Abstract
This study was carried out to determine the effect of autophagy modulation in radiation treatment of cervical cancer cells. HeLa and CaSki cells were irradiated with γ-rays (2 Gy/min) after treatment with an autophagy inducer (rapamycin) and inhibitor (3-MA). Expression of LC3 and cell death in two cell preparations were examined. In addition, expression of Caspase-3 and PARP were examined after radiation alone and with autophagy inhibitor treatment. A notable increment of LC3 expression was detected after radiation in both cell lines. Cell viability was observed to decrease in 3-MA-treated cells compared to radiation alone, and even further in rapamycin-treated cells. Apoptosis was confirmed to occur later than autophagy in radiation treatment, and inhibition of autophagy derived a decrease in apoptosis. In conclusion, radiation-induced autophagy may be regulated by modulators, and autophagy augmentation yields an increase in cervical cancer cell death under radiation.
What is already known on this subject? Autophagy is known to contribute both to tumour cell survival and death against radiation therapy. The effect of induction or inhibition of radiation-induced autophagy on cervical cancer cell death is not clear.
What the results of this study add? Cell viability was observed to decrease in 3-MA-treated cells compared to radiation alone, and even further in rapamycin-treated cells. Apoptosis occurred later than autophagy in radiation treatment, and inhibition of autophagy derived a decrease in apoptosis.
What the implications are of these findings for clinical practice and/or further research? Our results suggest that radiation-induced autophagy may be regulated by modulators, and autophagy augmentation yields an increase in cervical cancer cell death under radiation.
Impact statement
Introduction
Dramatic advances have been made in the survival of early-stage cervical cancer over the past several decades, as prevention efforts, diagnosis and treatment options have been developed (Bogani et al. Citation2022, Giannini et al. Citation2022). However, advanced or recurrent cervical cancer patients still have few choices owing to the chemoresistance or radioresistance of cancer cells. Overcoming these resistances to therapeutic agents is a common mission in oncology, and novel therapeutic modalities are now being investigated based on translational research. Among the promising topics is autophagy.
Autophagy, which in Greek means “to eat oneself,” is a highly conserved catabolic process that employs double-membraned vesicles called autophagosomes to engulf intracellular proteins, lipids and organelles, and deliver them to lysosomes for degradation (Rabinowitz and White Citation2010). Then, degraded materials are exported back into the cytoplasm and recycled, making this process essential for cell survival, differentiation, development and after all, homeostasis. Autophagy is maintained at a low basal level, but it is significantly induced by stress or starvation, serving as a protective mechanism against diverse pathologies including cancer (Levine and Kroemer Citation2008). Thus, autophagy can be tumour suppressive by eliminating oncogenic substrates, but it can also be tumour promoting as well, as it acts to maintain the survival of the established tumour cell itself (White Citation2012).
Radiation is one of the most essential treatments modalities of advanced or recurrent cervical cancer, and either directly by interfering, or indirectly by free radicals derived from the water component of the cells, radiation damages cellular DNA and eventually leads to cell death (Jackson and Bartek Citation2009). When it comes to cancer cells, autophagy occurs to maintain stability and promote survival against radiation, therefore relating to radioresistance. Practically, several clinical trials have demonstrated the potential benefit of inhibiting autophagy in radiation treatment of glioblastoma, as well as brain metastasis of non-small-cell lung cancer and breast cancer (Levy et al. Citation2017). As a representative example, in phase III prospective randomised controlled trial conducted to examine the effect of autophagy inhibition in radiation combination treatment of glioblastoma multiforme, patients who were administered chloroquine (autophagy inhibitor acting to block the fusion of autophagosomes with lysosomes) had a significantly prolonged median survival compared to controls (33 months vs. 11 months, p < 0.0002) (Briceno et al. Citation2003).
On the other hand, when the autophagic activity becomes excessive and degradation overwhelms the capacity of a cell, autophagy paradoxically results in cell death, so-called type II programmed cell death (Gozuacik and Kimchi Citation2004). In this regard, studies intensifying autophagy in radiation treatment to derive more tumour cell death also have been performed, and corresponding results have been drawn in many cancer cell lines including glioma, prostate cancer, lung cancer and breast cancer (Xin et al. Citation2017).
