributes. Journal of the Science of Food and Agriculture. 85:2611–2616. doi: 10.1002/jsfa.2316
- Dickinson CD, Altabella T, Chrispeels MJ. 1991. Slow-growth phenotype of transgenic tomato expressing apoplastic invertase. Plant Physiology. 95:420–425. doi: 10.1104/pp.95.2.420
- Felsenstein J. 1985. Confidence limits on phylogenies: an approach using the bootstrap. Evolution. 39:783–791. doi: 10.1111/j.1558-5646.1985.tb00420.x
- Fridman E, Carrari F, Liu YS, Fernie AR, Zamir D. 2004. Zooming in on a quantitative trait for tomato yield using interspecific introgressions. Science. 305:1786–1789. doi: 10.1126/science.1101666
- Glaczinski H, Heibges A, Salamini F, Gebhardt C. 2002. Members of the Kunitz-type protease inhibitor gene family of potato inhibit soluble tuber invertase in vitro. Potato Research. 45:163–176. doi: 10.1007/BF02736112
- Greiner S, Köster U, Lauer K, Rosenkranz H, Vogel R, Rausch T. 2000. Plant invertase inhibitors: expression in cell culture and during plant development. Functional Plant Biology. 27:807–814. doi: 10.1071/PP99171
- Greiner S, Krausgrill S, Rausch T. 1998. Cloning of a tobacco apoplasmic invertase inhibitor proof of function of the recombinant protein and expression analysis during plant development. Plant Physiology. 116:733–742. doi: 10.1104/pp.116.2.733
- Greiner S, Rausch T, Sonnewald U, Herbers K. 1999. Ectopic expression of a tobacco invertase inhibitor homolog prevents cold-induced sweetening of potato tubers. Nature Biotechnology. 17:708–711. doi: 10.1038/10924
- He XX, Wei YY, Kou JY, Xu F, Chen ZH, Shao XF. 2018. PpVIN2, an acid invertase gene family member, is sensitive to chilling temperature and affects sucrose metabolism in postharvest peach fruit. Plant Growth Regulation. 86:169–180. doi: 10.1007/s10725-018-0419-z
- Hothorn M, D'Angelo I, Márquez JA, Greiner S, Scheffzek K. 2004. The invertase inhibitor Nt-CIF from tobacco: a highly thermostable four-helix bundle with an unusual N-terminal extension. Journal of Molecular Biology. 335:987–995. doi: 10.1016/j.jmb.2003.10.066
- Hothorn M, Ende WV, Lammensc W, Rybin V, Scheffzek K. 2010. Structural insights into the pH-controlled targeting of plant cell-wall invertase by a specific inhibitor protein. Proceedings of the National Academy of Sciences of the United States of America. 107:17427–17432. doi: 10.1073/pnas.1004481107
- Huang LF, Bocock PN, Davis JM, Koch KE. 2007. Regulation of invertase: a ‘suite’ of transcriptional and post-transcriptional mechanisms. Functional Plant Biology. 34:499–507. doi: 10.1071/FP06227
- Itai A, Hatanaka R, Irie H, Murayama H. 2015. Effects of storage temperature on fruit quality and expression of sucrose phosphate synthase and acid invertase genes in Japanese pear. Horticulture Journal. 84:227–232. doi: 10.2503/hortj.MI-047
- Jaynes TA, Nelson OE. 1971. An invertase inactivator in maize endosperm and factors affecting inactivation. Plant Physiology. 5:629–634. doi: 10.1104/pp.47.5.629
- Jutta E, Ina ST, Hardy SN, Sophia S, Engelbert W, Judith S. 2008. RNA interference-mediated repression of cell wall invertase impairs defense in source leaves of tobacco. Plant Physiology. 147:1288–1299. doi: 10.1104/pp.108.121418
- Kou XH, Zhao YN, Wu CE, Jiang BL, Zhang Z, Rathbun JR, He YL, Xue ZH. 2018. SNAC4, and, SNAC9, transcription factors show contrasting effects on tomato carotenoids biosynthesis and softening. Postharvest Biology and Technology. 144:9–19. doi: 10.1016/j.postharvbio.2018.05.008
- Kursanov AL, Dubinina IM, Burakhanova EA. 1971. A natural inhibitor of invertase from sugar beet roots. Soviet Plant Physiology. 18:480–484.
