Abstract
The small satellite renaissance began in the 1980s and is changing the economics of space. Technological trends have supported the advancement of small satellites in the 1–500 kg range. The number of countries actively participating has grown substantially during the past years. Satellite constellations (groups of satellites working in concert) are emerging as a powerful and effective application. In this paper, we focus on the small satellites than can perform remote sensing or Earth observation tasks. An overview is presented of the small satellite literature on Earth observation. The aim of the survey is threefold: an introduction for those new to the field, an overview for those working in the field and a reference for those searching for literature on a specific application for Earth observation. Small satellite programmes are classified according to the geographic regions. The small satellite industry and small satellite systems are addressed. In terms of applications, small satellite constellations are discussed in more detail. Finally, future developments are put forward. Telegeoprocessing combined with grid computing will provide the infrastructure and technologies for the development of Processing on Demand for Small Satellite Constellation systems.
1. History
In the late 1980s a new satellite paradigm, modern small satellites, arose and opened up a new class of space applications. Small satellites may be an enabling, even disruptive technology, but it is the mission they perform that matters in all cases. Whatever the application, the utility of a small space mission is the metric by which small satellites will ultimately be measured, and thereby gain further acceptance.
The classification of satellites according to mass is usually as follows (Konecny Citation2004, www.isprs.org/istanbul2004/comm4/papers/428.pdf):
large satellites: mass > 1000 kg; | |||||
medium satellites: mass 500–1000 kg; | |||||
mini‐satellites: mass 100–500 kg; | |||||
micro‐satellites: mass 10–100 kg; | |||||
nano‐satellites: mass 1–10 kg; | |||||
pico‐satellites: mass 0.1–1 kg; | |||||
femto‐satellites: mass <100 g. |
A very slightly different version of the classification is found in table of the companion article by Kramer and Cracknell (Citation2008) in this issue; and this involves extending the range of mini‐satellites from 100 to 1000 kg and abolishing the medium satellite class.
Micro‐ and nano‐satellites are important tools for exploring and testing new ideas and various new devices for space missions without spending huge amounts of money. The actual launch cost per kilogram payload on a micro‐ or nano‐satellite can be as high or even higher than ordinary satellites but the turnaround time and quick responses are extremely important (Becanovic et al. 1999). Nano‐satellites are attractive to many educational institutions to get involved in space, as commonly available technology now makes this type of satellite feasible and, most importantly, affordable. Pico‐satellites weighing less than 1 kg are still some time off for commercial applications. Boston University has received a NASA grant to study a pico‐satellite constellation. The PalmSAT programme is a pico‐satellite programme at the Surrey Space Centre at the University of Surrey, UK. Recently a renewed interest has developed for employing micro‐satellites in the scientific, commercial and military market, as technology has permitted sophisticated payloads to be accommodated in smaller volumes.
The first micro‐satellites were launched during the period 1957–1969. In the period 1980–1999 a total of 238 mini‐satellites and 249 micro‐satellites were launched from countries all over the globe including India, Germany, Japan, Korea, Saudi Arabia, China, Algeria, and Malaysia. A special issue on small satellite engineering was published in 1999 (Swinerd Citation1999). The International Academy of Astronautics (IAA) has conducted six symposia on small satellites organized by the DLR Berlin‐Adlershof in Berlin, Germany (1997, 1999, 2001, 2003, 2005, 2007) (http://www.dlr.de/iaa.symp/desktopdefault.aspx/tabid‐3606/). An ‘International Study on Cost‐Effective Earth Observation Missions’ edited by Dr Rainer Sandau (Citation2006) for IAA Commission IV Study Group: System Operation and Utilization, as stated, is the first of its type that attempted to detail the issues in achieving cost‐effective Earth Observation (EO) missions. It was compiled by an impressive set of experts with many years of experience in space development programmes. Its emphasis is on small satellite missions, which are being undertaken for EO programmes by both developed and developing countries. The 21st Annual AIAA/USU Conference on Small Satellites (http://www.smallsat.org/) focused on the mission and the underlying mission‐enabling technologies that make them uniquely capable platforms, either individually or in constellations. An International Workshop on Earth Observation Small Satellites for Remote Sensing Applications was held in 13–16 November 2007, in Kuala Lumpur, Malaysia (http://www.bilten.metu.edu.tr/ISPRS_WG_I_6/kuala_lumpur.html). Kramer and Cracknell (Citation2008) have produced an overview of small satellites in remote sensing based on Dr Kramer's presentation at that Workshop (Kramer Citation2007).
The web site, http://centaur.sstl.co.uk/SSHP/sshp_intro.html, lists the types of small satellite customers (e.g. commercial, military, university, etc.) in 1980–1999 as shown in figure and the application of these satellites in that period is shown in figure . For this paper, of special interest are those small satellites that were used for remote sensing. A selection of these is listed in table .
Figure 1 The uses of small satellite(i.e. customer types) in 1980–1999 (adapted from http://centaur.sstl.co.uk/SSHP/sshp_intro.html).
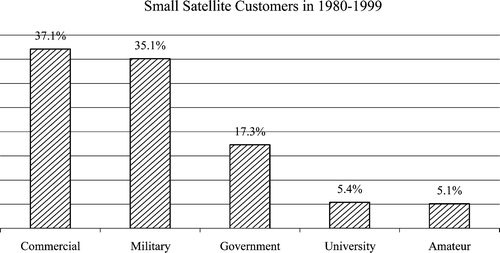
Figure 2 The application of small satellites in 1980–1999 (adapted from http://centaur.sstl.co.uk/SSHP/sshp_intro.html).
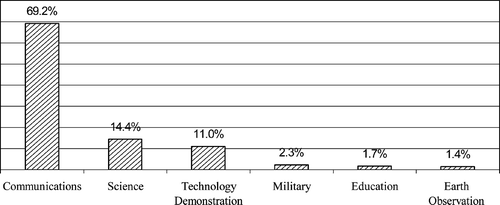
Table 1. Small remote sensing satellites.
1.1 America
In recent years, ‘faster, better, cheaper’ has become the National Aeronautics and Space Administration's (NASA's) motto for future missions. The aim of NASA's Earth Science Enterprise (ESE) is to develop a scientific understanding of the Earth system and its response to natural and human‐induced changes to enable improved prediction of climate, weather, and natural hazards for present and future generations (Neeck et al. Citation2005). The ESE has an end‐to‐end strategy to ensure that information, understanding and capabilities derived from its research programme achieve maximum usefulness to the scientific and decision‐making communities. Small satellites (<500 kg) have been crucial contributors to satisfying the research strategy since the inception of NASA's Earth Observation programme in the 1960s. In the last decade, NASA's ESE has placed a renewed emphasis on small satellites. This reemphasis reflects advances in compact sensor, small satellite bus, and launch vehicle technologies in addition to management innovations. Near term and advanced planning suggest that this trend will continue. A number of related small satellite missions have been recently launched, are in development, or are planned. SAC‐C was launched into a 705 km Sun‐synchronous orbit as a dual payload with the NASA EO‐1 mission in November 2000. The principal mission of the Argentine Satellite De Aplicaciones Cienificas (SAC‐C) is to utilize a five‐band camera for Earth observation (Ousley Citation2003). Multi‐satellite constellations under study include small satellites as key architectural elements. Studies indicate that low cost, capable microspacecraft along with compact sensors and increased autonomy are technology enablers to the sensorwebs and associated distributed spacecraft infrastructure required to realize the long‐term NASA Earth Science Vision (ESV).
1.2 Europe
Small satellites are becoming more important in the ESA programme because, by investing in small satellite technology, ESA expects to apply the results and experience gained to larger missions with a view to reducing the cost. Also, small satellite design and project management is good training for the engineers and managers who will take responsibility for larger projects in the future. ESA has launched one small satellite, which was built in a very short timescale by young graduate students and ESA staff (Creasey and Whitcomb Citation1999). Two others are in preparation, namely the PROBA (Project for On‐Board Autonomy) mission (http://www.esa.int/SPECIALS/Proba_web_site/index.html), which addresses technology developments in general for future missions, and the SMART‐1 mission, which is specifically aimed at proving the use of electric propulsion. ESA's PROBA spacecraft is one of the most advanced small satellites ever flown in space. Launched in 2001, as a technology demonstration mission, it is now operating as an Earth Observation Third Party Mission. SMART stands for Small Mission for Advanced Research and Technology. SMART‐1 was the first successful European spacecraft to the Moon (http://www.esa.int/SPECIALS/SMART‐1/index.html). Gampe et al. (Citation1995) described the results of an ESA study on two possible Earth observation missions intended to provide improved services based on advanced technologies for satellite bus and payload. The application areas were: (1) a (near) real‐time meteorological service for commercial airlines, and (2) a surface water management service for arid/semi‐arid regions of the world. They presented conceptual designs and showed the impact of advanced technologies compared to conventional ones. Advanced programmatics such as lean AIV (assembly, integration and verification) and management structures add to the picture of a smart approach. With a view to commercialization, all design concepts should be concentrated on one goal: ‘Sell a service not just a spacecraft!’.
D'Errico et al. (Citation1996) presented a new scientific space mission consisting of a satellite carrying a receiving‐only Synthetic Aperture Radar (SAR) which receives the signal transmitted by the ENVISAT‐1 SAR. Moccia et al. (Citation2000) conducted a feasibility study of a new space‐based observation technique, bistatic synthetic aperture radar, which involves an active microwave sensor operating with separated transmitting and receiving antennae. The integration of ENVISAT‐1 SAR and bistatic radar data offers an improved potential for surface classification, three‐dimensional observation, and the opportunity of advanced scientific experiments in the field of bistatic scattering. In addition to conventional ASAR images, echoes gathered by Bistatic and Interferometric SAR Satellite (BISSAT) with bistatic synthetic aperture radar form an additional dataset. They offer an added value to ENVISAT scientific exploitation, as they could be used for novel mapping applications, taking into account their unique pointing characteristics and differences in terrain‐scattering properties as a function of antennae separation. The proposed mission (BISSAT) concept consists of flying a passive SAR on board a small satellite, which observes the area illuminated by an active SAR, operating on an already existing large platform, e.g. ENVISAT. Several scientific applications of bistatic measurements can be envisaged, such as: evaluation of bistatic radar cross‐section; improvement of image classification and pattern recognition procedures; acquisition of terrain elevation and slope, acquisition of velocity measurements; interferometric surveys upon completion of the nominal bistatic mission and successful baseline control; and stereogrammetric applications. The small satellite nominal orbit and the attitude manoeuvres are designed in order to maintain an adequate overlap between the two radar swaths along the whole orbit, taking into account the ENVISAT‐1 attitude and pointing.
Liebig (2000) summarized the German small satellite programme and described all actual German small satellite Earth observation missions. A concrete example of the uses of small satellites is that of BIRD (Bispectral Infra‐Red Detection), a small satellite launched in October 2001, and developed and built by DLR (Briess et al. 2000). The BIRD mission is dedicated to hot spot detection and investigation from space by means of newly developed infrared array sensors. A dedicated two‐channel cooled infrared sensor system was developed for hot spot detection and investigation from space. It is combined with a two‐channel VIS/NIR sensor system for remote sensing of vegetation. They are suitable for Earth remote sensing with small satellites. This small satellite demonstrated for the first time that the full extent of forest fires and the resulting flame temperatures could be detected from orbit. The scientific objective of this project also embraces the accurate observation of volcano activity as well as cloud and vegetation analyses.
