Abstract
Epilepsy in the horse is diagnosed based on clinical signs, but diagnosing can be difficult if a grand mal is not present. The future prospects of the horse and potentially the safety of the owner depend on an accurate diagnosis. This review presents information on epilepsy and focuses on the diagnostic potential of (Ambulatory) electroencephalography ((A) EEG). An epileptic seizure is a brain disorder, which expresses itself as a recurrent episode of involuntary abnormal behaviour. The aetiology can originate from inside or outside the brain or is idiopathic. Besides those categories, seizures can be classified as generalised or partial. A typical generalised tonic–clonic seizure is characterised by the prodrome, the ictus and the post-ictal phase. EEG is the graphic recording of rhythmic bioelectric activity which originates predominantly from the cerebral cortex. In human medicine, the 10/20 international basis system for electrode placement is used. This makes comparison more reliable and consistent. The normal human brainwaves recorded are alpha, beta, theta and delta waves. In the horse, fewer descriptions of normal signals are available. In humans suffering from epilepsy, spikes, complexes, spike-and-wave discharges and rhythmical multi-spike activity are seen. In horses suffering from epilepsy, spikes, sharp waves and spike-and-wave discharges are seen. In humans, AEEG has numerous advantages above short-duration EEG in diagnosing epilepsy or intracranial pathology. In future, AEEG might be useful to record brain signals in awake horses expressing their behaviour under natural circumstances.
1. Introduction
In all species epilepsy is exhausting for the patient and carries a high risk of accidents (Aleman et al. Citation2006). Clinical signs of an epileptic grand mal in the horse are recurrent episodes of the loss of consciousness, while muscles contract uncontrollably. It can start after excitation and can begin with abnormal head and neck bending over backwards and trembling (Mayhew Citation1989; De Lahunta Citation2001; Aleman et al. Citation2006). The future prospects of the horse and maybe the safety of the owner depend on the ability to accurately diagnose epilepsy. In addition, it is also important for insurance companies to have a definitive diagnose of epilepsy since a horse with epilepsy is often not able to fulfil its purpose (Rose and Hodgson Citation2000). Treatment is possible, however daily medication impairs its sports career due to the medication control program. Some horses would be needing drugs on a daily basis (Reed et al. Citation2004).
Today, epilepsy in the horse is diagnosed mostly based on clinical signs (Reed et al. Citation2004) and these are not always obvious if a grant mal is not present to its full extend. Electroencephalography (EEG) has become increasingly available for horses (Williams et al. Citation2012). In human medicine, especially ambulatory EEG (AEEG) has proven to be useful in diagnosing epilepsy (Barlow Citation1993c; Noachtar and Rémi Citation2009; Shih et al. Citation2009). Even though some research in horses has been performed on diagnostic techniques regarding epilepsy (Mittel Citation1987; Mayhew Citation1989; Lewin and Tönhardt Citation1998; Lacombe et al. Citation2001; Aleman et al. Citation2006; Williams et al. Citation2008), information on the classification and the technique of EEG in horses is rather limited (Lacombe et al. Citation2012) whilst AEEG is a potential diagnostic tool for epilepsy in horses (van der Ree Citation2011).
In this review the clinical symptoms, the pathophysiology, etiology and current diagnostic tools in horses suffering from epilepsy are discussed. In addition, the advantages of AEEG as a diagnostic tool for epilepsy in humans and the potential value of this technique in horses will be discussed.
2. The pathophysiology and aetiology of epilepsy in horses
Epilepsy is a brain disorder, which expresses itself as a recurrent seizure of involuntary abnormal behaviour (Rose and Hodgson Citation2000; De Lahunta Citation2001; Robinson et al. Citation2003; Waterhouse Citation2003; Reed et al. Citation2004; Shih et al. Citation2009). Neurons receive large quantities of inhibitory and excitatory neurotransmitters. The summations of these neurotransmitters determine the activity of the neuron. The neuronal membrane potential changes by sodium and potassium exchange within the cell environment. When this environment is altered, the regulation of the neuronal discharges in the brain will differ. This can result in an epileptic seizure. Intracranial or extracranial diseases can alter this environment. During a seizure, a hyperactive, hypersynchronic electric discharge exists in neurons of the cerebrum (De Lahunta Citation2001; Robinson et al. Citation2003; Reed et al. Citation2004). This can be caused by an imbalance of inhibitory and excitatory neurotransmitters, which can influence the depolarisation threshold (De Lahunta Citation2001; Reed et al. Citation2004).