- Lin Y, Liu J, Liu X, Ou YB, Li M, Zhang HL, Song BT, Xie CH. 2013. Interaction proteins of invertase and invertase inhibitor in cold-stored potato tubers suggested a protein complex underlying post-translational regulation of invertase. Plant Physiology and Biochemistry. 73:237–244. doi: 10.1016/j.plaphy.2013.09.012
- Link M, Rausch T, Greiner S. 2004. In Arabidopsis thaliana, the invertase inhibitors AtC/VIF1 and 2 exhibit distinct target enzyme specificities and expression profiles. FEBS Letters. 573:105–109. doi: 10.1016/j.febslet.2004.07.062
- Liu X, Song BT, Zhang HL, Li XQ, Xie CH, Liu J. 2010. Cloning and molecular characterization of putative invertase inhibitor genes and their possible contributions to cold-induced sweetening of potato tubers. Molecular Genetics and Genomics. 284:147–159. doi: 10.1007/s00438-010-0554-3
- Liu X, Zhang C, Ou YB, Lin Y, Song BT, Xie CH, Liu J, Li XQ. 2011. Systematic analysis of potato acid invertase genes reveals that a cold-responsive member, StvacINV1, regulates cold-induced sweetening of tubers. Molecular Genetics and Genomics. 286:109–118. doi: 10.1007/s00438-011-0632-1
- Livak KJ, Schmittgen TD. 2001. Analysis of relative gene expression data using real-time quantitative PCR and the 2 −ΔΔ C T method. Methods-A Companion To Methods in Enzymology. 25:402–408. doi: 10.1006/meth.2001.1262
- Mckenzie MJ, Chen RK, Harris JC, Ashworth MJ, Brummell DA. 2013. Post-translational regulation of acid invertase activity by vacuolar invertase inhibitor affects resistance to cold-induced sweetening of potato tubers. Plant Cell and Environment. 36:176–185. doi: 10.1111/j.1365-3040.2012.02565.x
- Mckenzie MJ, Sowokinos JR, Shea IM, Gupta SK, Lindlauf RR, Anderson JAD. 2005. Investigations on the role of acid invertase and UDP-glucose pyrophosphorylase in potato clones with varying resistance to cold-induced sweetening. American Journal of Potato Research. 82:231–239. doi: 10.1007/BF02853589
- Miao XR, Niu JQ, Mo ZZ, Wang AQ, He LF. 2018. Cloning and expression analysis of invertase inhibitor gene families from Dendrobium officinale. Biotechnology Bulletin. 34:129–136.
- Paynel F, Leroux C, Surcouf O, Schaumann A, Pellou J, Driouich A, Mollet JC, Lerouge P, Lehner A, Mareck A. 2014. Kiwi fruit PMEI inhibits PME activity, modulates root elongation and induces pollen tube burst in Arabidopsis thaliana. Plant Growth Regulation. 74:285–297. doi: 10.1007/s10725-014-9919-7
- Qin GZ, Zhu Z, Wang WH, Cai JH, Chen Y, Li L, Tian SP. 2016. A tomato vacuolar invertase inhibitor mediates sucrose metabolism and influences fruit ripening. Plant Physiology. 172:1596–1611. doi: 10.1104/pp.16.01269
- Rausch T, Greiner S. 2004. Plant protein inhibitors of invertases. Biochimica et Biophysica Acta. 1696:253–261. doi: 10.1016/j.bbapap.2003.09.017
- Roitsch T, Balibrea ME, Hofmann M, Proels R, Sinha AK. 2003. Extracellular invertase: key metabolic enzyme and PR protein. Journal of Experimental Botany. 54:513–524. doi: 10.1093/jxb/erg050
- Schaffer AA, Rylski I, Fogelman M. 1989. Carbohydrate content and sucrose metabolism in developing Solanum muricatum fruits. Phytochemistry. 28:737–739. doi: 10.1016/0031-9422(89)80105-0
- Schwimmer S, Makower RU, Rorem ES. 1961. Invertase & invertase inhibitor in potato. Plant Physiology. 36:313–316. doi: 10.1104/pp.36.3.313
- Shivalingamurthy SG, Anangi R, Kalaipandian S, Glassop D, King GF, Rae AL. 2018. Identification and functional characterization of sugarcane invertase inhibitor (ShINH1): a potential candidate for reducing pre- and post-harvest loss of sucrose in sugarcane. Frontiers in Plant Science. 9:1–14. doi: 10.3389/fpls.2018.00598
- Stitt M, Schaewen AV, Willmitzer L. 1991. Sink regulation of photosynthetic metabolism in transgenic tobacco plants expressing yeast invertase in their cell wall involves a decrease of the Calvin-cycle enzymes and an increase of glycolytic enzymes. Planta. 183:40–50. doi: 10.1007/BF00197565
- Tang XF, Su T, Han M, Wei L, Wang WW, Yu ZY, Xue YG, Wei HB, Du YJ, Greiner S, et al. 2016. Suppression of extracellular invertase inhibitor gene expression improves seed weight in soybean (Glycine max). Journal of Experimental Botany. 68:469–482.