The German Space Operations Center (GSOC) has the main responsibility for BIRD operations, while data reception was carried out by the receiving station in Neustrelitz. An experimental ground station (EGS) for the Earth observing small satellite project BIRD of the German Aerospace Center (DLR) was developed. The objective of the EGS is to investigate and demonstrate direct user reception of regional data from Earth observing satellites with a low‐cost ground station as well as to analyse autonomous activity capabilities and experimental tests for mission operations (Kayal 2000). For this purpose, a small ground station with data reception and commanding capabilities was built. The station is located in the Institute of Aeronautics and Astronautics (ILR) of the TUB (Technical University of Berlin), Berlin, Germany, on the top of the institute building.
The success of the Swedish small satellite programme, in combination with an active participation by Swedish research groups in major international missions, has placed Sweden in the frontline of experimental space research (Marklund et al. Citation2004). The programme started with the development of the research satellite Viking, which was launched in 1986, for detailed investigations of the aurora. To date, Sweden has developed and launched a total of six research satellites – five mini‐ and micro‐satellites for space plasma investigations and the most recent mini‐satellite Odin, for research in astronomy and aeronomy. The five space plasma missions have now ended and a comprehensive scientific data analysis has been conducted, which allows for a judgement of their role and impact on progress in auroral research. The two mini‐satellites Viking and Freja, the most well‐known missions of this programme, were pioneers in the exploration of the aurora. The more recent micro‐satellites, Munin, Astrid and Astrid‐2, proved to be powerful tools, both for testing new technologies and for carrying out advanced science missions. The Swedish small satellite programme has been internationally recognized as cost efficient and scientifically very successful.
ASI, the Italian Space Agency, is going to finance the development of a small satellite platforms production line called PRIMA (Piattaforma Riconfigurabile Italiana Multi‐Applicativa) with Alenia Aerospazio partnership as Prime Contractor and sub‐contractors preferably chosen within the Italian Space Industries. The main objective of the programme is to develop a multi‐mission, easily adaptable platform for low Earth orbits, which allows rapid, efficient and low cost bus for application and scientific missions (Galeazzi Citation2000). The Italian national space plan foresees the build up of the Skymed/Cosmo constellation as application Earth observation programme plus a number of small scientific spacecraft with a rate of two missions every three years. To fulfil these schedules, the PRIMA platform should complete its development and qualification programme in order to be ready for the first unit (proto‐qualification flight) to interface the first payload (science or application).
Urech et al. (Citation2000) described the CESAR Mission, an Earth Observation Satellite Mission developed by cooperation between INTA (Instituto Nacional de Tecnica Aeroespacial) from Spain and CONAE (Comision Nacional de Actividades Espaciales) from Argentina. The Mission consists of the design, construction, launch and operation of a small satellite, less than 500 kg, and the update of the existing ground segment capabilities in Spain and Argentina to receive and process the generated data. The primary objectives will be to examine cartography, natural resources and geophysics, with a satellite payload composed of a panchromatic camera with 5 m geometric resolution and 10 bits radiometric resolution, for cartography and topography studies; and a multispectral camera with six bands, 34 m geometric resolution and 10 bits radiometric resolution, for thematic studies; and high sensibility panchromatic camera with 1 km geometric resolution and a spectrometer, for geophysics studies. The proposed CESAR satellite orbital parameters were: perigee 400 km, apogee 1000 km, inclination 70°, period 98.8 min.
France and Denmark also developed their own small satellite programmes (Tastemain Citation1995, Thomsen and Hansen Citation2000).
1.3 Asia and Africa
A number of developing countries are undertaking projects pertaining to the design and development of small satellites, either using their own resources or in collaboration with foreign countries on a regional or international basis. Vibulsresth et al. (Citation1997) described the Thai Remote Sensing Small Satellite (TRSSS) Programme and its overall system concept. The mission requirements, design philosophy, preparation, programme management, human resource development, technology transfer, applications development, users' networking and operational scenario for the planned 5‐year lifespan of the satellite were also described. The programme's main aim was to provide more effective tools for management of natural resources and the environment of the kingdom of Thailand, which is one of the world's major agricultural produce exporting nations. The programme was also a part of Thailand's input for international cooperation with special focus for providing services to neighbouring ASEAN (Association of South East Asian Nations) countries. TRSSS's system architecture consists of four main components, namely: Ground Segment; Mission Control Centre (MCC) and Data Acquisition and Processing Facility (DAPF): Space Segment, comprising a spacecraft bus and an instrument; Launch Segment; and Applications Segment.
Mahanakorn University of Technology (MUT), in Thailand, entered into a technology transfer programme to design and test a microsatellite with the University of Surrey, in the UK. A team of 12 staff members from MUT joined in designing and building Thailand's first microsatellite, named Thai‐Paht, over a 1‐year period (Jantarang Citation2000). Thai‐Paht was launched into a Sun‐synchronous low Earth orbit on a Zenith‐Il rocket from Baikonur cosmodrome in Kazakhstan on 10 July 1998. The average height of the orbit is 815 km. Thai‐Paht weighs 50 kg and has imaging and digital communication payloads. Three narrow‐angle optical cameras take pictures, using a snapshot technique, in three spectral bands with 65 m resolution. The picture size is 1024×1024 pixels. A wide‐angle camera photographs an area of 1156 km×1152 km. The ground station is installed at MUT to control and communicate with Thai‐Paht two or three times each day during two time periods, 8.30–12.30 h and 20.30–00.30 h. Thai‐Paht will provide immense benefits in education and in allowing Earth observation at very low cost.
In Turkey the Information Technologies and Electronics Research Institute (BILTEN) has initiated a low‐Earth orbit small satellite project. This project is part of a programme to achieve expertise in satellite‐related technologies. The main goal of the project is to transfer technology on building small satellites. Details of BILTEN and the programme are given in Askar and Tekinalp (Citation2000).
Pakistan is also actively involved in the development of the Small Multi‐Mission Satellite (SMMS) project of the Asia Pacific Multilateral Cooperation in Space Technology and Applications (AP‐MCSTA). In this project, Pakistan's Space and Upper Atmosphere Research Commission (SUPARCO) is collaborating with counterpart space agencies in China, Thailand, the Republic of Korea and other nearby countries. The main aims of these efforts were to give a boost to the country's R&D efforts in space technology and develop human resources in this field through hands‐on experience in the building and operation of satellites, and acquisition of new skills in project definition, funding and implementation.
Alex et al. (Citation1983) addressed the remote sensing payload for a small Indian satellite. The Polar Satellite Launch Vehicle (PSLV) developed by the Indian Space Research Organization (ISRO) has entered its operational phase, the successful launches being the PSLV‐Cl/IRS‐1D mission carried out in September 1997. In this flight, the enhanced payload capability of the vehicle, namely 1200 kg in 800 km SSPO (Sun‐Synchronous Polar Orbit), had been validated and this uprated configuration served as the standard workhorse to orbit operational Indian Remote Sensing (IRS) satellites for the country's Earth Observation Programme (Ramakrishnan et al. Citation1999). The second operational flight of PSLV (PSLV‐C2) carries the auxiliary satellites KITSAT‐3 developed by Satellite Technology Research Centre (SaTReC) of South Korea and DLR‐TUBSAT developed by DLR/Technical University of Berlin. The primary satellite is the Indian Remote Sensing Satellite, IRS‐P4.
Ono et al. (Citation1998) introduced the Hypersat and Mission Demonstration Satellite programmes, which were small satellite programmes in the National Space Development Agency of Japan (NASDA). The goals of the programmes were to establish sophisticated microelectronics and mechanics technology; to achieve low cost, high performance satellite; and to respond quickly to the variety of requests from end users. A wide swath strip mode operation SAR design was also introduced.
TiungSat‐1 is Malaysia's first micro‐satellite and was developed as a collaborative effort between the Malaysian space agency, ANGKASA, and Surrey Satellite Technology Ltd.
The efforts involved in developing a small satellite for scientific purposes in China in the 1990s have been described by Hu and Du (Citation1994) and in Aviation Week & Space Technology (Anonymous Citation1996). The project was arranged on a case‐to‐case principle depending upon requirements and financial support. The space technology of satellites and rockets, which has been developed over a relatively long period in China, has been transferred to the scientific research of small satellites for improvement of the quality requirements. The surplus payloads of the rocket and satellite are used as the payloads of the small satellite and scientific experiments at a low cost.
Surrey Satellite Technology Limited (SSTL) developed the NigeriaSat‐1 enhanced microsatellite during a know‐how and technology transfer programme for the Federal Ministry of Science and Technology (FMST) of Nigeria. NigeriaSat‐1 is a satellite of the standard Disaster Monitoring Constellation (DMC) design. It carries an optical imaging payload developed by SSTL to provide 32 m ground resolution with an exceptionally wide swath width of over 640 km. Nigeria formed a National Space Research and Development Agency (NASRDA), which now manages the NigeriaSat‐1 programme. NigeriaSat‐1 was launched in September 2003 from Pletsesk on a Kosmos launch vehicle.
2. Small satellite industry
Space‐based activities are extremely demanding and are unforgiving of shortfalls in technology, organization and management – especially once in orbit. To achieve success, a commercial company must therefore tackle each of the aforementioned categories. At the lower cost end of the market, mission objectives are generally well defined, thereby aiding the design to cost and schedule, important in times of fast technological advancement and the need for immediate results. However, to crown this with a successful mission, a novel satellite engineering philosophy is required – particularly for faster, cheaper, better and smaller satellites (Smithies et al. Citation2003).
Dobrozrakov et al. (Citation1996) considered the demonstrative small satellite projects (DSSP) for appreciation of the small satellites' effective use in the perspective of national, space systems of remote sensing environment, as well as the ozone layer and the near Earth space. The peculiarities of the Russian market of remote sensing information products and services were analysed to specify and stress the significance and perspectives of this market. In view of budgetary constraints in the transition period from centralized to market economics in Russia, the different sources of DSSP financial support are considered. They also discussed the national potential for the development, building, launch and missions of small satellites.
Dale et al. (Citation1994) reviewed the background to the joint ESA/industry dialogue that has been taking place in recent years with a view to further improving the contractual and managerial interfaces within the ESA Scientific Programme. It described the main events in this continuing dialogue and summarized industry's and the Agency's perspectives on the major topics addressed in the various working groups. Particular emphasis was placed on the outcome of the joint Workshop held in October 1993, which was a concluding milestone of the initial phase in the ESA/industry exchange.
Table 2. Small satellite constellations.