In this study, we inquired about the regulation of radiation-induced autophagy relating to radiosensitivity in cervical cancer cell lines. To investigate the topic, we first identified radiation-induced autophagy and autophagy regulations with modifiers, then cell viability after radiation in each condition. Moreover, we investigated the relationship between autophagy and apoptosis in radiation-treated cervical cancer cells.
Materials and methods
Cell culture, reagent and γ-irradiation
This study was conducted with the approval of the Institutional Review Board of Seoul St. Mary’s Hospital (KC18SESI0567). HeLa and CaSki human cervical cancer cell lines were cultured and maintained in Dulbecco’s Modified Eagle Medium containing foetal bovine serum at 37 °C under 5% CO2. Cells were irradiated using a γ-ray at a dose rate of 2 Gy/min. Rapamycin and 3-methyladenine (3-MA) were purchased from Sigma-Aldrich (St. Louis, MO, USA).
Western blotting
Cells were harvested and reacted in a lysis buffer at 4 °C for 30 minutes and then dissolved. The cell lysate was centrifuged at 12,000 rpm at 4 °C, and protein was harvested. The protein was boiled for five minutes, subjected to 10% sodium dodecyl sulphate polyacrylamide gel electrophoresis (SDS-PAGE), and transferred to a polyvinylidene difluoride (PVDF) membrane. The membranes were blocked with 5% skim milk and tris buffered saline with Tween 20 (TBS-T) solution for an hour, then incubated with primary antibodies against light chain (LC) 3 (Santa Cruz Biotechnology, Santa Cruz, CA, USA), Caspase-3 (Santa Cruz Biotechnology), poly (ADP-ribose) polymerase (PARP) (Cell Signalling Technology, Beverly, MA, USA) and actin (Cell Signalling Technology) overnight at 4 °C. Each membrane was rinsed with the tris buffered saline and TBS-T, and reacted with the horseradish peroxidase-conjugated secondary antibodies (anti-mouse IgG, Santa Cruz Biotechnology) at room temperature for an hour. Then, the membrane was rinsed with TBS-T three times. The specific protein bands were obtained using the ECL Western blotting system (Amersham, Piscataway, NJ, USA).
LC3 transfection and fluorescence microscopy
For confirmation of autophagy, HeLa and CaSki cells were labelled with green fluorescent protein (GFP)-LC3, and GFP- LC3 spots were examined with a confocal microscope (Olympus Fluo View 1000, Olympus, Melville, NY, USA). Cells were transiently transfected into a pCMV-LC3-GFP expression vector (Cell Biolab, San Diego, CA, USA), irradiated with 2 Gy, and incubated for 36 hours. Then, they were rinsed with phosphate-buffered saline (PBS) and fixed with 4% paraformaldehyde for 10 minutes at 4 °C. For permeabilization and blocking, cells on the cover glass were incubated with 0.2% Triton X-100 on ice for 20 minutes, then with 1% bovine serum albumin for 1 hour at 37 °C. After adding one drop of 4′, 6-diamidino-2-phenylindole dihydrochloride (DAPI) containing mounting solution, cells were analysed using fluorescence microscopy.
Transmission electron microscopy
Cells were fixed in 3% glutaraldehyde for six hours, washed in 0.1 M phosphate buffer, and embedded into 2.5% agarose, which again was rinsed in 0.1 M Sorensen’s phosphate buffer. Post-fixation of cells was performed with 1% OsO4 in 0.1 M phosphate buffer, and cells were dehydrated by an ethanol series for 10 minutes. The dehydrated specimens were incubated in propylene oxide for 30 minutes, in a mixture of Epon resin and propylene oxide (1:1) for 1 hour and finally in pure Epon for one hour. Samples were embedded in pure Epon. Epon polymerisation was performed at 37 °C for 12 hour and then 80 °C for 48 hours. Ultrathin sections (70-100nm) were cut with a diamond knife (Leica, Wetzlar, Germany) by an ultramicrotome (Reichert Ultradcut S, Leica) and transferred to copper and nickel grids (HR23 Maxtaform, Plano, Wetzlar, Germany). Negative staining by uranyl acetate and lead citrate (all EMS, Munich, Germany) was performed to enhance transmission electron microscopy contrast. The specimens were viewed using a Zeiss Leo-906 electron microscope (Oberkochen, Germany), operated at an acceleration voltage of 60 kV.