- Tauzin AS, Sulzenbacher G, Lafond M, Desseaux V, Reca IB, Perrier J, Bellincampi D, Fourquet P, Lévêque C, Giardina T. 2014. Functional characterization of a vacuolar invertase from Solanum lycopersicum: post-translational regulation by N-glycosylation and aproteinaceous inhibitor. Biochimie. 101:39–49. doi: 10.1016/j.biochi.2013.12.013
- Valero D, Serrano M, Martínez-Madrid MC, Riquelme F. 1997. Polyamines, ethylene, and physicochemical changes in low-temperature-stored peach (Prunus persica L. cv. Maycrest). Journal of Agricultural and Food Chemistry. 45:3406–3410. doi: 10.1021/jf970182p
- Wang WH, Cai JH, Wang PW, Tian SP, Qin GZ. 2017. Post-transcriptional regulation of fruit ripening and disease resistance in tomato by the vacuolar protease SlVPE3. Genome Biology. 18:1–23. doi: 10.1186/s13059-016-1139-1
- Wang K, Shao XF, Gong YF, Zhu Y, Wang HF, Zhang XL, Yu DD, Yu F, Qiu ZY, Lu H. 2013. The metabolism of soluble carbohydrates related to chilling injury in peach fruit exposed to cold stress. Postharvest Biology and Technology. 86:53–61. doi: 10.1016/j.postharvbio.2013.06.020
- Weber H, Borisjuk L, Wobus U. 1996. Controlling seed development and seed size in Vicia faba: a role for seed coat-associated invertases and carbohydrate state. The Plant Journal. 10:823–834. doi: 10.1046/j.1365-313X.1996.10050823.x
- Weil M, Krausgrill S, Schuster A, Rausch T. 1994. A 17-kDa Nicotiana tabacum cell-wall peptide acts as an in-vitro inhibitor of the cell-wall isoform of acid invertase. Planta. 193:438–445.
- Wu L, Bhaskar PB, Busse JS, Zhang RF, Bethke PC, Jiang JM. 2011. Developing cold-chipping potato varieties by silencing the vacuolar invertase gene. Crop Science. 51:981–990. doi: 10.2135/cropsci2010.08.0473
- Xu XX, Hu Q, Yang WN, Jin Y. 2017. The roles of cell wall invertase inhibitor in regulating chilling tolerance in tomato. BMC Plant Biology. 17:1–13. doi: 10.1186/s12870-016-0951-9
- Ye J, Shakya R, Shrestha P, Rommens CM. 2010. Tuber-specific silencing of the acid invertase gene substantially lowers the acrylamide-forming potential of potato. Journal of Agricultural and Food Chemistry. 58:12162–12167. doi: 10.1021/jf1032262
- Yu LN, Liu HX, Shao XF, Yu F, Wei YZ, Ni ZM, Xu F, Wang HF. 2016. Effects of hot air and methyl jasmonate treatment on the metabolism of soluble sugars in peach fruit during cold storage. Postharvest Biology and Technology. 113:8–16. doi: 10.1016/j.postharvbio.2015.10.013
- Yu F, Ni ZM, Shao XF, Yu LN, Liu HX, Xu F, Wang HF. 2015. Differences in sucrose metabolism in peach fruit stored at chilling stress versus nonchilling stress temperatures. Hortscience. 50:1542–1548. doi: 10.21273/HORTSCI.50.10.1542
- Yun HS, Yoon IS, Kang BG. 2002. Rapid repression of vacuolar invertase in mungbean hypocotyl segments and regulation by sucrose, auxin and light. Plant Growth Regulation. 38:181–189. doi: 10.1023/A:1021251618606
- Zhang N, Jiang J, Yang YL, Wang ZH. 2015. Functional characterization of an invertase inhibitor gene involved in sucrose metabolism in tomato fruit. Journal of Zhejiang University-Science B. 16:845–856. doi: 10.1631/jzus.B1400319