The RapidEye (http://www.rapideye.de/) business concept was initiated in 1996 by Kayser‐Threde GmbH, a German space engineering company, with support from the German Space Agency (DLR). The concept is to provide end‐to‐end solutions to clients whose geospatial information needs require large‐area coverage, repetitive monitoring and frequent revisits with an integrated system that includes a constellation of five Earth observation satellites and a ground processing segment capable of delivering custom geospatial solutions. RapidEye was established as an independent company in December 1998 once the concept had matured sufficiently to receive seed financing from the German Space Agency (DLR) and Vereinigte Hagelversicherung (VH), the largest German agro‐insurance company, as well as a few private investors. Over nearly a decade of development, the RapidEye technical and business plan has retained its original primary objective of providing end‐to‐end solutions to clients whose geospatial information needs require large‐area coverage, repetitive monitoring and frequent revisits. With this overall objective in mind, RapidEye has designed an integrated system that includes a constellation of five Earth observation satellites and a ground processing segment capable of delivering custom geospatial solutions.
Alenia Aerospazio has created a new centre for the Assembly, Integration and Testing (AIT) of small satellites to meet these new demands for a constellation of tens (in some cases hundreds) of small satellites. Located in Rome near Alenia Aerospazio production sites for easy access to consolidated expertise in specific related disciplines, the approach selected for the new Alenia Aerospazio plant was based on the use of ‘islands’ and working areas where a series of homogeneous activities is performed at the end of which the rate and quality of output can be checked (Simeone and Pignatelli Citation1997). An advanced data management system allows the real‐time collection and analysis of test results. The chosen method is flexible enough to allow the integration of small satellites for different types of missions. The Small Satellite Centre is currently occupied with the production of the satellites of the Globalstar programme. This programme foresees a constellation of 48 satellites in low orbit, plus eight as backup, placed in groups of six on eight different orbital planes.
Carayannis and Roy (Citation1998) studied the nature, structure and dynamics of technological innovation and in particular, its speed and acceleration, in the small satellite manufacturing sector in both the US and international markets. A conceptual framework of the nature, structure and dynamics of technological innovation was developed (Carayannis and Roy Citation2000), which applied to the small satellite industry. Important components of this framework include: (a) the speed and acceleration of technological innovation and (b) the linear and non‐linear interactions between technology producers and users (technology and market push and pull mechanisms). They conceptualized technology innovation and commercialization as an ongoing cooperative and competitive process among enabling and inhibiting factors or mechanisms. Enabling factors include Cooperative Research And Development Agreements (CRADAs), strategic alliances, spin‐offs, intellectual property rights, Small Business Innovation Research (SBIR), and mentor–protégé relationships. Inhibiting factors include excessive regulation at state, national and international levels, technological, structural or financial barriers to market entry, competitor response to market entry and culture clashes such as engineering versus marketing culture or company versus government versus university cultures. Two of the companies studied using the above framework were US start‐ups and one is a British university spin‐off.
Surrey Satellite Technology Limited (SSTL) (http://www.sstl.co.uk/) is a world leader in the design, manufacture and operation of high performance small satellites. The company was formed in 1985 by the University of Surrey to commercialize the results of its innovative small satellite engineering research. SSTL was the first professional organization to offer low‐cost small satellites within rapid timescales employing advanced terrestrial technologies. In 25 years SSTL has built a profitable business around a unique approach to space (Morring Citation2001). Liddle et al. (Citation2004) gave a description of the development at SSTL in the UK of a satellite platform for use in a Geostationary Earth Orbit. Commercial Space Technologies, Ltd (CST) (http://www.commercialspace.co.uk/) has undertaken several studies of the methods available for the launch of GEMINI‐1, a 400 kg test satellite being built by SSTL in collaboration with Nigeria, for the British National Space Centre (BNSC), who have included it in their MOSAIC UK small‐satellite programme. Mosaic, or Micro Satellite Applications in Collaboration, was a government project led by BNSC with one of its partners, the UK Ministry of Defence (MoD), funding half the TopSat project. Mosaic gave scientists and companies in the UK the opportunity to submit ideas for new missions that would test the effectiveness and application of small satellite capability. The three winners, announced in 2000, were Gemini, TopSat and DMC. The three teams responsible received the funding to take their ideas through to launch. The results of these studies with cost comparisons were presented in the paper by Webb et al. (Citation2006). It was shown that the cost per kilogram of placing a mini‐satellite into geostationary orbit need not be any more than that for larger satellites and in some special circumstances may be significantly cheaper. This interesting result could have far‐reaching consequences for the communication marketplace and technologies. It could also encourage research and development, innovation and independence. SSTL has the world's most extensive track record of providing small satellite missions. This section of our site provides information on our current missions, and will soon include summaries of all past missions.
In Japan, Mitsubishi plans bigger small experimental satellite (Anonymous Citation2003). Orii et al. (Citation2002) introduced the versatile small satellite system architecture developed by NEC Corporation, Japan. The versatile small satellite design features the standardization concept both in the electrical functional interface and also the physical interface and the modular configuration, which results in the reduction of not only the delivery time but also the cost of the satellite. The versatile small satellite has the satellite mass range of 400–1000 kg adaptability.
Aerospace Dongfanghong Satellite Company (DFHSat) (http://www.cast.cn/CastEn) is a modern hi‐tech enterprise invested in and established by China Aerospace Science and Technology Corporation and Chinese Academy of Space Technology (CAST) on 8 August 2001. The company is mainly engaged in the development of small satellites and serialized common platforms, the system design and the product development of satellite applications engineering. As one important department of CAST, with responsibility for small and microsatellite development, DFHSat manufactured and launched six small satellites from 2000 to 2004 (Zhang et al. Citation2007). Nowadays, DFHSat is developing micro‐satellite and new enhanced small satellite series. The first micro‐satellite as a basic type is named HummerSat‐1. HummerSat‐l is three‐axis stabilized with orbit control capability. Information and power control are implemented through an on‐board network, a GaAs solar cell and a Li‐ion battery are adopted to obtain and store power, S‐band TT&C (Telemetry, Tracking & Command) and data transmission works are used. The payload of HummerSat‐1 has a weight of 60 kg and power consumption of 200 W. The enhanced small satellite series based on the technology of HummerSat‐1 was planned by DFHSat. It would be aiming to form a sequential product spectrum of 80–200 kg. The enhanced series is focused on satisfying different missions, including Earth remote sensing, communication, science exploration, etc.
3. Small satellite systems
In the late 1980s a new satellite paradigm, modern small satellites, arose and opened up a new class of space applications. Cvetkovic and Robertson (Citation1993) discussed the idea of adapting existing small satellite technology for remote sensing purposes. The major design problems and constraints influencing the design of a small low‐cost remote sensing satellite bus are identified using the subsystem approach. Key design areas include the improvement of battery technology and the development of a deployable solar array, attitude control assemblies, on‐board data processing/storage and ground station data acquisition. Although the eventual satellite would also have to be somewhat larger, more powerful and, above all, more sophisticated than the previous small satellites, this is considered to be a natural progression of research in this area, and will form part of a more detailed future study.
Recent announcements by commercial semiconductor manufacturers to introduce Silicon On Insulator (SOI) technology into their commercial product lines is driven by the need for high‐performance low‐power integrated devices. Moreover, SOI has been the technology of choice for many space semiconductor manufacturers where radiation requirements are critical. This technology has inherent radiation latch‐up immunity built into the process, which makes it very attractive to space applications. Alkalai (Citation2000) described the advanced microelectronics and avionics technologies under development by NASA's Deep Space Systems Technology Program (also known as X2000). These technologies are of significant benefit to both the commercial satellite as well as the deep‐space and Earth orbiting science missions. Such a synergistic technology roadmap may truly enable quick turnaround, low‐cost, and highly capable small satellite systems for both Earth observation as well as deep‐space missions.
Garrido et al. (Citation2000) presented an overview of the software subsystem for Earth Observation satellites and the life cycle model to be followed. From the on‐board software subsystem point of view inside the Small Satellite Spanish Programme for Earth Observation satellites, it has been attempted to develop a general subsystem, and then particularize for each satellite. On the other hand, they proposed that a Software Life Cycle Model should mirror the industrial scheme (i.e. the companies that do the different parts of the job) for the development and test tasks. In order to apply this idea a ‘Spread‐V’ Software Life Cycle Model had been defined, which showed in just one diagram the whole embedded software subsystem. The main functions to be performed by the on‐board software of a generic Earth observation satellite were summarized. It also showed the subsystem decomposition into three different components, each one to be developed by a different company. The proposed Software Life Cycle to be followed was presented. Finally, the close relationship between the general software architecture and the Software Life Cycle Model was identified.
Over the past few years Satellites International has developed an integrated suite of satellite sub‐systems and small satellite buses. The sub‐systems include S‐band communications, attitude sensing and control, power conversion and distribution, and on‐board data handling. They are inherently modular and readily adaptable to different satellite configurations, a concept known as semi‐standardization (Ward et al. Citation1996, Boland et al. Citation1999). This concept has been adopted by two generic low‐cost buses: MicroSIL for satellites in the mass range 40–80 kg; and MiniSIL for satellites in the range 100–500 kg. Their architecture is based on the semi‐standard sub‐systems, but easily modified to utilize sub‐systems from other manufacturers. They can support all stabilization methods including spinning, three‐axis control and gravity gradient and are adaptable to a wide variety of missions including Earth resources, scientific, communications and technology demonstration. The company also manufactures a range of low cost ground support equipment and complete ground stations to complement the space‐borne systems.
A fully autonomous magnetic attitude control system was proposed by Grassi (Citation1997) for a small satellite for remote sensing applications. The attitude determination hardware consists of a horizon sensor and a pair of two‐axis magnetometers. Three‐axis attitude control is performed by means of three magnetic torquers. Control laws are derived separately for the attitude acquisition and the station keeping phases. The proposed control strategy is then tested by using a numerical code which simulates the satellite orbit and attitude dynamics and control, and the sensor measurement errors. The final results show that the proposed magnetic control system limits the attitude angles and rates within values adequate for low/medium resolution remote sensing applications.
Bartlett et al. (Citation1995) described an approach to a space‐based ocean altimetry mission configured by Matra Marconi and Fairchild to perform a follow‐on of TOPEX/Poseidon. The space segment was composed of a subset of the TOPEX/Poseidon payload accommodated on a Fairchild Class II small satellite multimission platform. This combination included the advantages of a low cost satellite, short development schedule and high confidence in the mission success. The ground segment comprised the two classic function – the mission/control centre and the data processing centre. The design was such that a maximum of existing ground infrastructures and systems could be used so that a low cost was obtained and compatibility with existing data was simply achieved.
The FTIR (Fourier Transform InfraRed) spectrometer is devoted to the detection and study of the concentration and spatial distribution of atmospheric trace gases (e.g. CH4, SO2, NH3, HNO3, NO3, HCl) and of natural ozone in the wavelength band 2–16 µm with spectral resolution 0.1 cm−1 and in the height range between 10 and 70 km (±1 km) with vertical spatial resolution around 2 km. Measurements will take place during sunset and sunrise for absorption spectrum. The instrument will be looking at the Sun through the Earth's atmosphere. These measurements will be very useful in the study of the Earth's environment. Moreover, the instrument is used to study the designing and manufacturing of light and compact FTIR spectrometers for low‐cost small satellite missions. Rataj and Orleanski (Citation1996) presented the general description of the instrument and preliminary measurements.