Cell viability assay
Following radiation and autophagic modulator treatment, cell viability was determined using the 3-(4,5-dimethylthiazol-2-yl)-2,5-diphenyltetrazolium bromide (MTT; Sigma-Aldrich, St. Louis, Mo, USA) assay. HeLa and CaSki cells were seeded in 96-well plates, MTT was added at a dose of 20 μl/well, and the plates were incubated at 37 °C for one hour. The cells were then re-suspended in dimethyl sulfoxide (DMSO). Absorbance was measured at 570 nm using an enzyme-linked immunosorbent assay (ELISA) reader (Molecular Devices Co., Sunnyvale, CA, USA).
Statistics
All measurements for each condition were performed in triplicate. Quantitative data are represented as the mean ± standard deviation. The dose-response curve fit was determined using GraphPad Prism (ver. 6.0, GraphPad Software, La Jolla, CA). The statistical analysis was assessed using a two-sided Student’s t test. A p-value less than 0.05 was considered statistically significant.
Results
Radiosensitivity of cell lines
To determine the radiosensitivity of HeLa and CaSki cell lines by growth inhibition assay, radiation was applied to cells in 96-well plates at 20% to 30% confluency. At 36 hours later, HeLa cells revealed surviving fractions of 0.86 at 1 Gy, 0.57 at 2 Gy and 0.17 at 3 Gy, and CaSki cells revealed surviving fractions of 0.88 at 1 Gy, 0.74 at 2 Gy and 0.46 at 3 Gy (Supplementary Figure S1). The calculated 50% inhibitory concentration (IC50) of radiation was 2.2 Gy for HeLa and 2.8 Gy for CaSki (non-linear regression, Sigmoid-response model). Based on these results, we set a radiation dose of 2 Gy for an optimal condition to observe autophagy and apoptosis after irradiation.
Figure 1. (A) Western blot after a radiation dose of 2 Gy up to 36 hours in 12-hour intervals revealed the temporal increment of LC3 fraction of both cell lines. (B) Fluorescence microscope analysis identified radiation-induced intracellular autophagy (yellow arrows) (x 40). (C) Electron microscope analysis confirmed radiation-induced autophagy by visualisation of autophagic vesicles (arrows).
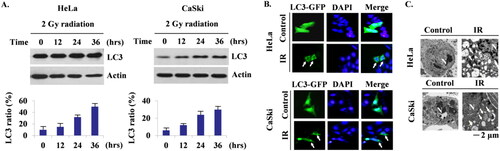
Radiation induces autophagy in cervical cancer cells
We confirmed radiation-induced autophagy by measuring the ratio of LC3 protein bands, the most widely used marker of the autophagosome, up to 36 hours in 12-hour intervals under a radiation dose of 2 Gy. A temporal increment of the LC3 ratio was revealed on the western blot of both cell lines after irradiation (). A discernible increase in the LC3 ratio was observed 24 hours after irradiation in both cell lines. Fluorescence microscope () and electron microscope () analyses visualised autophagy occurrence after radiation in both cell lines as well. By identifying autophagosomes on the fluorescence microscope and autophagic vesicles on the electron microscope, which is the golden standard to confirm autophagic activity, we clarified the intracellular process of autophagy in relevant cell lines.
Radiation-induced autophagy contributes to cell death, and it can be regulated by autophagic modulators
Compared to the LC3 ratio expressed in untreated radiated cells, 3-MA-treated cells showed a decreased LC3 ratio, and rapamycin-treated cells showed an increased LC3 ratio after radiation (). These findings demonstrate that radiation-induced autophagy is well-regulated by autophagic modulators in cervical cancer cells. Although the LC3 ratio of 3-MA-treated cells was lower than that of cells treated by radiation alone, it was still higher than that of the control (untreated, non-radiated cells). When cells were pre-treated with rapamycin, LC3 expression almost doubled after radiation.
Figure 2. (A, B) Compared to radiation treatment alone, prior treatment with 3-MA showed decreased autophagy after radiation, and prior treatment with rapamycin showed increased autophagy after radiation. (C) Cell viability assay results. When autophagy was inhibited with 3-MA, cytoprotective function of autophagy declined, resulting in more cell death than radiation alone, and when autophagy was augmented by rapamycin, even more cell death was induced, demonstrating an autophagic switch to cytotoxic form.
