ABSTRACT
To investigate the association between dietary acrylamide and advanced prostate cancer, we examined acrylamide-gene interactions for advanced prostate cancer risk by using data from the Netherlands Cohort Study.
Participants (n = 58,279 men) completed a baseline food frequency questionnaire (FFQ), from which daily acrylamide intake was calculated. At baseline, 2,411 men were randomly selected from the full cohort for case-cohort analysis. Fifty eight selected single nucleotide polymorphisms (SNPs) and two gene deletions in genes in acrylamide metabolism, DNA repair, sex steroid systems, and oxidative stress were analyzed. After 20.3 years of follow-up, 1,608 male subcohort members and 948 advanced prostate cancer cases were available for Cox analysis.
Three SNPs showed a main association with advanced prostate cancer risk after multiple testing correction: catalase (CAT) rs511895, prostaglandin-endoperoxide synthase 2 (PTGS2) rs5275, and xeroderma pigmentosum group C (XPC) rs2228001. With respect to acrylamide-gene interactions, only rs1800566 in NAD(P)H quinone dehydrogenase 1 (NQO1) and rs2301241 in thioredoxin (TXN) showed a nominally statistically significant multiplicative interaction with acrylamide intake for advanced prostate cancer risk. After multiple testing corrections, none were statistically significant.
In conclusion, no clear evidence was found for interaction between acrylamide intake and selected genetic variants for advanced prostate cancer risk.
Introduction
Prostate cancer is a hormone-related cancer that is responsive to androgen deprivation (hormonal) therapy (Citation1). In the western world, prostate cancer has one of the highest incidence rates of all cancers in men, with approximately 759,000 new cases in 2012 (Citation2). Age, family history of prostate cancer, and black race are accepted risk factors, but other risk factors have not been convincingly established (Citation3). Incidence rates (both overall and age-specific) vary widely between countries, which can partly be explained by the increase of prostate-specific-antigen (PSA) testing in developed countries (Citation4). Environmental factors may also contribute to these differences. For example, migrant studies have shown that prostate cancer incidence rates increased among men who migrated to a region with higher prostate cancer incidence (Citation5). Of course, increased access of migrants to PSA testing may contribute to this rise in incidence, but dietary factors are also believed to influence the incidence of prostate cancer (Citation6). However, to date, there is still little known about a possible association between diet and prostate cancer (Citation3).
Since its discovery in food in 2002, dietary acrylamide has been the subject of numerous epidemiologic studies on cancer. Acrylamide arises as a by-product of the Maillard reaction between the amino acid asparagine and reducing sugars (e.g., fructose, sucrose), during high-temperature cooking of foods such as cookies, potato chips, and French fries. The International Agency for Research on Cancer (IARC) classified acrylamide as a probable human carcinogen, based on evidence derived from rodent studies. Epidemiologic studies in humans, thus far, have reported inconsistent findings on cancer risk, with some studies showing increased risk for hormone-related cancers (endometrial and ovarian cancer) (Citation7). In a previous study by our group (Citation8), high intake of acrylamide was non-significantly inversely associated with advanced prostate cancer among never-smokers after 13.3 years of follow-up. The analysis was restricted to never-smokers to exclude any possible confounding effect of smoking, which is a major source of acrylamide. While another cohort study (Citation9) also found acrylamide intake to be non-significantly inversely associated with advanced prostate cancer risk in never-smokers, the third other cohort study (Citation10) did not show any associations with advanced prostate cancer. It thus remains unclear whether acrylamide intake influences advanced prostate cancer risk.
A number of mechanisms may explain the effect of acrylamide on cancer risk (Citation11). The first mechanism involves glycidamide, an epoxide metabolite of acrylamide. Glycidamide forms DNA adducts and is therefore thought to be the carcinogenic compound in acrylamide-induced carcinogenesis due to its genotoxicity (Citation12). Second, in previous analyses by our group (Citation8, Citation13), findings with hormone-related cancers support the hypothesis of a hormonal mechanism of acrylamide. A third mechanism is acrylamide-induced oxidative stress. Oxidative stress occurs when reactive oxygen species (ROS), generated by pro-oxidants, outbalance the antioxidant system (Citation14). This imbalance becomes more common with increasing age (Citation15) and may therefore play an important role in the development and progression of age-related cancers including prostate cancer (Citation16). However, it is unclear whether and how these mechanisms may provide a causal explanation for the association between acrylamide and prostate cancer.
Therefore, in the current study, we used data from the Netherlands Cohort Study on diet and cancer and explored whether genetic variation modifies the association between dietary acrylamide and advanced prostate cancer risk. For that matter, 60 single nucleotide polymorphisms (SNPs) and two gene deletions in genes involved in acrylamide metabolism and the hypothesized mechanisms of acrylamide-induced carcinogenesis (DNA repair, a sex hormonal effect and oxidative stress) were selected. We examined the association between selected genetic variants and advanced prostate cancer risk and investigated acrylamide-gene interactions.