Convulsions seem to happen more often in foals, because larger and older animals tend to have a higher threshold for seizures (Mayhew Citation1989; Rose and Hodgson Citation2000; Robinson et al. Citation2003; Reed et al. Citation2004). Seizure threshold differs between individuals and is stipulated by neuronal excitability and environment. The metabolic and structural bases for the threshold are thought to be genetic (De Lahunta Citation2001; Robinson et al. Citation2003; Reed et al. Citation2004; Aleman et al. Citation2006). Healthy individuals have a high threshold, and thus seizures do not occur. When patients with a low seizure threshold are stimulated, for instance by photic stimulation, a seizure can appear. In patients who have a very low threshold, seizures can occur spontaneously (De Lahunta Citation2001; Robinson et al. Citation2003; Reed et al. Citation2004).
Three origins of epilepsy are identified: (1) extracranial: for instance medication, toxins and metabolic disorders; (2) intracranial: for instance encephalitis, scar tissue, brain trauma and tumours and (3) idiopathic. Because the idiopathic form has no known cause, it is called primary epilepsy. The other forms of epilepsy are secondary to other diseases and are therefore called secondary epilepsy (Colahan et al. Citation1999; De Lahunta Citation2001; Reed et al. Citation2004). Besides the above-mentioned categorisation, seizures can be classified as generalised or partial (focal). A partial seizure originates from a small part of the cerebral cortex in which a small amount of neurons discharge spontaneously. This discharge occurs mostly in the periphery of the cerebrum. In case excited neurons induce surrounding neurons to discharge spreading the seizure progressively, it is called a generalised seizure (Colahan et al. Citation1999; Rose and Hodgson Citation2000; De Lahunta Citation2001; Robinson et al. Citation2003). Generalised seizures are the result of diffuse sensitivity of the brain, or a deep, centrally localised epileptiform focus; in those cases the entire cerebral cortex is involved (Rose and Hodgson Citation2000; Reed et al. Citation2004).
3. Clinical signs
The appearance of an epileptic fit depends on the localisation of the focus, the routes of spreading and the parts of the brain that are involved (De Lahunta Citation2001; Robinson et al. Citation2003). In a partial seizure the body contracts partially and consciousness is retained. The clinical signs sometimes are indicative of the location of the origin of the electrical discharge and are mostly asymmetrical in presentation (Rose and Hodgson Citation2000; De Lahunta Citation2001). For instance, a frequently observed clinical sign is involuntary head and neck movements and muscle contractions in the nose area. This indicates that the focus is localised in the motor area of the cerebrum. There are no signs to predict the duration of the seizure or whether it will proceed into a generalised seizure (Mayhew Citation1989; De Lahunta Citation2001; Aleman et al. Citation2006). During a generalised seizure, the whole body is involved with a loss of consciousness and the clinical signs being symmetrical in appearance (Mayhew Citation1989).