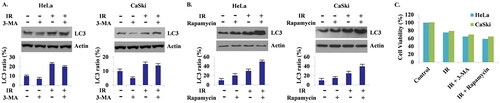
In addition, we analysed the cell viability of each preparation; radiation-only-treated cells, 3-MA pre-treated radiated cells and rapamycin pre-treated radiated cells. Compared to the control, cell viability decreased by 25% and 22% in HeLa and CaSki cells after radiation, respectively. When 3-MA treatment was preceded, viability decreased further by 35% and 31%, and even further by 41% and 36% when rapamycin treatment was performed before radiation ().
From these findings, altogether with the confirmation of radiation-induced autophagy, we can suggest that autophagy contributes to the process of cell death in radiated cervical cancer cells, and thus cell death, which in turn leads to radiosensitivity and can be regulated by autophagic modulators.
Apoptosis occurs later than and is induced by autophagy in radiation-treated cervical cancer cells
Western blotting to examine Caspase-3 and PARP protein bands of HeLa and CaSki cells after 2 Gy radiation indicated apoptosis occurrence 48 hour after radiation treatment, which was 24 hours later than autophagy occurrence (). Cleaved Caspase-3, which plays a central role in the execution phase of apoptosis, appeared 36 hours after radiation in HeLa and 24 hours after radiation in CaSki cells and became apparent 48 hours later. Cleaved PARP, a specific marker of late-stage apoptosis, was detected 48 hours after radiation.
Figure 3. Western blot to examine Caspase-3 and PARP of HeLa and CaSki cells after radiation of 2 Gy. (A) Apoptosis occurred 48 hours after radiation initiation, 24 hours later than autophagy. (B) When autophagy was inhibited by 3-MA, apoptosis decreased as well in both cell lines.
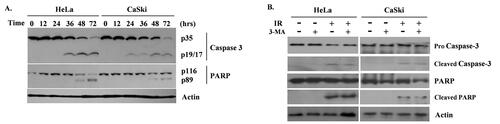
Since we determined that autophagy precedes apoptosis in the radiation of cervical cancer cells, next we investigated the effect of autophagy inhibition on apoptosis. HeLa and CaSki cells were treated with 3-MA and irradiated under 2 Gy. Cleaved Caspase-3 and cleaved PARP bands waned when 3-MA was added compared to untreated radiated cells ().
Taken together, we observed that autophagy occurs earlier and apoptosis occurs later in radiated cervical cancer cells, and that radiation-induced apoptosis is at least partly induced by autophagy.
Discussion
Cervical cancer is the fourth most common malignancy in women worldwide and the most common gynaecological malignancy affecting those younger than 45 (D'oria et al. Citation2022). Cervical cancer is mainly caused by a human papillomavirus infection, and host immunity, which consists of cervical immune cells and certain microbes, is thought to play an important role (Cascardi et al. Citation2022, Dellino et al. Citation2022). Radiation therapy is the main treatment option for locally advanced cervical cancer (Monk et al. Citation2022). However, about 30–50% of patients who receive radiation therapy experience treatment failure (Kumar and Gupta Citation2016), and the effectiveness of salvage treatment options is exceedingly poor (Yuh et al. Citation2009). Therefore, more research and efforts are needed to increase the effectiveness of radiation therapy in cervical cancer.
Autophagy has gained attention, especially in regard to cancer treatment, due to its innate function involved in cell survival and death. It is upregulated to maintain cellular stability against tumorigenesis and, paradoxically, anticancer therapy. When radiation is applied, the cytoprotective effect of tumour cells is aroused, and autophagy inhibition is suggested to be therapeutically advantageous (Wang et al. Citation2014, Tam et al. Citation2017). Studies have proven that pharmacologic autophagy inhibition increases radiosensitivity in breast, cervical, colorectal, nasopharyngeal, oesophageal and lung cancer cell lines (Xin et al. Citation2017). On the contrary, induction of autophagy has been proposed to improve radiosensitivity by promoting type II autophagic cell death (Chang et al. Citation2014, Ondrej et al. Citation2016). A recent study verified enhanced radiosensitivity of glioma by autophagy induction with borneol via inhibition of the mammalian target of rapamycin complex 1/eukaryotic initiation factor 4E (mTORC1/eIF4E) pathway (Li et al. Citation2021).