Methods
Study Population and Design
The prospective Netherlands Cohort Study (NLCS) on diet and cancer included 58,279 men aged 55–69 years. At baseline (1986), participants completed a one-time self-administered questionnaire on dietary habits, lifestyle, and other risk factors for cancer. Participants provided informed consent for study participation by completing and returning this questionnaire. About 75% of the participants provided toenail clippings for DNA-analyses. For reasons of efficiency, a case-cohort approach was used (Citation17). To this end, a subcohort, including 2,411 men, was randomly selected from the full cohort immediately after baseline. Subcohort members were then followed up for migration and vital status, to accurately estimate the accumulated person years of the full cohort. Advanced prostate cancer cases were derived from the full cohort and identified by regular record linkage to the Netherlands Cancer Registry and the Dutch Pathology Registry (PALGA) (Citation18). Further details on the study can be found elsewhere (Citation19). The NLCS has been approved by the institutional review boards of the University Hospital Maastricht and TNO Nutrition and Food Research.
Cases were classified by the Netherlands Cancer Registry (NCR) according to the International Union Against Cancer tumor-node metastasis classification (TNM) staging system (Citation20). We included prostate cancers with a pathologic or clinical TNM staging score of T3/T4, N+, or M1 at diagnosis. Prevalent cancer cases (other than skin cancer) at baseline were excluded from analysis. Furthermore, cases and subcohort members were excluded if dietary data were either incomplete or inconsistent, toenail clippings were not provided or genotyping was unsuccessful (sample call rate < 95%). With respect to acrylamide-gene interaction analysis, cases and subcohort members were additionally excluded if they had missing data on covariables. After 20.3 years of follow-up, 1,608 subcohort members and 948 incident cases of advanced prostate cancer were available for analysis. shows a flow diagram of the exclusion criteria applied to cases and subcohort members.
Figure 1. Flow diagram of subcohort members and advanced prostate cancer cases for 20.3 years of follow-up; Netherlands Cohort Study on diet and cancer (1986–2006). (a) Analysis on the association between selected genetic variants and advanced prostate cancer risk including 1,720 subcohort members and 1,000 advanced prostate cancer cases. (b) Analysis on the interaction between selected genetic variants and acrylamide intake on advanced prostate cancer risk including 1,608 subcohort members and 948 advanced prostate cancer cases.
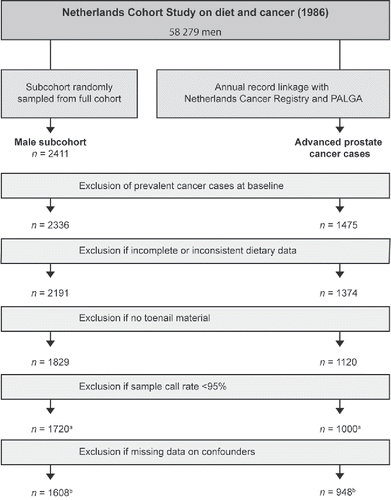
Assessment of Acrylamide Intake
The baseline questionnaire included a 150-item food frequency questionnaire (FFQ) to estimate daily food and nutrient intake, which has been tested for validity and reproducibility (Citation21,Citation22). As described in detail elsewhere (Citation13), we used data on acrylamide levels in foods on the Dutch market. Daily acrylamide intake was estimated by multiplying frequency of consumption by portion size and the mean acrylamide content of each acrylamide-containing food.
A 24-hour duplicate diet study by our group indicated that subjects can be reliably ranked with respect to acrylamide intake using mean acrylamide values for individual foods (Citation23). The Spearman's correlation between the calculated and (chemically) measured acrylamide intake was 0.82 (P < 0.001).
Gene and SNP Selection
A detailed description of the gene and SNP selection has been provided elsewhere (Citation24). Briefly, we selected SNPs in genes involved in acrylamide metabolism and the hypothesized mechanisms of acrylamide-induced carcinogenesis: genotoxicity (selected SNPs in DNA repair genes), a sex hormonal effect and oxidative stress, and that were shown to be associated with a sex hormone-related cancer (endometrial, ovarian, breast, or prostate cancer). In addition, we selected SNPs that, to our knowledge, have not been evaluated for their association with hormone-related cancers, but were shown to be of significance in acrylamide-related polymorphism- or gene expression studies.
Only validated SNPs with a minor allele frequency of ≥10% in Caucasians in dbSNP were selected.