A compact Fourier Transform Imaging Spectrometer (FTIS) for small satellite remote sensing missions is currently being studied under ESA contract with the objective to demonstrate its feasibility by breadboarding. Compared to classical hyperspectral imagers using dispersive spectrometers, the major advantages of the FTIS are the very compact optics module and the tolerable higher detector temperature, thus reducing the overall mass and easing the instrument's thermal design. Harnisch et al. (Citation2003) discussed the feasibility of this instrument concept, payload requirements to the satellite attitude stability and the data‐handling concept.
A history of in‐orbit operation of 15 low Earth orbit satellites by Surrey Space Centre has highlighted the usefulness to the civilian market of measuring and analysing the crowded radio frequency spectrum from the low Earth orbit perspective. A desirable instrument would be a payload that performs spectral analysis of a wide frequency range rapidly and to a useful resolution, while combining a capability for further time domain analysis of particular signals. A conventional system architecture adopts a channelized approach, sampling and processing each channel in turn over some repetition period. Whittaker and Hodgart (Citation2000) proposed a novel sweeping system which provides a more elegant and possibly more efficient solution. Digital chirp filter processing can be employed to achieve suitable resolution spectral analysis. They presented an explanation of the operation of a sweeping system, including chirp filter spectral analysis along with a comparison with a channelized approach.
The Optical Remote Sensing Small Satellite is for high‐tech flight demonstration's test and three‐dimensional mapping. Its system overview was presented in a paper by Cao et al. (Citation2004), and it included such items as mission objective and mission requirements, satellite system scheme, reliability, cost budget project schedule and management and operation. Topsat is a mission designed to demonstrate the capabilities of small satellites for classically high value remote sensing missions (Brooks Citation2003). The mission, conceived by the UK's Defence Evaluation and Research Agency (DERA), was designed to provide 2.5 m resolution imagery direct to users in the locale of the imaged area and allow the direct transmission of the image data to a local mobile ground station. Price (Citation2002) provided an overview of the mission and an update to the status of the mission as of July 2002 and described the Topsat satellite programme, focusing on the key technical, operational and commercial aspects of the mission. Topsat is designed to demonstrate the capabilities of small satellites for classically high value remote sensing missions. Topsat is a 120 kg, Sun‐synchronous satellite. It is an end‐to‐end mission encompassing development, build, launch, operation and exploitation. Its objectives are to demonstrate the capability to cost performance available from small satellites and the utility of direct tasking and reception of remotely sensed imagery. The Rutherford Appleton Laboratory is developing a near diffraction limited, obstruction free camera which, when combined with an agile SSTL spacecraft capable of pitch compensation, allows highly demanding optical performance characteristics to be achieved. Infoterra (formerly NRSC (National Remote Sensing Centre)) are responsible for developing these potential markets for Topsat. Demonstration of such a high capability at very low cost finally tackles the most significant problem with commercial remote sensing from space – timeliness – by providing a means for affordable constellations of satellites.
A great many small satellite systems are based on a constellation of tens (in some cases hundreds) of small satellites to be launched over the next few years. Consequently, large numbers of spacecraft need to be produced in a very short time. The traditional concept for space production are no longer adequate to meet the requirements of these new systems and so a new approach to Assembly, Integration and Testing (AIT) is needed that satisfies the intensive production rate demands but without introducing complete process automation (Simeone and Pignatelli Citation1997). It is expected that Topsat will be exploited both through its imagery and through the demonstration of affordability of constellations and individually owned assets.
4. Earth observation applications of small satellites
The concept of the Teaching Company Scheme Satellite (TuCSAT), has been developed to meet the requirements of the Earth observation user community for inexpensive and flexible opportunities to launch remote sensing instruments into low Earth orbit. Gardner et al. (Citation1996) described the satellite design process, together with the philosophy behind the selection of a baseline mission. The satellite was shown to demonstrate the ability to meet a wide range of requirements within a strict low mass and low cost philosophy, while making use of currently available technology in order to achieve the design aims.
4.1 Hot spot and fire detection
High temperature events strongly influence the environmental processes. Therefore, their observation is an important constituent of the global monitoring network. Unfortunately the current remote sensing systems are not able to deliver the necessary information about the worldwide burn out of vegetation and its consequences. For global observations a dedicated system of small satellites is required. The main components of the corresponding instrumentation are the infrared channels. The proposed HSRS (Hot Spot Recognition Sensor) has to demonstrate the possibilities of such an instrumentation and its feasibility for small satellites (Skrbek et al. Citation1996). The main drawbacks of the HSRS design are the handling of the hot spot recognition in the subpixel area and of the saturation in the case of larger hot areas by a suitable signal processing hardware.
For forest fire detection with operative spaceborne systems, the simultaneous imaging in various spectral channels is of high importance. With existing space systems, the related very large data volume needs substantial transmission and interpretation efforts. There are some difficulties in the time coverage for efficient use of data in fire management. A fire classification depending on the temperature is not possible due to saturation of the sensors. Existing spaceborne instruments are not designed for measurement of fire parameters. To overcome these drawbacks, a small multi‐sensor system was proposed which is suitable for small satellites (Briess et al. Citation1996). Starting from the mission objectives and the requirements on the instrumentation for fire detection the principal structure of the sensor system and the orbit definition were developed. The parameters of the model payload and the structure of the signal processing for fire detection were given. A dedicated spacecraft design based on defined system parameters showed the feasibility of fire investigation from space by means of small satellite missions.
Wildfires have a range of significant environmental effects with respect to both the Earth's surface and atmosphere. Spaceborne remote sensing of active fires has been undertaken for more than two decades, but the Bispectral InfraRed Detection (BIRD) Experimental Small Satellite (2001–2004) was the first mission dedicated to this task. With the successful launch of the BIRD satellite in October 2001, new possibilities of the observation of hot events like forest fires, volcanic eruptions, etc. from space were opened up. The BIRD was the first satellite equipped with space instrumentation dedicated to recognize high temperature events. Current remote sensing systems have the disadvantage that they were not designed for the observation of hot events. This mission aims to answer technological and scientific questions related to the operation of a compact bi‐spectral infrared push‐broom sensor and related to the detection and investigation of fires from space. The payload of BIRD is a multi‐sensor system designed to fulfil the scientific requirements under the constraints of a micro‐satellite. Skrbek et al. (Citation2002) described the basic ideas for fire detection and the estimation of fire temperature, fire size, and energy release in the sub‐pixel domain and described the technical solution for the infrared sensor system on board BIRD.
Starting with the FIRES Phase A Study, the principal requirements and ideas for a fire recognition system were defined. With the German BIRD demonstrator mission, a feasible approach of these ideas has been realized and carried out in space. Zhukov et al. (Citation2006) summarized the experience gained during the BIRD mission, which has focused both on active fire detection and active fire characterization, in terms of quantifying effective fire temperature (T–F), effective fire area (A(F)) and fire radiative power (FRP). A detailed error analysis for each parameter is undertaken, and the accuracy of FRP retrieval is shown to be significantly better than that of T–F or A(F). For key fire‐affected forest, bush and savanna environments (Australia, Benin, Borneo, Brazil, Canada–USA, Portugal and Siberia) BIRD data allows FRP estimation to within 30% in 75% of fires examined, and for the first time from space BIRD is able to allow estimation of fireline length, effective fireline depth and radiative fireline intensity for the more pronounced fire fronts. Some indication of the predominant combustion regime (smouldering or flaming), which has implications for the relative concentrations of emitted pollutant products, is possible through use of the T–F parameter. This experience demonstrates the advantages of the new infrared sensor technologies employed in BIRD, and offers suggestions for future fire monitoring sensors based on similar technologies.
4.2 Environment monitoring and land surface survey
During the International Space Year in 1992, scientists, teachers and students exploited the global perspective provided by Earth‐observing satellites to better understand global environmental change and how it will affect humanity's future. One of the most dramatic expressions of environmental change can be seen in the patterns of human health and disease. To monitor and predict the human health consequences of environmental change, a growing segment of the public health community is advocating the use of satellite imagery to monitor environmental parameters that influence the spatial and temporal patterns of vector‐borne diseases. It is anticipated that a dedicated remote sensing surveillance capability will lead to the development of a ‘disease early warning system’ for identifying areas of high disease transmission risk and directing control measures. For malaria, an example relevant to many other vector‐borne diseases, key environmental parameters include the spatial and temporal patterns of vegetation type and condition, land use, standing water, and human settlements. Analysis of data from existing satellite systems (e.g. Landsat and France's Système Pour l'Observation de la Terre (SPOT)) suggests both potential opportunities and shortcomings in the ability to monitor these parameters in malarial areas by using remote sensing data. The shortcomings of current operational systems, including timeliness, lack of rapid accessibility to data, and spatial and temporal resolution, have stimulated interest in developing a satellite system designed to focus exclusively on the public health needs associated with the surveillance of vector‐borne diseases. Wood et al. (Citation1992) presented a preliminary set of design requirements for a remote sensing satellite dedicated to monitoring environmental parameters that influence the patterns of vector‐borne diseases. Also, a preliminary student engineering design for a satellite platform was presented and reviewed. The goal of this remote sensing system would be to provide near‐real‐time data acquisition and processing to public health users at a number of sites where malaria or other vector‐borne diseases are significant human health problems. The International Space Year provided an excellent forum in which to pursue discussions among public health investigators, remote sensing scientists, and aerospace engineers toward defining the technical design requirements for a system, including satellite platform, sensor characteristics, communications, and ground infrastructure.
The estimation of land surface fluxes has been recognized in the last 10 years as a major scientific issue for the improvement of our knowledge of heat and water budgets and therefore of models in meteorology, hydrology, agriculture and the environment. Remote sensing is an adequate means for filling the gap which exists between small‐scale instruments or modelling (10 m) and the regional or global scales where they have to be determined with a typical grid element of the order of 1–10 km. IRSUTE (Infra‐Red miniSatellite Unit for Terrestrial Environment) is a scientific small satellite mission providing thermal imagery for the determination and analysis of soil/vegetation/atmosphere processes at the field scale and therefore for providing the necessary data for a scaling‐up of these processes from local to regional scales (Becker et al. Citation1996). The main specifications will allow this instrument to optimize the correction of the sensed radiance and to retrieve the fluxes with an accuracy of the order of 50 W m−2. IRSUTE is designed to have high spatial resolution (50 m), across‐ and along‐track viewing capabilities, five channels: visible/NIR, 3.7 µm, and three TIR in the 8.2–11 µm band with a good radiometric sensitivity (noise‐equivalent temperature difference, NEΔT = 0.1 K). The instrument is to be implemented onboard a small satellite (typically a PROTEUS platform) placed on a Sun‐synchronous orbit allowing high repetitivity (1–3 days). It is based on the push‐broom technique which uses infrared CCD linear array detectors positioned in the cryocooled focal plane of large bandwidth collecting optics.