We designed this study to determine radiation-induced autophagy, its contribution to cell death and the feasibility of regulation of radiosensitivity by autophagic modulators in cervical cancer cells. The temporal increment of radiation-induced autophagy was confirmed by increased LC3 fractions on western blot, LC3-GFP on a fluorescence microscope and decisively by visualisation of autophagic vesicles on an electron microscope. This radiation-induced autophagy turned out to respond to autophagy manipulation in cervical cancer cell lines. Cell viability decreased further with autophagy inhibition by 3-MA treatment prior to radiation, and even more, increased cell death was observed with autophagy induction by rapamycin treatment, raising the possibility of an “autophagic switch” to a cytotoxic (i.e. tumour-suppressing) effect (Gewirtz Citation2014a, Gewirtz Citation2014b).
The concept of an autophagic switch is derived from the complexity of autophagy, in which autophagy can switch its function within the same cellular reaction process to certain external stress (Baskar et al. Citation2012). Wilson et al. (Citation2011) demonstrated the cytoprotective effect of the autophagy of breast tumour cells when induced by radiation alone, which was supported by the fact that radiosensitivity increased when autophagy was inhibited, and it could change its function into cytotoxic form when radiation was delivered in combination with vitamin D. Likewise, in our experiment, we identified cytoprotective autophagy first with radiation alone, thereby enhancing radiosensitivity when autophagy was inhibited. Then, we switched to cytotoxic autophagy with rapamycin combination, intensifying the radiosensitivity even further (Supplementary Figure S2).
Moreover, we investigated the sequential relation of autophagy with apoptosis in radiated cervical cancer cells. Gozuacik and Kimchi (Citation2004) proposed three types of autophagy-apoptosis interconnection in the programmed cell death context: first, circumstances where autophagy is indispensable and preceding apoptosis, which may even be a key to turning on apoptosis; second, when autophagy has an antagonising effect on apoptosis; and last, when two mechanisms occur independently of each other. From our study, we identified that apoptosis is preceded by autophagy by about 24 hours and that it is at least partly dependent on autophagy, supporting the first model. Although the specific pathways remain obscure, autophagy may degrade mitochondria during radiation, leading to intrinsic apoptosis (Surova and Zhivotovsky Citation2013).
Through this study, we newly demonstrated radiation-induced autophagy in cervical cancer cells, which is switched from cytoprotective form to cytotoxic form with autophagic augmentation. When cytoprotective autophagy is present with radiation alone, the therapeutic outcome can be improved by inhibiting autophagy. Cytotoxic autophagy, on the other hand, was realised with autophagic induction in combination with radiation in relevant cell lines. Our results suggest that autophagic modifiers may have a potential role in the treatment of cervical cancer. However, our study is limited by the fact that autophagic modulators do not exclusively alter autophagy but are involved in other signalling pathways in the tumour microenvironment. This can lead to heterogeneity in the tumour response to autophagic modulators (Roy et al. Citation2022). Further preclinical studies are needed to evaluate the combination therapy of autophagy modulators and radiotherapy in cervical cancer.
In conclusion, autophagic modulators can regulate radiation-induced autophagy, which contributes to cell death and induces apoptosis in cervical cancer cell lines.
Supplemental Material
Download MS Word (819.9 KB)Disclosure statement
The authors declare that there are no conflicts of interest or financial ties to disclose regarding this article.
Data availability statement
The data that support the findings of this study are available from the corresponding author, [KHL], upon reasonable request.
Additional information
Funding
References
- Baskar, R., et al., 2012. Cancer and radiation therapy: current advances and future directions. International Journal of Medical Sciences, 9 (3), 193–199.
- Bogani, G., et al., 2022. Development of a nomogram predicting the risk of persistence/recurrence of cervical dysplasia. Vaccines, 10 (4), 579.
- Briceno, E., Reyes, S. and Sotelo, J., 2003. Therapy of glioblastoma multiforme improved by the antimutagenic chloroquine. Neurosurgical focus, 14 (2), e3.