The SNPs (n = 60) we selected were in the following genes: aldo-keto reductase family 1, member C1 (AKR1C1), aldo-keto reductase family 1, member C2 (AKR1C2), catalase (CAT), catechol-O-methyltransferase (COMT), cytochrome P450 family 1 subfamily A member 1 (CYP1A1), cytochrome P450 family 1 subfamily A member 2 (CYP1A2), cytochrome P450 family 1 subfamily B member 1 (CYP1B1), cytochrome P450 family 11 subfamily A member 1 (CYP11A1), cytochrome P450 family 17 subfamily A member 1 (CYP17A1), cytochrome P450 family 19 subfamily A member 1 (CYP19A1), cytochrome P450 2E1 (CYP2E1), epoxide hydrolase 1 (EPHX1), estrogen receptor 1 (ESR1), estrogen receptor 2 (ESR2), glutathione peroxidase 1 (GPX1), glutathione S-transferase alpha 5 (GSTA5), glutathione S-transferase P1 (GSTP1), hydroxysteroid (17-beta) dehydrogenase 3 (HSD17B3), 3beta-hydroxysteroid dehydrogenase (HSD3B1/B2), MGC12965, mutY DNA glycosylase (MUTYH), nuclear factor kappa B subunit 1 (NFKB1), nitric oxide synthase 2 (NOS2), NAD(P)H quinone dehydrogenase 1 (NQO1), 8-oxoguanine DNA glycosylase 1 (OGG1), progesterone receptor (PGR), prostaglandin-endoperoxide synthase 2 (PTGS2), ribonucleotide reductase regulatory subunit M2 (RRM2), sex hormone binding globulin (SHBG), solute carrier family 7 (cationic amino acid transporter, y+ system), member 11 (SLC7A11), superoxide dismutase 1 (SOD1), superoxide dismutase 2 (SOD2), steroid 5 alpha-reductase 1 (SRD5A1), sulfotransferase family 1A member 1 (SULT1A1), sulfotransferase family 1E member 1 (SULT1E1), thioredoxin (TXN), UDP glucuronosyltransferase family 1 member A6-10 (UGT1A6-10), xeroderma pigmentosum, complementation group C (XPC), and x-ray repair complementing defective repair in Chinese hamster cells 1 (XRCC1).
In addition, glutathione s-transferase mu 1 (GSTM1) and glutathione s-transferase theta 1 (GSTT1) were selected as genes involved in acrylamide metabolism (Citation25). Since the beginning and ending of the GSTM1 and GSTT1 deletions are not exactly known, it was impossible to design one SNP assay (based on single base extension) for the deletion. Therefore, we chose three SNPs for GSTM1 (rs10857795, rs200184852, and rs74837985) and four SNPs for GSTT1 (rs2844008, rs4630, rs140309, and rs8140585) to represent the presence or absence of the gene. In case all SNPs within a gene were not called, we interpreted this as a deletion of the gene.
Finally, 67 SNPs (60 SNPs, plus 7 SNPs to represent the GST deletions) were genotyped using two multiplex panels. Supplementary Table S1 provides an overview of the genotyped SNPs (not including the seven SNPs representing the GST deletions).
DNA Isolation and Genotyping
DNA was isolated from 15 mg of toenail clippings, according to a protocol described in detail elsewhere (Citation26). SNP genotyping was done on the MassARRAY system in conjunction with the iPLEX TM assay (Citation27).
The reproducibility of genotyping for the analyzed SNPs (minus 7 SNPs representing the GST deletions) was assessed from 146 duplicate samples, which was >99% (excluding missing values). Out of 60 SNPs, two SNPs (rs3736599 and rs7741) were excluded from analyses due to insufficient genotyping success (call rate < 80%); the assay for rs3736599 failed completely (0% call rate). After correction for multiple testing, using the Benjamini–Hochberg (1995) false discovery rate (FDR) approach (Citation28), two SNPs (rs1001179 and rs5746136) were not in Hardy–Weinberg equilibrium (FDR-adjusted P value <0.20) (see Supplementary Table S1).
A total of 229 samples (120 cancer cases, 109 subcohort members) were excluded due to a sample call rate below 95%. With respect to the three selected SNPs to represent the GSTM1 deletion, rs10857795 was not called in 39%, rs200184852 in 44%, and rs74837985 in 2% of the subcohort. The GSTM1 gene is deleted in approximately 40–50% of the Caucasians. This probably indicates that the low proportion of missings for rs74837985 was due to genotyping error, possibly caused by unspecific amplification. Therefore, only rs10857795 and rs200184852 were selected to represent the GSTM1 deletion. With respect to the four SNPs representing the GSTT1 deletion, it was found that rs2844008 was not called in 64%, rs4630 in 15%, rs140309 in 11%, and rs8140585 in 85% of the subcohort. The GSTT1 gene is deleted in about 20% of the Caucasians, thus rs2844008 and rs814058 were probably not correctly genotyped and therefore not statistically analyzed in isolation.
Statistical Analysis
A Cox proportional hazards model was used to calculate hazard ratios (HRs) with 95% confidence intervals (CIs). Robust standard errors were calculated to account for the additional variance introduced by sampling a subcohort from the full cohort (Citation29). Follow-up time (time-on-study) was used as the time scale and defined as time from baseline (Sept. 1986) to either diagnosis of advanced prostate cancer, death, emigration or loss to follow-up, whichever came first. The proportional hazards (PH) assumption was assessed by using the scaled Schoenfeld residuals (Citation30).
In models that examined the main effect of dietary acrylamide and acrylamide-gene interactions, age, family history of prostate cancer, and smoking were included as predefined covariables. Internal acrylamide exposure and smoking are strongly associated, because smoking is an important source of acrylamide. For smoking to be a confounder, it must be associated with advanced prostate cancer risk as well. The evidence for this association is mixed (Citation31,Citation32) and unpublished results by our group did not reveal an association between smoking and advanced prostate cancer risk. However, to minimize any residual confounding, we decided to adjust for smoking. In this perspective, we also analyzed never-smokers (main effect dietary acrylamide only) and non-smokers (never smokers combined with former smokers who had quit smoking more than 10 years before baseline). Preferably, we would have analyzed the never-smokers group for acrylamide-gene interactions (given the previously reported inverse association; ref. Citation8), but this was impossible due to the insufficient number of cases and subcohort members in this group. Therefore, we chose to analyze non-smokers and also adjusted for (former) smoking within that group. The smoking variables that were entered into the models were: cigarette smoking status (never/former/current), frequency of cigarettes smoked per day, and duration of smoking (years).