Suspended matter deposited in reservoirs reduces their storage capacity and suspended material reduces the light penetration in water, thus reducing fish production. The major factors affecting surface water quality are suspended sediments, chlorophyll, nutrients and pesticides. The standard traditional mapping and monitoring techniques have already become too expensive compared to the information achieved for environmental use. A solution could be to optimize our efforts and more frequently base our surveillance on remote sensing techniques to improve the information content and limit the cost. Remote sensing offers potentially a significant source of information and methods are being developed for operational large‐scale monitoring of water quality. MatJafri et al. (Citation2002) used satellite scene from Tiungsat‐1 for water quality mapping around Penang Island in Malaysia. This preliminary study indicated the possibility of using Tiungsat‐1 satellite image for monitoring coastal water quality (http://www.gisdevelopment.net/application/nrm/water/quality/watq0005.htm). Rufino and Moccia (Citation2002) presented these applications within the BISSAT mission, with particular reference to interferometric ones, showing the requirements on mission operation and discussing the expected results and performance.
The objective of ALCATEL ESPACE and AEROSPATIALE is to cooperate on the definition of a small satellite system using an imaging radar and then to propose to civilian customers, for a moderate cost, a round‐the‐clock and all‐weather dedicated system. The system is designed following a ‘faster, better and cheaper’ approach, based on off‐the‐shelf technologies, space qualified product lines and the large synergy between telecoms and radar space programmes (Vincent et al. Citation1998). The satellite is composed of a specific SAR (Synthetic Aperture Radar) imaging payload mounted on the PROTEUS multi‐mission platform, issued of a product line developed by AEROSPATIALE for CNES (the French Space Agency). The SAR payload designed by ALCATEL ESPACE is flexible enough to take, across a swath of 350 km, images of areas of about 20 km to 100 km width and several tens of kilometres in length with a resolution varying from a few metres for the smallest areas to several tens of metres for the largest ones. The ground system is based on standard systems with re‐use whenever possible of existing products.
Yang and Yang (Citation2002) conducted a feasibility study of using small satellite constellation to perform Interferometric Synthetic Aperture Radar (InSAR) global 3D imaging. A scheme in which three identical small satellites make up a constellation is given. Two of them fly along parallel orbits with slightly different ascending nodes and observe the same area and make interferometry without any time decorrelation. The third flies along another orbit with a certain angular separation behind the two satellites, and observes the same area in a short revisit time. These three satellites together can perform differential interferometry, which is of great benefit when observing surface changes with short time baseline. Their simulation results showed the potential of the system for accurate global three‐dimensional measurements.
4.3 Disasters
Small satellites are playing an increasingly essential role in the creation of systems for land mobile communications, for environmental observation and for scientific applications. Since the launch in October 2001 from SHAR/India the BIRD payload has been fully operational. Among others, forest fires (Australia), volcanoes (Etna, Chile) and burning coal mines (China) have been detected and their parameters such as size, temperature and energy release could be determined (Walter et al. Citation2005). As the status of the payload system is satisfactory it has the potential to be applied in new missions with the help of modern detector technology.
At the IAF Congress in 1992 a multiple small satellite Earth observation system was put forward with sensors of visible and infrared spectrums. The system could shorten the revisiting period so that any place in the world could be observed twice a day. Qiang et al. (Citation2000) extended the idea to the microwave remote sensing satellite system. The main purpose of the system was to forecast impending earthquakes. Through the observation of temperature increase of the low layer of atmosphere and its moving trend caused by some sorts of radiation and gases released from the Earth's interior, an impending strong earthquake could be predicted in time. As the temperature increase is detected by thermo‐infrared spectrum sensors on the meteorological satellites, the observation may sometimes be obstructed by cloud or rain. In the suggested system, mm‐wave radiometers are used and those obstructions could be generally overcome. Besides, radiometers of some other microwave frequencies are also included so as to make the small satellite system useful for observation of the atmosphere, soil and crops. The platform construction and the attitude control system of the satellites suitable for the sweeping radiometer antennas are closely coupled implemented. The orbit of the satellites in the system is well designed so that any place in the world could be observed twice a day in accordance with the optical small satellite system.
Remote sensing satellites can provide valuable inputs on disaster management both for an accurate prediction and for a rapid assessment of the location and extent of damage. Currently, several remote sensing satellites are orbiting the Earth, collecting large amount of data. Characteristic parameters of spaceborne remote sensing are spectral coverage, spectral resolution, revisit time and spatial resolution. The analysis of currently operating spaceborne infrastructure makes it clear that it covers global aspects of environmental monitoring excellently but is not well suited for disaster mitigation (Iglseder et al. Citation1995). This is mainly due to a low repetition in time and a relatively bad spatial resolution. For disaster monitoring, a growing demand for observations with high repetition rates between 0.5–1 h and 7 days and medium to high resolution in time between 10 m and 1 km is evident. Constellations of small satellites can close the gap, left by the existing systems, in a cost‐effective manner. Small satellites are characterized by low volume, a mass limited to 250–400 kg and a power consumption below 350 W. A design to cost is achieved by using subsystem components which are commercially available. Indicative are the short development time and the numerous launch possibilities, making small satellites highly attractive for constellations. Different constellations with 4–32 satellites were investigated. The main focus was given to constellations using Sun‐synchronous orbits. It will be inferred that a resolution in time of about half an hour can be achieved by a system comprising 32 satellites.
After a short introduction to the Totally Integrated Maritime Traffic Surveillance System concept Perrotta and Xefteris (Citation1996) examined the key issues related to three main problems concerning oil pollution at sea:
the early detection of accidental oil spills; | |||||
the progress of oil spills to support containment and/or clean‐up intervention; and | |||||
the identification of culprits in the case of intentional discharges, for law enforcement purposes. |
They discussed the contribution made by advanced remote sensing satellite constellations, equipped with a complement of suitable instruments for the specific test cases of the Mediterranean Basin and the North Sea. They also discussed in detail key system features that enable an effective monitoring of both oil spills and the identification of the ships responsible for environmental damages. Avanesov et al. (Citation1996) considered a problem of providing users with the necessary remote sensing data in the visible and near‐infrared spectral bands. The solution of the problem should increase spatial and spectral resolution for imaging from space, provide high periodicity of surveying the same sites on the Earth's surface and deliver spaceborne data to users in real time. This problem solution proposed was to use a cluster of small satellites and to implement the Local Space Service (LOCSS) programme. The main aspects of this concept were as follows:
optimization of remote sensing instrumentation parameters; | |||||
image data compression onboard a small spacecraft; | |||||
compressed data downlinking via the low rate radio channel; | |||||
direct reception of the image data by users at small cheap receiving stations; and | |||||
image data decompression and processing using personal computers and special processors. |
China is one of the countries struck by many kinds of natural disasters with high frequency, broad distribution and great losses, and environment protection is a very important task in the country. China is one of the most frequently disaster‐hit countries in the world, with about 200 million victims of various natural disasters every year according to the China International Committee for Natural Disaster (CICND). In 2003, natural disasters caused a financial loss amounting to 188.4 billion Yuan in China, equal to 1.6% of the country's gross domestic product. Natural disasters in China caused 2475 deaths and 204.2 billion Yuan (25.5 billion US dollars) of direct losses in 2005. Currently, China has more than 2000 environment‐monitoring ground stations nationwide which produce about 30 million environment monitoring‐related data. In order to take advantage of remote sensing technology in the field of disaster and environment monitoring, a proposal for the building of a satellite constellation system monitoring and forecasting disasters was put forward by the State Environmental Protection Administration of China, the National Commission for Disaster Reduction and the China Aerospace Corporation Organization. The Environment and Disaster Monitoring and Forecasting Small Satellite Constellation is the space segment of China's environment and disaster monitoring system, hereinafter called the ‘Huanjing constellation’. The satellite constellation system is listed as a key civilian satellite development in the ‘China Space Development White Paper’ published in 2001. In February 2003 China's State Council approved the satellite constellation project. The Small Satellite Constellation for Environment Protection and Disaster Monitoring (Huanjing constellation) will involve a network of eight affordable small satellites (two optical satellites and one SAR satellite were launched in 2006). The payload instrument on board includes a CCD camera, an infrared camera, a hyper‐spectral camera and an S‐band SAR. The average revisit time is 48 h. Bai et al. (Citation2004) introduced the 2+1 constellation under the development, including the application requirements, orbit selection, the payloads and platform design. The system performances are presented, including revisit interval, coverage period, instrument band, spatial and radiation resolution, swath, image location accuracy, signal and image processing requirement, etc.
The Disaster Monitoring Constellation (DMC) is an international programme initially proposed in 1996 and led by SSTL, to construct a network of five affordable low Earth orbit microsatellites. The objective is to provide a daily global imaging capability at medium resolution (30–40 m), in three or four spectral bands, for rapid‐response disaster monitoring and mitigation. The DMC consists of five remote sensing satellites constructed by SSTL and operated for the Algerian, Nigerian, Turkish, UK and Chinese governments by DMC International Imaging. The DMC provides emergency Earth imaging for disaster relief under the International Charter for Space and Major Disasters, which the DMC formally joined in November 2005. Other DMC Earth imagery is used for a variety of civil applications by a variety of governments. Spare available imaging capacity is sold under contract.
The individual DMC satellites are:
AlSAT‐1 (Algeria), launched November 2002; | |||||
BilSAT (Turkey), launched September 2003, and completed its mission in August 2006 due to failed battery cells; | |||||
NigeriaSAT‐1 (Nigeria), launched September 2003; | |||||
UK‐DMC (UK), launched September 2003; and | |||||
Beijing‐1 (China), launched October 2005. |
The Sun‐synchronous orbits of these satellites are coordinated so that the satellites follow each other around an orbital plane, ascending north over the Equator at 10:15 am local time (and 10:30 am local time for Beijing‐1).
Some of these satellites also include other imaging payloads and experimental payloads: on‐board hardware‐based image compression (on BilSAT), a GPS reflectometry experiment and onboard Internet router (on the UK‐DMC satellite). The DMC satellites are notable for communicating with their ground stations using the Internet Protocol for payload data transfer and command and control, so extending the Internet into space. Many of the technologies used in the design of the DMC satellites, including Internet Protocol use, were tested in space beforehand on SSTL's UoSAT‐12 satellite.
The DMC provides far larger areas of imagery than, but at comparable resolution to, established government imaging satellites such as Landsat. DMC imagery was deliberately designed to be comparable to Landsat imagery, in order to leverage the expertise and software of the large established remote sensing community which is familiar with working with Landsat images. Imagery can be provided far more rapidly from the DMC than from Landsat, thanks to having multiple similar satellites in orbit ready to cross over a point of interest, and the larger images produced. This brings the responsiveness that is needed for emergencies and for disaster support, with images provided across the Internet from the responsive satellite and a member country's ground station within a day or less of a request being made. Applications of the DMC are many and varied but of particular demand and suitability have been:
land cover and vegetation; | |||||
hydrology; | |||||
fire and burn scar mapping; and | |||||
flood monitoring. |
The DMC has monitored the effects and aftermath of the Indian Ocean Tsunami (December 2004), Hurricane Katrina (August 2005), and many other floods, fires and disasters.