- Cascardi, E., et al., 2022. Association between cervical microbiota and HPV: could this be the key to complete cervical cancer eradication? Biology, 11 (8), 1114.
- Chang, L., et al., 2014. PI3K/Akt/mTOR pathway inhibitors enhance radiosensitivity in radioresistant prostate cancer cells through inducing apoptosis, reducing autophagy, suppressing NHEJ and HR repair pathways. Cell death & Disease, 5 (10), e1437.
- Dellino, M., et al., 2022. Lactobacillus crispatus M247 oral administration: is it really an effective strategy in the management of papillomavirus-infected women? Infectious Agents and Cancer, 17 (1), 53.
- D'oria, O., et al., 2022. New advances in cervical cancer: from bench to bedside. International Journal of Environmental Research and Public Health, 19 (12), 7094.
- Gewirtz, D.A., 2014a. An autophagic switch in the response of tumor cells to radiation and chemotherapy. Biochemical pharmacology, 90 (3), 208–211.
- Gewirtz, D.A., 2014b. The four faces of autophagy: implications for cancer therapy. Cancer Research, 74 (3), 647–651.
- Giannini, A., et al., 2022. Advances on prevention and screening of gynecologic tumors: Are We Stepping Forward? Healthcare, 10 (9), 1605.
- Gozuacik, D. and Kimchi, A., 2004. Autophagy as a cell death and tumor suppressor mechanism. Oncogene, 23 (16), 2891–2906.
- Jackson, S.P. and Bartek, J., 2009. The DNA-damage response in human biology and disease. Nature, 461 (7267), 1071–1078.
- Kumar, L. and Gupta, S., 2016. Integrating chemotherapy in the management of cervical cancer: a critical appraisal. Oncology, 91 (Suppl 1), 8–17.
- Levine, B. and Kroemer, G., 2008. Autophagy in the pathogenesis of disease. Cell, 132 (1), 27–42.
- Levy, J.M.M., Towers, C.G. and Thorburn, A., 2017. Targeting autophagy in cancer. Nature Reviews Cancer, 17 (9), 528–542.
- Li, Q., et al., 2021. Role of borneol-induced autophagy in enhancing radiosensitivity of malignant Glioma. Frontiers in Oncology, 11, 749987.
- Monk, B.J., et al., 2022. Proportions and incidence of locally advanced cervical cancer: a global systematic literature review. International Journal of Gynecological Cancer : Official Journal of the International Gynecological Cancer society, 32 (12), 1531–1539.
- Ondrej, M., et al., 2016. To live or let die: unclear task of autophagy in the radiosensitization battle. Radiotherapy and Oncology: Journal of the European Society for Therapeutic Radiology and Oncology, 119 (2), 265–275.
- Rabinowitz, J.D. and White, E., 2010. Autophagy and metabolism. Science, 330 (6009), 1344–1348.
- Roy, A., et al., 2022. Role of autophagy in tumor response to radiation: Implications for improving radiotherapy. Frontiers in Oncology, 12, 957373.
- Surova, O. and Zhivotovsky, B., 2013. Various modes of cell death induced by DNA damage. Oncogene, 32 (33), 3789–3797.
- Tam, S.Y., Wu, V.W. and Law, H.K., 2017. Influence of autophagy on the efficacy of radiotherapy. Radiation Oncology, 12 (1), 57.
- Wang, Y., Yin, W. and Zhu, X., 2014. Blocked autophagy enhances radiosensitivity of nasopharyngeal carcinoma cell line CNE-2 in vitro. Acta oto-Laryngologica, 134 (1), 105–110.
- White, E., 2012. Deconvoluting the context-dependent role for autophagy in cancer. Nature reviews. Cancer, 12 (6), 401–410.
- Wilson, E.N., et al., 2011. A switch between cytoprotective and cytotoxic autophagy in the radiosensitization of breast tumor cells by chloroquine and vitamin D. Hormones & Cancer, 2 (5), 272–285.
- Xin, Y., et al., 2017. Role of autophagy in regulating the radiosensitivity of tumor cells. Journal of Cancer Research and Clinical Oncology, 143 (11), 2147–2157.
- Yuh, W.T., et al., 2009. Predicting control of primary tumor and survival by DCE MRI during early therapy in cervical cancer. Investigative Radiology, 44 (6), 343–350.