Based on literature, the following variables were a priori considered as potential confounders and only included in the model if they changed the acrylamide hazard ratio by >10%: BMI (kg/m2), non-occupational physical activity (min/day), level of education (four categories), positive history of diabetes (yes/no), total energy intake (kcal/day), fruit (g/day), vegetables (g/day), dairy products (g/day), lycopene (μg/day), calcium (mg/day), and vitamin E (μg/day). None of the variables changed the acrylamide hazard ratio by >10% and were thus not included in the final model. Throughout the analyses, we adjusted for age, family history of prostate cancer, and smoking, except for the association between SNPs and advanced prostate cancer risk (age-adjusted only).
As a first analysis, we examined whether the inverse association between dietary acrylamide and advanced prostate cancer risk in never-smokers after 13.3 years of follow-up persisted after 20.3 years of follow-up.
The association between variants in CAT (rs1001179), GPX1 (rs3448), NQO1 (rs1800566), OGG1 (rs1052133), SOD1 (rs10432782), and SOD2 (rs4880) and advanced prostate cancer risk were previously reported by our group (Citation33,Citation34). For that reason, the main effects of these SNPs on advanced prostate cancer risk will not be presented in the current study.
Multiplicative interaction P values for the interaction between acrylamide and genotypes (assuming a dominant genetic model) were tested using product terms between acrylamide intake (continuous) and genotype. Dose-response across genotype strata was tested by using the median acrylamide intake of each quartile as a continuous variable. In sensitivity analysis, we repeated the acrylamide-gene interaction analysis in non-smokers for the 13.3 year follow-up period. P trends (main effect SNPs only) and acrylamide-gene interaction P values were corrected for multiple testing, using the Benjamini–Hochberg (1995) FDR approach (Citation28). The FDR threshold for these analyses was set at 0.20, which is common in candidate gene studies (Citation33). FDR-adjusted P values were separately calculated for the total study population and for non-smokers.
All statistical analyses were performed with STATA (version 13.1, StataCorp LP, College Station, TX, USA) and reported P values were two-sided, with P < 0.05 considered nominally statistically significant.
Results
At baseline, cases were comparable to subcohort members regarding acrylamide intake, age, BMI, education, cigarette smoking status, and diet (). In the subcohort, former smokers with more than 10 years of cessation had quit smoking for a mean (SD) of 20.8 (7.0) years, which was comparable to that of cases. As compared to subcohort members, cases more often had a family history of prostate cancer but less often a history of diabetes. Subcohort members (and cases) that provided toenail clipping were comparable to subcohort members (and cases) that did not provide toenail clippings, except for level of education and cigarette smoking status (data not shown).
Table 1. Baseline characteristics of subcohort members and advanced prostate cancer cases in the Netherlands Cohort Study on diet and cancer (1986–2006)Footnote1.
Main Effect of Acrylamide Intake
After 20.3 years of follow-up no associations were found between acrylamide intake and advanced prostate cancer risk in the total study population [HR(Q5 vs. Q1) = 1.03, 95% CI: 0.82–1.29; P trend = 0.89], never-smokers [HR(Q5 vs. Q1) = 0.90, 95% CI: 0.51–1.60; P trend = 0.68] and non-smokers [HR(Q5 vs. Q1) = 0.93, 95% CI: 0.68–1.26; P trend = 0.78] (). Also, analysis with 13.3 years of follow-up did not reveal any association apart from the previously reported (Citation8) non-statistically significant inverse dose response relationship in never-smokers ().
Table 2. Association between dietary acrylamide intake and advanced prostate cancer risk after 13.3 and 20.3 years of follow-up; Netherlands Cohort Study on diet and cancer (1986).
Main Effect of SNPs
Six SNPs showed a nominally statistically significant association with advanced prostate cancer risk after 20.3 years of follow-up (). Men with variant alleles of rs11252887 (AKR1C2), rs511895 (CAT), rs10923823 (HSD3B1/B2), and rs7546652 (HSD3B1/B2) showed an increase in risk for advanced prostate cancer, with HRs per minor allele of 1.16 (95% CI: (1.03–1.32); P trend = 0.02), 1.17 [95% CI: (1.05–1.31); P trend = 0.01], 1.14 [95% CI: (1.01–1.27); P trend = 0.03] and 1.14 [95% CI: (1.02–1.28); P trend = 0.03], respectively. A decreased risk of advanced prostate cancer was observed for men with variant alleles of rs5275 (PTGS2) and rs2228001 (XPC), with HRs per minor allele of 0.85 [95% CI: (0.76–0.96); P trend = 0.01] and 0.83 [95% CI: (0.74–0.94); P trend = 0.002], respectively. After multiple testing correction, rs5118895 in CAT (FDR-adjusted P value = 0.15), rs5275 in PTGS2 (FDR-adjusted P value = 0.19) and rs2228001 in XPC (FDR-adjusted P value = 0.11), remained significant at level 0.20. For the other SNPs and 2 gene deletions, we did not observe clear associations with advanced prostate cancer risk (data not shown).