5. Current and future
Future small satellite systems for both Earth observation as well as deep‐space exploration are greatly enabled by the technological advances in deep sub‐micron microelectronics technologies. Whereas these technological advances are being fuelled by the commercial (non‐space) industries, more recently there has been an exciting new synergism evolving between the two otherwise disjoint markets. In other words, both the commercial and space industries are enabled by advances in low‐power, highly integrated, miniaturized (low‐volume), lightweight, and reliable real‐time embedded systems.
5.1 Integration of small satellite constellations
To achieve an effective operational revisit frequency it is necessary to have several satellites coordinated in a constellation and to integrate different small satellite constellations. Historically, this desirable capability has been too expensive to implement because of the cost of the satellites. However, small low‐cost satellites are changing the equation. With a cost one‐tenth of the original satellites, and a much more rapid build–launch cycle, these are bringing Earth observation into a fully operational phase. The small satellites cannot carry as many instruments as the giant research satellites, but they can put multiples of the same instrument into a coordinated orbit at an affordable cost. This enables the provision of a daily revisit service that reduces the impact of cloud and enables business to build new services using Earth Observation data. Examples of satellite constellations include the Global Positioning System (GPS), Galileo and GLONASS constellations for navigation and geodesy, RASAT, the Disaster Monitoring Constellation (DMC), RapidEye, COSMO‐SkyMed (COnstellation of small Satellites for the Mediterranean basin Observation), and Huanjing constellation (the Small Satellite Constellation for Environment Protection and Disaster Monitoring) for remote sensing (table ).
Small satellite constellations open a new era in sustainable Earth observation. The next generation of small satellite constellation will have enhanced sensors, provide continuity data and extraordinary pace. The next improvement in the DMC is the increase in sensor resolution from 32 m GSD (ground sample distance) to 2 m GSD. The GSD refers to the size of the pixels in a digital orthophoto, expressed in ground units. For example, if an orthophoto has a 1.0 m GSD, each pixel represents a ground area measuring 1 m×1 m. The combination of multiple spacecraft in constellation provides for daily coverage at a resolution that enables effective monitoring of the rapidly changing environment.
The RASAT Project from BILTEN, Turkey, is funded by the State Planning Office as a research project for an 8 m resolution low Earth orbit Earth observation satellite. TUBITAK UZAY (formerly known as TUBITAK BILTEN – Information Technologies and Electronics Research Institute) has gained the capability to build small satellites in the framework of the BilSat technology transfer project. After the completion of the BilSat project, TUBITAK UZAY initiated the RASAT Earth Observation Satellite Project, which aims at building and launching the first domestic Earth observation microsatellite of Turkey. In April 2006, a preliminary design study of RASAT was already performed. It is expected to be launched in late 2008 and send images in early 2009.
RapidEye is a commercial multispectral Earth observation mission of RapidEye AG of Brandenburg, Germany, that includes a constellation of five mini‐satellites. The mission will provide high‐resolution multispectral imagery along with an operational GIS (Geographic Information System) service on a commercial basis. The objectives are to provide a range of Earth‐observation products and services to a global user community. The RapidEye sensor images five optical bands in the 400–850 nm range and provides 6.5 m pixel size at nadir. It provides products for the following applications:
Agricultural producers (farmers): crop monitoring and mapping, yield prediction. | |||||
Agricultural insurance: provision of regularly updated field maps to help insurers assess insurance contracts and claims by providing quick and reliable information about damaged areas. | |||||
Cartography – satellite‐based maps (scale 1:25 000), orthophotos, DEM (Digital Elevation Model) generation. | |||||
Other markets – disaster assessment, 3D visualization. | |||||
Service spectrum at completion mission: guaranteed daily revisit, global coverage, product delivery to the customer within 24 h, possibility of dedicated programming, capability of direct transmission and imagery transfer within hours, global digital database of ‘orthomaps’ of 1:25 000 scale and DEMs of 20 m×20 m resolution. The service also permits the merging of multi‐temporal imagery with information from other sources. |
The five RapidEye Earth observation satellites are planned to be launched on a single Russian Dnepr rocket from the Baikonur Cosmodrome in Kazakhstan at the beginning of 2008. They will be deployed in orbits at an altitude of 630 km. The satellites will be placed equally spaced in a single Sun‐synchronous orbit to ensure consistent imaging conditions and a short revisit time. The satellites follow each other in their orbital plane at about 19 min intervals. The constellation approach in a single orbital plane permits a cumulative swath to be built up (the spacecraft view adjacent regions of the ground, with image capture times separated by only a few minutes). A revisit time of 1 day can be obtained anywhere in the world (±70° latitude) with body pointing techniques. The average coverage repeat period over mid‐attitude regions (e.g. Europe and North America) is 5.5 days at nadir. The RapidEye system can access any area on Earth within a day and cover the entire agricultural areas of North America and Europe within 5 days.
The RapidEye satellite platforms are under construction by SSTL. This makes RapidEye the second multiple‐satellite imaging constellation that SSTL has been involved with, after building and launching the Disaster Monitoring Constellation. Taiwan's national space agency has hired Italian and German companies to assist in the design of a multimission Earth observation satellite platform ARGO, whose first application may be as part of the RapidEye commercial constellation.
China envisages deploying a constellation of satellites for the monitoring of the world environment. China plans to launch six small optical satellites and five small SAR satellites in the next few years to form a satellite constellation for environmental and disaster monitoring and prediction. The objective is to establish an operational system for disaster monitoring and mitigation using remote sensing technology and improve the efficiency of disaster mitigation and relief. Accompanying the construction of the small satellite constellation, a long term and stable national‐level disaster reduction operational system in China will be established, as well as the network system at regional and provincial levels. The network system will be a powerful support system for rapid‐response disaster monitoring and mitigation.
This first constellation of the name of Huanjing‐1 will be made up of two microsatellites with optical equipment (Huanjing‐1A and Huanjing‐1B) and of a microsatellite equipped with a RADAR with synthesis of opening (Huanjing‐1C). The average revisit period is 32 h, and the average global coverage time is 48 h. It will be managed by the administration for environmental protection (SEPA, State Environmental Protection Administration) and the National Committee for the Reduction of the Catastrophes (CNCDR, China National Committee for Disaster Reduction). The first phase of this deployment must make it possible to provide data for the study of integration of this constellation in the international systems of monitoring the planet. The constellation could thus form part of the project of system of climatic observation total (GOS, Global Observing System) of the world weather organization of the United Nations (WMO, World Meteorological Organization).
After that, four small optical satellites and four small SAR satellites will be launched by 2010 to realize a satellite visiting period of once every 12 h and the average global coverage time of 24 h. By the end of 2010, the complete Huanjing constellation (four optical satellites and four SAR satellites) will have been constructed (Wang et al. Citation2005). These launches fall under the Chinese project of information system made up from 60 to 70 satellites during the 11th 5‐year plan (2006–2010), with applications in various fields such as telecommunications, remote transmission, natural resources, scientific meteorology, navigation and experiments. The Huanjing satellite constellation will also be part of the anti‐disaster platform in the Asia‐Pacific region.
COSMO‐SkyMed (COnstellation of small Satellites for the Mediterranean basin Observation) is an Earth observation satellite system funded by the Italian Ministry of Research and Ministry of Defence and conducted by the Italian Space Agency (ASI), intended for both military and civilian use. The programme is managed in cooperation by ASI and MoD. The overall objective of this programme is global Earth observation and the relevant data exploitation for the needs of the military community as well as for the civil (institutional, commercial) community.
COSMO‐SkyMed is a four‐spacecraft constellation. Each of the four satellites is equipped with a SAR instrument and is capable of operating in all visibility conditions at high resolution and in real time with global coverage of the planet. The imagery will be applied to defence and security assurance in Italy and other countries, seismic hazard analysis, environmental disaster monitoring, and agricultural mapping. Sample applications of COSMO‐SkyMed data are seen in the following fields:
Defence and security applications: surveillance, intelligence, mapping, damage assessment, vulnerability assessment, target detection/localization. | |||||
Risk management applications: floods, droughts, landslides, volcanic/seismic, forest fire, industrial hazards, water pollution. | |||||
Other applications: marine and coastal environments, agriculture, forestry, cartography, environment, geology and exploration, telecommunication, utilities and planning. | |||||
Provision of commercial imaging services. | |||||
The high revisit frequency offered by the four X‐band SAR spacecraft is also expected to provide a unique potential to the operational meteorological user community through provision of ancillary data and/or data on meteo‐correlated phenomena, in particular as regards sea ice monitoring and study of ocean wave patterns. |
United Launch Alliance is providing launch services for the satellites. Satellite processing for the first two satellites is being handled by the Astrotech Space Operations subsidiary of SPACEHAB. The first satellite was launched at 22:34 GMT, on 8 June 2007, and the second satellite of the COSMO‐SkyMed constellation was launched at 02:30 GMT, on 9 December 2007, both aboard Delta II rockets from Vandenberg AFB. COSMO‐3 is scheduled to launch in summer 2008. All spacecraft of the SAR constellation will be positioned in the same orbital plane. The nominal repeat cycle is 16 days; however, each single satellite will have a near revisit time of 5 days. Although the swath width of the SAR instrument is between 10 and 200 km (depending on support mode), a total FOR (Field of Regard) of 1300 km in the cross‐track direction is available for event monitoring applications (for revisit times of less than 12 h for the full constellation). The nominal full constellation will achieve a revisit time of a few hours on a global scale.
In the framework of COSMO‐SkyMed follow‐on activities, ASI plans to design, develop and operate a bistatic and interferometric mission, SABRINA. The SABRINA mission concept uses a passive satellite, namely BISSAT (Bistatic and Interferometric SAR Satellite), which is to co‐orbit in close formation with one of the COSMO‐SkyMed constellation spacecraft.
The Galileo positioning system is a planned Global Navigation Satellite System, to be built by the European Satellite Navigation Industries for the European Union (EU) and European Space Agency (ESA) as an alternative to the US Global Positioning System (GPS) and the Russian GLONASS. The Galileo Operating Company, the concession holder and private consortium that was to run Galileo, was to have its main headquarters in Toulouse, France, with some specialized command centres also located in Munich (performance control), London (system operations), Rome (performance control), and Madrid (Safety of Life signals and redundancy control). It was reported on 18 May 2007 that, at the recommendation of Transport Commissioner Jacques Barrot, the EU will take direct control of the Galileo project from the private sector.
Galileo is intended to provide more precise measurements to all users than available through GPS or GLONASS, better positioning services at high latitudes, and an independent positioning system upon which European nations can rely even in times of war or political disagreement. The current project plan has the system as operational by 2011–2012, 3 or 4 years later than originally anticipated.
GLONASS (Global Navigation Satellite System) is a radio‐based satellite navigation system, developed by the former Soviet Union and now operated for the Russian government by the Russian Space Forces. The US counterpart is the GPS. Development on the GLONASS began in 1976, with a goal of global coverage by 1991. Beginning on 12 October 1982, numerous rocket launches added satellites to the system until the constellation was complete in 1995. Following completion, the system rapidly fell into disrepair with the collapse of the Russian economy. Beginning in 2001, Russia committed to restoring the system, and in recent years has diversified, introducing the Indian government as a partner, and accelerated the programme with a goal of restoring global coverage by 2009.