The course of a typical generalised tonic–clonic seizure has three stages: the prodrome, the ictus and the post-ictal phases. The first stage is the prodrome. This period is characterised by an abnormal behaviour (Mayhew Citation1989). The most characteristic symptom is restlessness. Other horses may be more affectionate, hyperaesthetic or have a wide-eyed stare (Colahan et al. Citation1999; Rose and Hodgson Citation2000; Reed et al. Citation2004). The duration can be seconds or days. The second stage is the real seizure, the ictus. The muscles of the head, neck and face contract abnormally (Mayhew Citation1989). The lip retracts and the jaw clamps (Colahan et al. Citation1999; Rose and Hodgson Citation2000). The frequency of the muscle contractions increases and movement becomes more prominent. The contractions spread to the whole body, which make the horses movements unstable and uncoordinated. This worsens to the stage that eventually the horse drops down and loses its consciousness while the tonic muscle contractions start. Especially in the extensors hypercontraction appears. The tonic convulsions change into clonic contractions causing the whole body to shock violently. Foam can appear around the mouth as a result of hypersecretion of saliva. Also clonic convulsions of the chewing muscles, wide-eyed stare, urination and defecation may occur. Most horses sweat extensively. In the end the clonic contractions disappear and the muscles relax. The duration and intensity of the ictus differs among patients. The ictus can vary from seconds to hours (Rose and Hodgson Citation2000; De Lahunta Citation2001). In some cases the ictus evolves in a status epilepticus: because of the amount of energy that is lost during a seizure, the nervous system is unable to return to normal. This can lead to insufficient breathing caused by disturbed signal transduction in the brain. Some patients remain in the status epilepticus and die of hypoxia (De Lahunta Citation2001).
The last and third stage is the post-ictal phase. The post-ictal phase can last several hours or even days. With the start of the post-ictal phase the horse regains its consciousness, breathing is accelerated and the animal stands up. Most horses are disorientated, are uncoordinated, walk in circles, can experience insufficient visibility, walk into objects or explore the formerly familiar surroundings (Aleman et al. Citation2006). The horses often do not respond to the owner. The animal can be insecure and confused, which can result in aggressiveness. Patients drink and eat greedily and seem exhausted (Mayhew Citation1989; Colahan et al. Citation1999; De Lahunta Citation2001).
There is a high risk of trauma during a seizure especially in the ictal phase, and it often contains head trauma with corneal ulcers. Also long bone fractures are a common finding (Mayhew Citation1989). In other cases, horses recover without permanent consequences (Mayhew Citation1989; Rose and Hodgson Citation2000; De Lahunta Citation2001).
4. Diagnostic tools
4.1. Basic principles of EEG
EEG is the graphic recording of rhythmic bioelectric activity which originates predominantly from the cerebral cortex (Lacombe et al. Citation2001). It is a method to record brain waves by placing electrodes at several locations on the scalp of a patient (Rose and Hodgson Citation2000). The system measures potential differences between the electrodes. The electrical signals from the brain are passed from the electrodes through electrode wires to a computer box. This box converts the signals into graphic recordings made visible on a computer screen, which then can be analysed by neurologists.
4.2. EEG in humans
Normal and abnormal brain signals can be recognised using EEG in humans (Empson Citation1986a; Liasis et al. Citation2006; Beleza et al. Citation2009; Tassi et al. Citation2009; Capovilla et al. Citation2011). An anatomical placement system for EEG electrodes was developed in 1958 by Jasper called the 10/20 international system for electrode placement (Empson Citation1986a; Klem et al. Citation1999). This system has a fixed, reproducible structure for placement of the electrodes which enables obtaining electrical signals generated by the whole brain. The surface of the scalp is symmetrically divided in segments in order to place the electrodes systematically. Because of its accuracy, the Jasper method is still used in human medicine to date. The placement of the electrodes influences the outcomes of EEG data between individuals. As a consequence, using a uniform system for electrode placement internationally at different institutes makes comparison more reliable and consistent (Empson Citation1986a; Klem et al. Citation1999).
In order to maximise the chances of diagnosing epileptiform insults, it can be beneficial to be able to diagnose epilepsy inter-ictally. In humans, inter-ictal epileptic discharges are frequently seen in the temporal lobe, especially in patients with temporal lobe epilepsy (TLE) (Beleza et al. Citation2009; Noachtar and Rémi Citation2009; Tassi et al. Citation2009).