Table 3. SNPs showing nominally statistically significant association (P trend < 0.05) with advanced prostate cancer risk after 20.3 years of follow-up; Netherlands Cohort Study on diet and cancer (1986–2006).
Interactions between Acrylamide Intake and SNPs
Out of 58 analyzed SNPs and 2 gene deletions, two SNPs showed a nominally statistically significant multiplicative interaction with acrylamide intake in the total study population (); rs1800566 (NQO1) with a P interaction of 0.03 and rs2301241 (TXN) with a P interaction of 0.04. Neither remained significant at level 0.20 after adjusting for multiple comparisons and we did not observe a clear dose-response relationship for acrylamide in strata of those genotypes. In non-smokers, no SNPs showed evidence of multiplicative interaction with acrylamide intake. A detailed overview of the acrylamide-gene interactions is provided in Supplementary Table S2.
Table 4. SNPs showing nominally statistically significant interaction (P for interaction < 0.05) with dietary acrylamide on advanced prostate cancer risk after 20.3 years of follow-up; Netherlands Cohort Study on diet and cancer (1986–2006).
In sensitivity analyses, we analyzed the acrylamide-gene interactions in non-smokers for 13.3 years of follow-up. Two SNPs (rs11252859 in AKR1C1 and rs8192120 in SRD5A1) showed a nominally statistically significant multiplicative interaction with acrylamide intake, but they did not withstand correction for multiple testing (data not shown).
Discussion
In this large population-based prospective cohort study, we analyzed acrylamide-gene interactions for advanced prostate cancer risk, which has not been done before. Six SNPs were associated with advanced prostate cancer risk, three of which remained significant after multiple comparisons correction (rs511895 in CAT, rs5275 in PTGS2, and rs2228001 in XPC). Two SNPs (rs1800566 in NQO1 and rs2301241 in TXN) showed a nominally statistically significant multiplicative interaction with acrylamide intake, but neither remained significant after adjusting for multiple comparisons.
CAT is an antioxidant enzyme that plays a key role in oxidative stress protection by degrading hydrogen peroxide (Citation35). The intronic CAT rs511895 SNP showed no association with lethal prostate cancer in the Health Professionals Follow-up Study (HPFS) (Citation36). In the same study, this SNP was associated with circulating levels of alpha-tocopherol (Vitamin E), an antioxidant that may reduce prostate cancer risk. This may indicate that CAT rs511895 is a functional SNP or in linkage disequilibrium with another functional SNP. In the present study, participants with one or two variant alleles of CAT rs511895 had a higher advanced prostate cancer risk than homozygous wild type participants. In a previous study by our group (Citation34), another CAT SNP (rs1001179) was also associated with increased advanced prostate cancer risk. As discussed by the authors, this association could be explained by reduced catalase activity and, consequently, deficiency in antioxidant protection against oxidative stress. We cannot provide such an explanation for CAT rs511895, which may indicate that our finding is due to chance (even after correction for multiple testing). PTGS2 encodes COX-2, an enzyme that converts arachidonic acid to prostaglandin H2 (Citation37). COX-2 promotes inflammation and is overexpressed in various cancers, including prostate cancer (Citation38). According to a meta-analysis (Citation39), PTGS2 rs5275 was not associated with prostate cancer risk in Caucasians, but in that study prostate cancer subtypes were not examined. In our study, the rare allele of PTGS2 rs5275 was associated with a decreased advanced prostate cancer risk. PTGS2 rs5275 is located in the 3′-untranslated region of the PTGS2 gene and thought to regulate mRNA stability and degradation (Citation40), thereby possibly influencing prostate cancer carcinogenesis. However, the PTGS2 rs5275 SNP is not clearly associated with PTGS2 gene expression in lymphoblastoid cel lines (Citation41). XPC is a protein (encoded by the XPC gene) that plays an important role in DNA damage recognition, in the global genome nucleotide excision repair (GG-NER) pathway (Citation42). A meta-analysis found that the non-synonymous coding XPC rs2228001 SNP was not associated with prostate cancer risk in Caucasians (Citation43). However, only two studies were included (including one study with a small sample size) and prostate cancer subtypes were not examined. In our study, we show that the rare allele of XPC rs2228001 was associated with a decreased advanced prostate cancer risk. Various studies, however, have shown that this polymorphism was associated with increased cancer risk through decreased DNA repair capacity (Citation44). This indicates that the inverse association we found lacks biological plausibility, and may therefore represent a chance finding. With regard to all three SNPs (rs511895 in CAT, rs5275 in PTGS2, and rs2228001 in XPC), future well-designed gene-association studies with large sample size are required to confirm our findings.
