ABSTRACT
In this paper, the effect of temperature dependent internal source on the onset of convection and on the heat transfer in a porous layer saturated by a non-Newtonian nanofluid is examined. The Oldroyd-B type model incorporates the effects of Brownian motion and thermophoresis is used for the non-Newtonian nanofluid, while Darcy model is employed for porous medium. The flux of volumetric fraction of nanoparticles is considered to be zero on the boundaries. Linear and nonlinear stability analyses are used to examine onset and heat transfer in the system. The linear stability analysis is studied with the help of normal mode technique, and the nonlinear stability analysis is made using truncated representation of Fourier series. The effects of the internal heat source parameter, the stress relaxation parameter, the strain retardation parameter, the heat capacity ratio, and the nanoparticle parameters on the onset of convection and on the heat transfer are obtained and depicted graphically. It is observed that the effects of increasing internal heat source parameter, the stress relaxation parameter, and the nanoparticles parameters have a destabilizing effect on the stability of the system and also increase the heat transfer across the porous layer, whereas an opposite trend is noticed with increasing the strain retardation parameter and the heat capacity ratio.
Nomenclature
a | = | dimensionless wave number |
ac | = | critical wave number |
d | = | thickness of porous layer (m) |
DB | = | Brownian diffusion coefficient (m2/s) |
DT | = | thermophoresis diffusion coefficient (m2/s) |
= | acceleration due to gravity (m/s2) | |
Hs | = | internal heat source parameter |
km | = | effective thermal conductivity (W/(m.K)) |
Le | = | Lewis number |
NA | = | modified diffusivity ratio |
NB | = | modified particle-density increment |
p | = | pressure (Pa) |
(ρc) | = | heat capacity (J/K) |
= | Darcy velocity (m/s) | |
RD | = | Darcy-Rayleigh number |
Rn | = | nanoparticle Darcy-Rayleigh number |
t* | = | time (s) |
T* | = | temperature (K) |
(x*, y*, z*) | = | space co-ordinates (m) |
Greek symbols
α | = | thermal diffusivity (m2/s) |
βT | = | coefficient of thermal expansion (K−1) |
μ | = | viscosity (Pa.s) |
λ1 | = | stress relaxation parameter |
λ2 | = | strain retardation parameter |
σ | = | heat capacity ratio |
ρ | = | density of the non-Newtonian nanofluid (kg/m3) |
ρ0 | = | reference density of non-Newtonian nanofluid (kg/m3) |
ρp | = | density of nanoparticles (kg/m3) |
φ | = | volume fraction of the nanoparticles |
φ0* | = | reference scale for the nanoparticle fraction |
∇2P | = | horizontal Laplacian operator |
∇2 | = | Laplacian operator |
Subscripts
p | = | particle |
b | = | basic state |
c | = | critical value |
0 | = | lower boundary |
1 | = | upper boundary |
Superscripts
* | = | dimensional variables |
′ | = | perturbed quantities |
Additional information
Notes on contributors
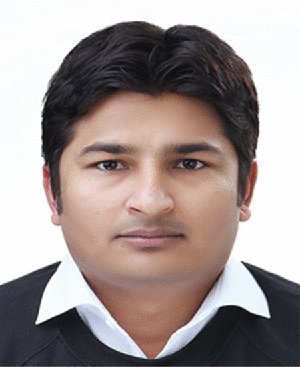
Dhananjay Yadav
Dhananjay Yadav is currently working as an Assistant Professor in Department of Mathematical and Physical Sciences, University of Nizwa, Oman. Prior to that, he had worked as Senior Post Doctorate Fellow at Athabasca University, Canada and Yonsei University, South Korea. He received his Ph.D. degree from Department of Mathematics, Indian Institute of Technology (IIT) Roorkee, India in 2013 and post-graduation (M.Sc.) in Mathematics from DDU University Gorakhpur, India in 2007. His expertise is in CO2 capture, storage and oil recovery, computational sustainability and environmental analytics, fluid mechanics, numerical analysis, hydrodynamic and hydromagnetic stability, transient stability, nanofluids and fluid flow in porous media. He has published over 50 reviewed research papers and book chapters.
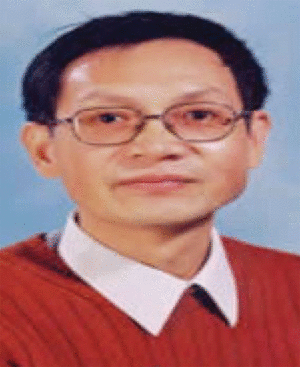
Junye Wang
Junye Wang is a Professor and CAIP Research Chair of Computational Sustainability and Environmental Analytics at the Athabasca River Basin Research Institute, Athabasca University, Canada. He holds a Ph.D. from the East China University of Science and Technology, and has worked on environment, energy and sustainability for over 35 years. He joined ARBRI as a CAIP Research Chair in Computational Sustainability and Environmental Analytics in September, 2013. Prior to that, he had served as Principal Research Scientist at Rothamsted Research in the UK, the longest running agricultural research station in the world. He is a leading expert in modeling and simulation of complex environmental and energy processes and systems, such as ecosystems, nutrient cycling and carbon sequestration, groundwater, pollutants, greenhouse gas emission and mitigation, fuel cells, microbial fuel cells, biogas, bioenergy, and biomass. He and his co-workers have published over 70 reviewed research papers and book chapters.