4.3. Normal EEG findings in humans
The normal human brainwaves seen in an EEG are alpha, beta, theta and delta waves () (Berger Citation1929; Walter Citation1936; Empson Citation1986a; Barlow Citation1993a, Citation1993b; Engel Citation2005). The frequency and amplitude of delta waves, also visible during deep sleep, are 0.5–3.5 Hz and 10–20 µV, respectively. For theta waves, mostly seen during drowsiness, these values are 4–7 Hz and 20–40 µV, respectively. When people are in a state of relaxed wakefulness with their eyes closed, sinusoidal alpha waves are visible on the occipital lobe with a frequency and amplitude of 8–13 Hz and 25–100 µV, respectively. Alpha waves can be blocked during a visual task. Beta waves have a frequency and amplitude of 14–40 Hz and 5–60 µV, respectively. Sleep spindles are periodically seen during all stages of sleep with a frequency of 12–15 Hz (Loomis et al. Citation1936). They appear with an amplitude similar to the stage of sleep () (Loomis et al. Citation1936; Empson Citation1986a, Citation1986b; Barlow Citation1993a, Citation1993b; Noachtar and Rémi Citation2009). Less frequently observed waves in the human EEG are, for instance, lambda waves occurring during visual exploration and cone waves occurring from infancy to childhood (Engel Citation2005). Chewing and eye movements are recognisable on the EEG chart as electromyographic (EMG) activity (Dement and Kleitman Citation1957; Engel Citation2005; Priyadharsini and Rajan Citation2012). Chewing (EMG) is visible as dense activity with a recurrent pattern and has a higher frequency and amplitude than that of EEG recorded waves (Engel Citation2005; Priyadharsini and Rajan Citation2012). Eye movements result in loop-shaped signals with variable duration and amplitude with a maximum amplitude on the electrodes placed frontally (Dement and Kleitman Citation1957; Engel Citation2005; Priyadharsini and Rajan Citation2012).
Figure 1. EEG recordings of alpha, beta, theta and delta waves in humans. Notes: The electrodes are named after their location on the brain lobes; P: parietal; O: occipital; F: frontal; C: central; Fp: frontopolar. The even numbers (2, 4) are electrodes placed on the right side of the head and the odd numbers (1, 3) electrodes placed on the left side of the head. The lines show the electrical activity measured between the electrodes named in front of the line. A: alpha waves visible on the occipital regions (P4-O2, P3-O1); B: beta waves with the greatest activity on the frontocentral regions (Fp1-F3, F3-C3); C: generalised theta waves; D: generalised delta waves. (source: this figure is based on information obtained from Section 3, Chapters 3–10, pp. 27–128 of Engel (2005)).
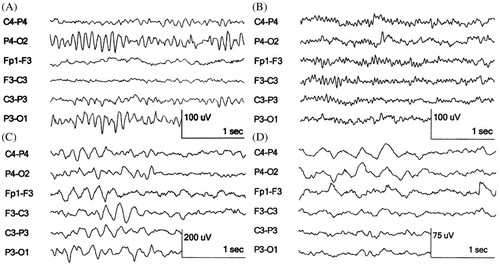
Table 1. Normal human EEG signals.
4.4. EEG in the horse
Nowadays the diagnosis ‘epilepsy’ in horses is made by the interpretation of clinical signs, sometimes supported with EEG examination. Even though EEG in horses has already been performed since 1985, it is still not used routinely and no standard reproducible electrode placement based on the equine anatomy exists (Mysinger et al. Citation1985; Lewin and Tönhardt Citation1998; Lacombe et al. Citation2001; Aleman et al. Citation2006; Williams et al. Citation2008). Williams et al. (Citation2008) examined EEG's recordings taken at several stages of vigilance in five healthy horses. The electrode placement used was based on the human system (Williams et al. Citation2008). Wakefulness, drowsiness, slow wave sleep (SWS) and rapid eye movement (REM) were identified as stages of vigilance (). Wakefulness was used to describe a horse in the alert state, actively engaged in exploring its environment (Williams et al. Citation2008). These authors determined that the state of wakefulness had the worst results due to movement artefacts. Epileptic signals are prominent in the human EEG during sleep (Tassinari et al. Citation2009; Overvliet et al. Citation2010), but horses only sleep three hours a day on average (Williams et al. Citation2008). Since epileptic seizures as well as inter-ictal activity are unpredictable and can occur during normal day activities (De Lahunta Citation2001; Robinson et al. Citation2003), it would be beneficial to be able to record during wakefulness as well as during sleep.