Prior to acrylamide-gene interaction analysis, we examined the association between dietary acrylamide and advanced prostate cancer risk after 20.3 years of follow-up. The (statistically non-significant) inverse association we observed across quintiles of acrylamide intake and advanced prostate cancer risk in never-smokers after 13.3 years of follow-up (Citation8) did not persist after 20.3 years of follow-up. Thus, the previously observed inverse association may have been due to chance since the association was not statistically significant. In our earlier study, we interpreted the putative inverse association in the context of the associations we found with other hormone-related cancers. A Swedish prospective study (Citation9), conducted after our study, also reported an (statistically non-significant) inverse association between acrylamide intake and advanced prostate cancer risk in never-smokers. However, the third other study (Citation10) did not find an association. Given this limited and inconsistent evidence, we examined acrylamide-gene interactions in order to better understand a possible association between acrylamide intake and prostate cancer. For that matter, we selected genetic variants involved in acrylamide metabolism and the hypothesized mechanisms of acrylamide-induced carcinogenesis: genotoxicity (DNA repair), a sex hormonal effect and oxidative stress (Citation11). While we observed two SNPs (rs1800566 in NQO1 and rs2301241 in TXN) that showed statistically significant multiplicative interaction in the total study population, we did not identify SNPs that survived multiple testing correction or multiple SNPs in the same gene or SNPs that showed a clear dose-response relationship for acrylamide in strata of the genotypes. Thus, the current study does not provide evidence for an interaction between selected genetic variants and acrylamide intake on advanced prostate cancer risk. Consequently, this study does not increase the strength of evidence for a causal association between acrylamide intake and prostate cancer risk.
Preferably, we would have performed acrylamide-gene interactions in never-smokers to eliminate any confounding effects by smoking (which is an important source of acrylamide) and to be able to shed more light on the previously reported inverse association for this group. However, the number of available cases in this group was too small for this purpose and therefore we combined never-smokers with former smokers who had quit smoking more than 10 years before baseline. Unpublished results by our group did not show an association between former smoking and advanced prostate cancer risk and other studies reported mixed results (Citation31,Citation32), which made us decide to combine these two groups. Of course, residual confounding by former smoking may still have been present, but we tried to eliminate this as much as possible by detailed adjustment for (former) smoking. It is therefore not to be expected that analyzing this non-smoking group has rendered importantly different results than analyzing never-smokers would have done.
Strengths of our study are the prospective nature, the (>96%) completeness (Citation45), and duration of cancer follow-up. A drawback of our study is the one-time baseline assessment of exposures and covariables. However, older people are likely to have relatively stable diets over time. Another drawback of our study is that we focused on functional candidate genes and variants associated with acrylamide metabolism and the hypothesized mechanisms of acrylamide-induced carcinogenesis. Therefore, we may have missed variants in genes that possibly interact with dietary acrylamide on advanced prostate cancer risk. Furthermore, even though we used data from a large cohort study, a relatively small number of cases per cell in acrylamide-gene analysis may have possibly resulted in limited statistical power to show statistically significant multiplicative interactions. Finally, baseline characteristics differed not significantly between participants who provided toenail clippings for DNA-analyses and participants who did not, except for level of education and cigarette smoking status. However, given the prospective cohort design of our study this is not likely to have biased our results.
In conclusion, the Netherlands Cohort Study on diet and cancer does not provide clear evidence for an interaction between acrylamide intake and selected genetic variants on advanced prostate cancer risk and does not increase the strength of evidence for a causal association between acrylamide intake and prostate cancer risk.
Conflict of interest
Dr. Leo Schouten received compensation as a member of a scientific advisory panel on acrylamide risk assessment of the European Food Safety Authority. The other authors have no conflict of interests to declare.
HNUC_A_1460682_Supplementary-material.zip
Download Zip (84.9 KB)Acknowledgments
We thank the study participants, the Netherlands Cancer Registry, the Dutch Pathology Registry, and the Biobank of the Maastricht University Medical Center. We thank Dr. Sandra Bausch as initiator of the NLCS study, together with Prof. Piet van den Brandt. We also thank Sacha van de Crommert, Jolanda Nelissen, Conny de Zwart, Ellen Dutman, Henny Brants, and Annemie Pisters for their assistance with data entry or data management, Harry van Montfort for programming assistance, and Stijn Lumeij, Kristien Lemmens, Joy Goessens, and Leonie Jonkers for technical assistance with DNA isolation and genotyping. Janneke Hogervorst is a postdoctoral research fellow of the Research Foundation Flanders – FWO (12J9516N).
Additional information
Funding
References
- Brawer MK: Hormonal therapy for prostate cancer. Rev Urol 8(Suppl 2), S35–S47, 2006.
- Ferlay J, Soerjomataram I, Dikshit R, Eser S, Mathers C, et al.: Cancer incidence and mortality worldwide: sources, methods and major patterns in GLOBOCAN 2012. Int J Cancer 136(5), E359–E386, 2015. doi:10.1002/ijc.29210.
- Discacciati A, and Wolk A: Lifestyle and dietary factors in prostate cancer prevention. Recent Results Cancer Res 202, 27–37, 2014. doi:10.1007/978-3-642-45195-9_3.
- Zhou CK, Check DP, Lortet-Tieulent J, Laversanne M, Jemal A, et al.: Prostate cancer incidence in 43 populations worldwide: an analysis of time trends overall and by age group. Int J Cancer 138(6), 1388–1400, 2016. doi:10.1002/ijc.29894.