5.2 Processing on demand for the Earth science community
The service of small satellites for the remote sensing community is the key issue for the future along with the purpose‐built small satellites.
Scientists have the processing algorithms. What they need is data! This includes near‐real‐time data, decades of data, and multi‐source data. Space missions such as small satellite constellations generate huge amount data every day. Earth Observation data archive is ‘scattered’. With the improvement of algorithms, Earth Observation data needs recurrent reprocessing. Moving the data to the users is costly. Large investments are required to handle the data at the scientist's home. Using grid computing can solve the above issues by virtually moving processors close to the data in a flexible and controlled way. Resources can be shared (data, tools, computing resources) in order to provide a common shared platform and collaborative environment.
Grid computing can provide access to a global distributed computing environment via authentication, authorization, negotiation and security (Xue et al. Citation2005). It can create a computational environment handling many petabytes of geographically distributed data, tens of thousands of heterogeneous computing resources, and thousands of simultaneous users from many research institutions. In the meantime, it can provide a powerful tool for remote sensing data sharing and remote sensing data processing middleware sharing. Grid Processing on Demand for the Earth science community promotes the access and use of small satellite constellation Earth observation mission data available at geographically distributed constellation organizations, offering online access to products with attached computing infrastructure and tools to assist the generation of ‘scientific added value products’.
Telegeoprocessing can be defined as a new discipline based on real‐time spatial databases updated regularly by means of telecommunications systems in order to support problem solving and decision‐making at any time and any place. It involves the integration of remote sensing, Geographic Information Systems (GIS), GPS and telecommunications. The goals of telegeoprocessing are to improve real‐time access to geographic information from anywhere in the world and to enhance the overall quality of decision‐making at affordable costs (Xue et al. Citation2002). Improved access to information and cost savings could be achieved by allowing a scientist to examine the situation remotely or to consult with specialists. This reduces or eliminates the time and expense of travel necessary to bring the data and the scientists or specialists together. The quality of decision making is improved by providing the required services in a timely fashion and expanding the pool of specialists available to a given facility, making it more likely that a given case could be handled by experts if necessary.
Figure shows the systematic structure of Processing on Demand for Small Satellite Constellation systems. The system will be built in a grid computing environment. The system will be open, sizeable and secure. The special middleware should be constructed in order to manage the data sharing and processing resource sharing. The processing algorithms developed by scientists around the world will be stored in a repository and reused. Telegeoprocessing combined with grid computing will provide the infrastructure and technologies for the development of Processing on Demand for Small Satellite Constellation systems.
6. Conclusion
Satellites, like any complex system, require the application of a systematic, rational process for their design. Although the essential elements and overall structure of systematic design processes are common, each application may require some customization to be as effective as possible.
There has been a recent increase in emphasis on small satellites because of their low cost, short development times, relative simplicity, and cost efficiency. However, these satellites do have drawbacks. Their small size results in small surface areas, which often translate into payload, thermal and power constraints. A small satellite may not have enough surface area for radiators and/or solar panels. Small satellite constellations should rapidly expand their services to customers, who need a reliable and responsive source of imagery. The service has matured considerably since its inception as a result of the hard work and experience gained coordinating large numbers of satellites for challenging rapid‐response services, both commercial and disaster response. The next development, providing direct broadcast services, promises to provide a valuable data stream for end‐users in the Earth science community.
Acknowledgements
Dr Xue was supported by research projects Multi‐scale Aerosol Optical Thickness Quantitative Retrieval from Remotely Sensing Data at Urban Area (40671142) and Grid Platform Based Aerosol Monitoring Modelling Using MODIS Data and Middlewares Development (40471091) funded by NSFC, China, Multi‐sources Quantitative Remote Sensing Retrieval and Fusion (KZCX2‐YW‐313) funded by CAS, and 973 Project – Active and Passive Remote Sensing of Land Surface Ecological and Environmental Parameters by MOST, China.
References
- Alex , T. K. , Jain , Y. K. , Ganage , R. A. and Kurian , K. K. 1983 . Remote‐sensing payload for a small Indian Satellite. . Proceedings of the Society of Photo‐Optical Instrumentation Engineers , 363 : 82 – 87 .
- Alkalai , L. 2000 . Advanced microelectronics technologies for future small satellite systems. . Acta Astronautica , 46 : 233 – 239 .
- Anonymous . 1996 . Chinese detail small‐satellite efforts. . Aviation Week & Space Technology , 16 : 33 – 33 .
- Anonymous . 2003 . Mitsubishi plans bigger small experimental satellite. . Aviation Week & Space Technology , 4 : 61 – 61 .
- Askar , M. and Tekinalp , O. 2000 . Turkish Small Satellite Program: goals and policies. . Acta Astronautica , 46 : 375 – 378 .
- Avanesov , G. A. , Ziman , I. L. and Krasnopevtseva , E. B. 1996 . LOCSS and its implementation on the basis of a small satellite cluster. . Acta Astronautica , 39 : 1025 – 1032 .
- Bai , Z. G. , Yu , D. Y. and Yang , F. 2004 . “ Small satellite constellation and its performance for disaster and environment monitoring. ” . In Proceedings of the World Engineers' Convention held in Shanghai, China , 528 – 533 . Dusseldorf : VDI Verlag . 2–6 November 2004, Vol D, Environment Protection and Disaster Mitigation
- Bartlett , R. O. , Vialet , C. and Pawlak , D. 1995 . Advanced small satellite concepts take maximum advantage from advances in technology. . Acta Astronautica , 35 : 55 – 64 .
- Becanovic , V. , Eklund , U. , Grahn , S. , Lindblad , T. , Lundin , R. , Lindsey , C. S. , Norberg , O. , Waldemark , J. and Waldemark , K. 1999 . “ HUGIN – a small satellite trying to be intelligent. ” . In Proceedings of the Society of Photo‐Optical Instrumentation Engineers (SPIE) , Edited by: Lindblad , T , Padgett , M. L and Kinser , J . 98 – 107 . Bellingham, Washington : SPIE . Stockholm: 9th Workshop on Virtual Intelligence/Dynamic Neural Networks
- Becker , F. , Seguin , B. , Phulpin , T. and Durpaire , J. P. 1996 . IRSUTE, a small satellite for water budget estimate with high resolution infrared imagery. . Acta Astronautica , 39 : 883 – 897 .
- Boland , L. , Brown , A. J. B. , Ward , A. K. and Wicks , A. 1999 . “ The MicroSIL and MiniSIL small satellite buses. ” . In Euro‐Asian Space Week – Co‐Operation in Space – Where East & West Finally Meet , 441 – 447 . Paris : ESA . Singapore, 23–27 November 1998, ESA Special Publication 430
- Briess , K. , Barwald , W. , Gerlich , T. , Jahw , H. , Lura , F. and Studemund , H. 2000 . The DLR small satellite minion BIRD. . Acta Astronautica , 46 : 111 – 120 .
- Briess , K. , Jahn , H. , Ginati , A. and Sommer , K. 1996 . A small satellite solution for the fire detection and monitoring from space. . Acta Astronautica , 39 : 447 – 457 .
- Brooks , P. 2003 . Topsat – High resolution imaging from a small satellite. . Acta Astronautica , 52 : 905 – 914 .
- Cao , X. B. , Zhang , F. , Lin , X. H. , Sun , Z. W. and Xu , G. D. 2004 . Optical remote sensing small satellite project. . Acta Astronautica , 54 : 139 – 143 .
- Carayannis , E. G. and Roy , R. I. S. 1998 . “ The speed and acceleration of technological innovation in the small satellite manufacturing industry: a co‐opetitive dynamics perspective. ” . In Pioneering New Technologies: Management Issues and Challenges in the Third Millennium, Proceedings, San Juan, Puerto Rico , Edited by: Peters , L. S . 221 – 230 . New York : IEEE . 1998
- Carayannis , E. G. and Roy , R. I. S. 2000 . Davids vs Goliaths in the small satellite industry: the role of technological innovation dynamics in firm competitiveness. . Technovation , 6 : 287 – 297 .
- Creasey , R. and Whitcomb , G. P. 1999 . Small satellite projects in the European Space Agency (ESA). . Proceedings of the Institution of Mechanical Engineers Part G – Journal of Aerospace Engineering , G4 : 201 – 212 .
- Cvetkovic , S. R. and Robertson , G. J. 1993 . Spacecraft design considerations for small satellite remote‐sensing. . IEEE Transactions on Aerospace and Electronic Systems , 2 : 391 – 403 .
- Dale , D. , Jagtman , F. A. and Deak , T. 1994 . Review of industry ESA business practices within the scientific program – the industrial workshops. . ESA Bulletin – European Space Agency , 78 : 7 – 14 .
- D'Errico , M. , Grassi , M. and Vetrella , S. 1996 . A bistatic SAR mission for Earth observation based on a small satellite. . Acta Astronautica , 39 : 837 – 846 .
- Dobrozrakov , A. , Kondranin , A. , Romanov , A. and Romanovsky , Y. 1996 . The analysis of investments in demonstrative small satellite projects for remote sensing. . Acta Astronautica , 39 : 933 – 942 .
- Galeazzi , C. 2000 . PRIMA: a new, competitive small satellite platform. . Acta Astronautica , 46 : 379 – 388 .
- Gampe , F. , Cinotti , C. , Lippner , G. and Faulks , H. 1995 . Small‐and‐smart satellite design concepts using advanced technologies for civil monitoring missions. . Space Technology – Industrial and Commercial Applications , 1 : 11 – 17 .
- Gardner , S. J. , Swinerd , G. G. and Ward , A. K. 1996 . The design of a small satellite for earth observation. . Proceedings of the Institution of Mechanical Engineers. Part G – Journal of Aerospace Engineering , G4 : 323 – 332 .
- Garrido , B. , Alfaro , N. , de Miguel , J. and Garcia , A. 2000 . “ Software subsystem and life cycle model for a small earth‐observation satellite. ” . In DASIA 2000: Data Systems in Aerospace, Proceedings , Edited by: Schurmann , B. 265 – 269 . Paris : ESA . Montreal, Canada, 2000, ESA Special Publication 457
- Grassi , M. 1997 . Attitude determination and control for a small remote sensing satellite. . Acta Astronautica , 40 : 675 – 681 .
- Harnisch , B. , Posselt , W. , Holota , K. , Tittel , H. O. and Rost , M. 2003 . Compact Fourier‐Transform Imaging Spectrometer for small satellite missions. . Acta Astronautica , 52 : 803 – 811 .
- Hu , W. R. and Du , H. 1994 . The scientific project of the small satellite of China. . Acta Astronautica , 32 : 687 – 691 .
- Iglseder , H. , Arensfischer , W. and Wolfsberger , W. 1995 . Small satellite constellations for disaster detection and monitoring. . Advances in Space Research , 11 : 79 – 85 .
- Jantarang , S. 2000 . “ Thai‐Paht, the small satellite for education. ” . In Remote Sensing for Tropical Ecosystem Management , 37 – 41 . New York : UN . 25–29 October 1999, Rangoon, Myanmar
- Kayal , H. 2000 . An experimental low‐cost ground station for the small satellite project BIRD. . Acta Astronautica , 46 : 213 – 220 .