- Cook LS, Goldoft M, Schwartz SM, and Weiss NS: Incidence of adenocarcinoma of the prostate in Asian immigrants to the United States and their descendants. J Urol 161, 152–155, 1999.
- Kimura T: East meets West: ethnic differences in prostate cancer epidemiology between East Asians and Caucasians. Chin J Cancer 31(9), 421–429, 2012. doi:10.5732/cjc.011.10324.
- Pelucchi C, Bosetti C, Galeone C, and La Vecchia C: Dietary acrylamide and cancer risk: an updated meta-analysis. Int J Cancer 136(12), 2912–2922, 2015. doi:10.1002/ijc.29339.
- Hogervorst JG, Schouten LJ, Konings EJ, Goldbohm RA, and van den Brandt PA: Dietary acrylamide intake and the risk of renal cell, bladder, and prostate cancer. Am J Clin Nutr 87, 1428–1438, 2008.
- Larsson SC, Akesson A, and Wolk A: Dietary acrylamide intake and prostate cancer risk in a prospective cohort of Swedish men. Cancer Epidemiol Biomarkers Prev 18(6), 1939–1941, 2009. doi:10.1158/1055-9965.epi-09-0280.
- Wilson KM, Giovannucci E, Stampfer MJ, and Mucci LA: Dietary acrylamide and risk of prostate cancer. Int J Cancer 131(2), 479–487, 2012. doi:10.1002/ijc.26383.
- Besaratinia A, and Pfeifer GP: A review of mechanisms of acrylamide carcinogenicity. Carcinogenesis 28(3), 519–528, 2007. doi:10.1093/carcin/bgm006.
- Besaratinia A, and Pfeifer GP: Genotoxicity of acrylamide and glycidamide. J Natl Cancer Inst 96, 1023–1029, 2004.
- Hogervorst JG, Schouten LJ, Konings EJ, Goldbohm RA, and van den Brandt PA: A prospective study of dietary acrylamide intake and the risk of endometrial, ovarian, and breast cancer. Cancer Epidemiol Biomarkers Prev 16(11), 2304–2313, 2007. doi:10.1158/1055-9965.epi-07-0581.
- Reuter S, Gupta SC, Chaturvedi MM, and Aggarwal BB: Oxidative stress, inflammation, and cancer: how are they linked? Free Radic Biol Med 49(11), 1603–1616, 2010. doi:10.1016/j.freeradbiomed.2010.09.006.
- Finkel T, and Holbrook NJ: Oxidants, oxidative stress and the biology of ageing. Nature 408, 239–247, 2000. doi:10.1038/35041687.
- Khandrika L, Kumar B, Koul S, Maroni P, and Koul HK: Oxidative stress in prostate cancer. Cancer Lett 282(2), 125–136, 2009. doi:10.1016/j.canlet.2008.12.011.
- Kulathinal S, Karvanen J, Saarela O, and Kuulasmaa K: Case-cohort design in practice – experiences from the MORGAM Project. Epidemiol Perspect Innov 4, 15, 2007. doi:10.1186/1742-5573-4-15.
- Van den Brandt PA, Schouten LJ, Goldbohm RA, Dorant E, and Hunen PM: Development of a record linkage protocol for use in the Dutch Cancer Registry for Epidemiological Research. Int J Epidemiol 19, 553–558, 1990.
- van den Brandt PA, Goldbohm RA, van 't Veer P, Volovics A, Hermus RJ, et al.: A large-scale prospective cohort study on diet and cancer in The Netherlands. J Clin Epidemiol 43, 285–295, 1990.
- Sobin LH, Gospodarowicz MK, and Wittekind C: TNM Classification of Malignant Tumors, 7th ed. Oxford, UK: Wiley-Blackwell; 2009.
- Goldbohm RA, van den Brandt PA, Brants HA, van't Veer P, Al M, et al.: Validation of a dietary questionnaire used in a large-scale prospective cohort study on diet and cancer. Eur J Clin Nutr 48, 253–265, 1994.
- Goldbohm RA, van 't Veer P, van den Brandt PA, van 't Hof MA, Brants HA, et al.: Reproducibility of a food frequency questionnaire and stability of dietary habits determined from five annually repeated measurements. Eur J Clin Nutr 49, 420–429, 1995.
- Konings EJ, Hogervorst JG, van Rooij L, Schouten LJ, Sizoo EA, et al.: Validation of a database on acrylamide for use in epidemiological studies. Eur J Clin Nutr 64, 534–540, 2010. doi:10.1038/ejcn.2010.17.
- Hogervorst JG, van den Brandt PA, Godschalk RW, van Schooten FJ, and Schouten LJ: The influence of single nucleotide polymorphisms on the association between dietary acrylamide intake and endometrial cancer risk. Sci Rep 6(34902), 2016. doi:10.1038/srep34902.
- Huang YF, Chen ML, Liou SH, Chen MF, Uang SN, et al.: Association of CYP2E1, GST and mEH genetic polymorphisms with urinary acrylamide metabolites in workers exposed to acrylamide. Toxicol Lett 203(2), 118–126, 2011. doi:10.1016/j.toxlet.2011.03.008.
- Hogervorst JG, Godschalk RW, van den Brandt PA, Weijenberg MP, Verhage BA, et al.: DNA from nails for genetic analyses in large-scale epidemiologic studies. Cancer Epidemiol Biomarkers Prev 23(12), 2703–2712, 2014. doi:10.1158/1055-9965.epi-14-0552.
- Gabriel S, Ziaugra L, and Tabbaa D: SNP genotyping using the Sequenom MassARRAY iPLEX platform. Curr Protoc Hum Genet Chapter 2, Unit 2.12, 2009. doi:10.1002/0471142905.hg0212s60.
- Benjamini Y, and Hochberg Y: Controlling the false discovery rate: a practical and powerful approach to multiple testing. J R Stat Soc Series B (Methodological) 57, 289–300, 1995.
- Lin DY, and Wei LJ: The robust inference for the cox proportional hazards model. J Am Stat Assoc 84(408), 1074–1078, 1989. doi:10.2307/2290085.
- Schoenfeld D: Partial residuals for the proportional hazards regression model. Biometrika 69(1), 239–241, 1982. doi:10.2307/2335876.
- Huncharek M, Haddock KS, Reid R, and Kupelnick B: Smoking as a risk factor for prostate cancer: a meta-analysis of 24 prospective cohort studies. Am J Public Health 100(4), 693–701, 2010. doi:10.2105/ajph.2008.150508.
- Islami F, Moreira DM, Boffetta P, and Freedland SJ: A systematic review and meta-analysis of tobacco use and prostate cancer mortality and incidence in prospective cohort studies. Eur Urol 66(6), 1054–1064, 2014. doi:10.1016/j.eururo.2014.08.059.
- Geybels MS, van den Brandt PA, Schouten LJ, van Schooten FJ, van Breda SG, et al.: Selenoprotein gene variants, toenail selenium levels, and risk for advanced prostate cancer. J Natl Cancer Inst 106, dju003, 2014. doi:10.1093/jnci/dju003.
- Geybels MS, van den Brandt PA, van Schooten FJ, and Verhage BA: Oxidative stress-related genetic variants, pro- and antioxidant intake and status, and advanced prostate cancer risk. Cancer Epidemiol Biomarkers Prev 24(1), 178–186, 2015. doi:10.1158/1055-9965.epi-14-0968.
- Liou GY, and Storz P: Reactive oxygen species in cancer. Free Radic Res 44(5), 479–496, 2010. doi:10.3109/10715761003667554.
- Van Blarigan EL, Ma J, Kenfield SA, Stampfer MJ, Sesso HD, et al.: Plasma antioxidants, genetic variation in SOD2, CAT, GPX1, GPX4, and prostate cancer survival. Cancer epidemiology, biomarkers & prevention: a publication of the American Association for Cancer Research, cosponsored by the American Society of Preventive Oncology 23, 1037–1046, 2014. doi:10.1158/1055-9965.EPI-13-0670.
- Stack E, and DuBois RN: Regulation of cyclo-oxygenase-2. Best Pract Res Clin Gastroenterol 15(5), 787–800, 2001. doi:10.1053/bega.2001.0235.
- Uotila P, Valve E, Martikainen P, Nevalainen M, Nurmi M, et al.: Increased expression of cyclooxygenase-2 and nitric oxide synthase-2 in human prostate cancer. Urol Res 29(1), 23–28, 2001.
- Yang X, Li B, Si T, Liu Y, and Guo Z: Association between the 8473T>C polymorphism of PTGS2 and prostate cancer risk: a metaanalysis including 24,716 subjects. Onkologie 36(4), 182–186, 2013. doi:10.1159/000349951.
- Hu Z, Miao X, Ma H, Wang X, Tan W, et al.: A common polymorphism in the 3′UTR of cyclooxygenase 2/prostaglandin synthase 2 gene and risk of lung cancer in a Chinese population. Lung Cancer 48(1), 11–17, 2005. doi:10.1016/j.lungcan.2004.09.004.
- Yang H, Gu J, Lin X, Grossman HB, Ye Y, et al.: Profiling of genetic variations in inflammation pathway genes in relation to bladder cancer predisposition. Clin Cancer Res 14(7), 2236–2244, 2008. doi:10.1158/1078-0432.ccr-07-1670.
- Melis JP, Luijten M, Mullenders LH, and van Steeg H: The role of XPC: implications in cancer and oxidative DNA damage. Mutat Res 728(3), 107–117, 2011. doi:10.1016/j.mrrev.2011.07.001.
- Chen Y, Zhong H, Gao JG, Tang JE, and Wang R: A systematic review and meta-analysis of three gene variants association with risk of prostate cancer: an update. Urol J 12(3), 2138–2147, 2015.
- Francisco G, Menezes PR, Eluf-Neto J, and Chammas R: XPC polymorphisms play a role in tissue-specific carcinogenesis: a meta-analysis. Eur J Hum Genet 16(6), 724–734, 2008. doi:10.1038/ejhg.2008.6.
- Goldbohm RA, van den Brandt PA, and Dorant E: Estimation of the coverage of Dutch municipalities by cancer registries and PALGA based on hospital discharge data. Tijdschr Soc Gezondheidsz 72, 80–84, 1994.