- Konecny , G. 2004 . Small Satellites – A Tool for Earth Observation? . Available online at: www.isprs.org/istanbul2004/comm4/papers/428.pdf (accessed 21 November 2007)
- Kramer , H. J. 2007 . “ An overview of small satellite in remote sensing. ” . In Proceedings of International Workshop on Earth Observation Small Satellites for Remote Sensing Applications Kuala Lumpur, Malaysia, 2007 CD, Universiti Teknologi Malaysia, Johor Bahru
- Kramer , H. J. and Cracknell , A. P. 2008 . An overview of small satellites in remote sensing. . International Journal of Remote Sensing , 29 : 4285 – 4337 .
- Liddle , D. , Davies , P. , Jason , S. , Paffett , J. , Underwood , C. and Sweeting , M. 2004 . A low‐cost geostationary minisatellite platform. . Acta Astronautica , 55 : 271 – 284 .
- Liebig , V. 2000 . Small satellites for earth observation – the German small satellite programme. . Acta Astronautica , 46 : 81 – 86 .
- Marklund , G. , Andre , M. , Lundin , R. and Grahn , S. 2004 . The Swedish small satellite programme for space plasma investigations. . Space Science Reviews , 3–4 : 377 – 413 .
- MatJafri , M. Z. , Abdullah , K. and Lim , H. S. 2002 . Malaysian Tiungsat‐1 Imagery for Water Quality Mapping. . Available online at: http://www.gisdevelopment.net/application/nrm/water/quality/watq0005.htm (accessed November 2007)
- Moccia , A. , Vetrella , S. and Bertoni , R. 2000 . Mission analysis and design of a bistatic synthetic aperture radar on board a small satellite. . Acta Astronautica , 47 : 819 – 829 .
- Morring , F. 2001 . At Surrey Satellite Technology, small and simple is beautiful. . Aviation Week & Space Technology , 25 : 209 – 209 .
- Neeck , S. P. , Magner , T. J. and Paules , G. E. 2005 . NASA's small satellite missions for Earth observation. . Acta Astronautica , 56 : 187 – 192 .
- Ono , M. , Machida , T. and Moriyama , T. 1998 . “ Mission studies of Japanese small satellite. ” . In Proceedings of the Society of Photo‐Optical Instrumentation Engineers (SPIE) – Sensors, Systems, and Next‐Generation Satellites II , Edited by: Fujisada , H. Vol. 3498 , 162 – 172 . Bellingham, Washington : SPIE . Barcelona, Spain, 1998
- Orii , T. , Ono , T. , Aoyama , J. I. , Todome , K. and Shibayama , Y. 2002 . Development of versatile small satellite. . Acta Astronautica , 50 : 557 – 567 .
- Ousley , G. W. 2003 . NASA/international small satellite programme. . Acta Astronautica , 53 : 771 – 777 .
- Perrotta , G. and Xefteris , P. 1996 . Small synthetic aperture radar satellite constellations for tracking oil spills. . Spill Science & Technology Bulletin , 1–2 : 73 – 82 .
- Price , M. E. 2002 . “ Topsat – A small satellite approach to high resolution optical imaging. ” . In Proceedings of the Society of Photo‐Optical Instrumentation Engineers (SPIE) – Earth Observing Systems VII , Edited by: Barnes , W.L. Vol. 4814 , 162 – 172 . Bellingham, Washington : SPIE . Seattle, Washington, U.S.A., 2002
- Qiang , Z. J. , Chen , F. Y. , Zhang , J. R. , Lin , L. X. and Lu , Z. L. 2000 . Suggestion of EFS‐small satellite system for impending earthquake forecast. . Chinese Science Bulletin , 2 : 189 – 192 .
- Ramakrishnan , S. , Sharma , S. V. and Sowmianarayanan , L. 1999 . “ Small satellite launch opportunities on PSLV. ” . In Euro‐Asian Space Week – Co‐Operation in Space – Where East & West Finally Meet , 547 – 556 . Paris : ESA . Singapore, 23–27 November 1998, ESA Special Publication 430
- Rataj , M. and Orleanski , P. 1996 . “ Fourier Transform InfraRed spectrometer for atmosphere monitoring for the small satellite mission CESAR. ” . In Proceedings of The Society Of Photo‐Optical Instrumentation Engineers (SPIE) – Satellite Remote Sensing and Modeling of Clouds and the Atmosphere , Edited by: Fischer , J. Vol. 2961 , 98 – 106 . Bellingham, Washington : SPIE . Taormina, Italy, 24 September 1996
- Rufino , G. and Moccia , A. 2002 . “ Scientific applications of a bistatic radar mission based on a small satellite. ” . In Proceedings of the Society of Photo‐Optical Instrumentation Engineers (SPIE) – SAR Image Analysis, Modeling, and Techniques , Edited by: Posa , F. Vol. 4543 , 1 – 12 . Bellingham, Washington : SPIE . Toulouse, France, 2001
- Sandau , R. 2006 . International Study of Cost‐effective Earth Observation Missions , London : Taylor & Francis .
- Simeone , A. and Pignatelli , F. 1997 . “ Small satellite centre. ” . In Third International Symposium on Environmental Testing for Space Programmes, Noordwijk, Netherlands Vol. 408 , 183 – 188 . 1997, T.D. Guyenne (Ed.), ESA Special Publications
- Skrbek , W. , Briess , K. , Oertel , D. , Lorenz , E. , Walter , I. and Zhukov , B. 2002 . “ Sensor system for fire detection on‐board the small satellite BIRD. ” . In Proceedings of the Society of Photo‐Optical Instrumentation Engineers (SPIE) – Infrared Spaceborne Remote Sensing X, Seattle, Washington , 23 – 36 . Bellingham, Washington : SPIE . 2002, M. Strojnik (Ed.), Vol. 4818
- Skrbek , W. , Bachmann , K. , Lorenz , E. , Neidhardt , M. , Peschel , M. , Walter , I. and Zender , B. 1996 . Infrared sensor for hot spot recognition for a small satellite mission. . Acta Astronautica , 39 : 741 – 749 .
- Smithies , C. , Sun , W. and Sweeting , M. 2003 . “ Success of ‘faster, better, cheaper’ relies on ‘Surrey Low Cost Small Satellite System Engineering’. ” . In 34th Symposium on Space Safety, Rescue and Quality , Edited by: Reid , M. S. and Romero , M. Vol. 106 , 13 – 22 . Paris : International Astronautical Federation . Toulouse, France, 2001
- Swinerd , G. 1999 . Special issue on small satellite engineering. . Proceedings of the Institution of Mechanical Engineers. Part G – Journal of Aerospace Engineering , G4 : I – II .
- Tastemain , C. 1995 . France thinks small for future satellite plans. . Nature , 6539 : 377 – 377 .
- Thomsen , P. L. and Hansen , F. 2000 . The Danish Small Satellite Programme is a reality! . Acta Astronautica , 46 : 97 – 100 .
- Urech , A. , Acedo , L. , Caruso , D. and Yelos , J. 2000 . “ CESAR: a small satellite mission for earth observation. ” . In ESA Special Publications – DASIA 2000: Data Systems in Aerospace, Proceedings , Edited by: Schurmann , B. Vol. 457 , 531 – 535 . Paris : ESA . Montreal, Canada, 2000
- Wang , X. L. , Wang , G. , Guan , Y. , Chen , Q. and Gao , L. R. 2005 . “ Small Satellite Constellation for Disaster Monitoring in China. ” . In IGARSS 2005: IEEE International Geoscience and Remote Sensing Symposium , Vol. 1–8 , 467 – 469 . Piscataway, NJ : IEEE . 25–29 July 2005, Seoul, South Korea
- Ward , A. K. , Barrington‐Brown , A. J. and Gardner , S. J. 1996 . Mature systems for small satellite missions. . Acta Astronautica , 39 : 961 – 970 .
- Webb , G. M. , Fadeev , A. and Pestmal , N. 2006 . The inexpensive injection of small satellites into GEO using Russian/Ukrainian solutions. . Acta Astronautica , 59 : 560 – 569 .
- Whittaker , P. E. and Hodgart , M. S. 2000 . “ Small satellite SIGINT payload. ” . In Proceedings of the IEEE 2000 National Aerospace and Electronics Conference – Engineering Tomorrow , 625 – 632 . Piscataway, NJ : IEEE . 10–12 October 2000, Dayton, Ohio, U.S.A
- Vibulsresth , S. , Musigasarn , W. , Ditsariyakul , P. and Suchaichit , W. 1997 . Thai remote sensing small satellite programme. . Acta Astronautica , 41 : 91 – 94 .
- Vincent , N. , Gaillard , D. and Banos , T. 1998 . “ Small satellite system for civilian radar imagery application. ” . In IGARSS ‘98–1998 International Geoscience and Remote Sensing Symposium, Proceedings , Edited by: Stein , T. I. Vol. 1–5 , 274 – 276 . Piscataway, NJ : IEEE . Seattle, Washington, U.S.A., 1998
- Walter , I. , Briess , K. , Baerwald , W. , Lorenz , E. , Skrbek , W. and Schrandt , F. 2005 . A microsatellite platform for hot spot detection. . Acta Astronautica , 56 : 221 – 229. (doi: 10.1016/j.actaastro.2004.09.009) .
- Wood , B. L. , Beck , L. R. , Lawless , J. G. and Vesecky , J. F. 1992 . Preliminary considerations for a small satellite to monitor environmental‐change associated with vector‐borne disease. . Journal of Imaging Science and Technology , 5 : 431 – 439 .
- Xue , Y. , Cracknell , A. P. and Guo , H. D. 2002 . Telegeoprocessing: the integration of remote sensing, Geographic Information System (GIS), Global Positioning System (GPS) and telecommunication. . International Journal of Remote Sensing , 9 : 1851 – 1893 .
- Xue , Y. , Wang , J. Q. , Wang , Y. G. , Wu , C. L. and Hu , Y. C. 2005 . Preliminary study of grid computing for remotely sensed information. . International Journal of Remote Sensing , 16 : 3613 – 3630 .
- Yang , Z. and Yang , R. L. 2002 . “ Feasibility study of using small satellite synthetic aperture radar for global 3D imaging. ” . In Proceedings of the IEEE International Geoscience and Remote Sensing Symposium and 24th Canadian Symposium on Remote Sensing 3162 – 3164 . Toronto, Canada, 2002
- Zhang , X. M. , Xie , B. , Dai , S. L. , Zhang , W. W. , Hu , G. F. and Lan , D. 2007 . First micro‐satellite and new enhanced small satellite series in DFH Satellite Co. Ltd. . Acta Astronautica , 61 : 234 – 242 .
- Zhukov , B. , Lorenz , E. , Oertel , D. , Wooster , M. and Roberts , G. 2006 . Spaceborne detection and characterization of fires during the bi‐spectral infrared detection (BIRD) experimental small satellite mission (2001–2004). . Remote Sensing of Environment , 1 : 29 – 51 .