Table 2. Normal EEG signals in horses based on the literature.
Table 3. Pathologic EEG signals indicating epilepsy in humans.
4.5. Normal EEG findings in the horse
As in humans, sleep waves are visible on the EEG of horses ( and , ) (Aleman et al. Citation2006; Williams et al. Citation2008). Signals indicating SWS appear with a frequency and amplitude of 1–8 Hz and 40–90 µV, respectively. During drowsiness and REM sleep, waves resembling human theta waves are seen with a frequency and amplitude of 4 Hz and 30–40 µV, respectively. During drowsiness, waves resembling human beta waves are seen with a frequency and amplitude of 20 Hz and 10–20 µV, respectively. REM sleep results in a low-amplitude pattern with a frequency and amplitude of 20–30 Hz and 5–10 µV, respectively. Sleep spindles have a frequency and amplitude of 10–14 Hz and 30–40 µV, respectively (Williams et al. Citation2008). However, how these sleep stages are defined remains unclear.
Figure 2. Normal EEG signals: chewing, eye movements and sleep spindles. Notes: s: seconds; µV: micro voltage; sleep spindles: →← and eye movements: *. Figure derived from horses is similar to humans.
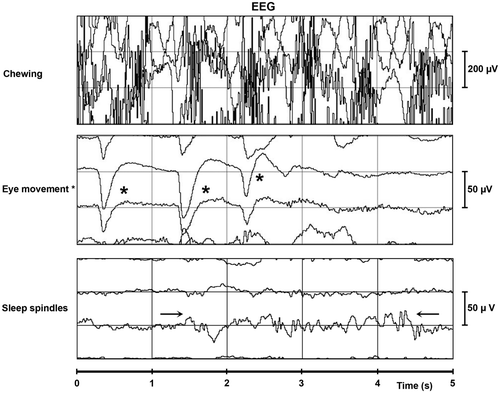
Eye movements () result in large loop-like signals with variable duration and amplitude (Williams et al. Citation2008). The interpretation of EEG signals in horses are largely based on information in human medicine (Tables ) (Williams et al. Citation2008). Unlike beta waves, which originate from the frontocentral brain lobes in humans, alpha waves have not been described in equine literature (Williams et al. Citation2008). This might be explained by the anatomical impossibility of placing electrodes on the posterior of the brain in the horse, from where alpha waves originate (Engel Citation2005). Moreover, a state of relaxed wakefulness with the eyes closed, as is the definition of alpha waves, is not part of the horse's frequently observed behavior.
Table 4. Pathologic EEG signals indicating epilepsy in horses.
4.6. Human signals indicating pathology for instance epilepsy
In patients suffering from epilepsy, spikes, spike-and-wave complexes, sharp wave-slow wave complexes and multi-spike activity are seen () (Barlow Citation1993c; Engel Citation2005; Noachtar and Rémi Citation2009; Seneviratne et al. Citation2012). They can occur locally or generalised, which is visible on the AEEG in a small number of electrode combinations or on all the electrode combinations, respectively. Spikes () occur solitary or multiple with a duration of <70 ms and slower spikes of 70–200 ms. The ascending (negative) phase of the peak in both types of spike show an increase in the amplitude of 2 µV/ms.
Figure 3. Examples of abnormal EEG activity as seen in epilepsy or intracranial pathology in humans. (source: this figure is based on information obtained from Section 3, Chapters 13 and 14, pp. 143-184 of Engel (2005)).
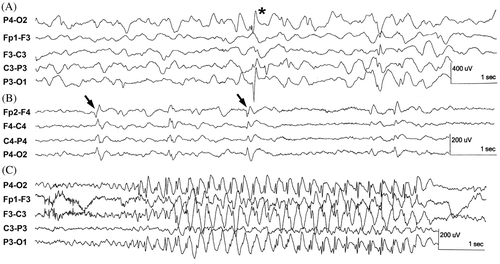
Spike and wave complexes consist of multiple phases () (Seneviratne et al. Citation2012). First, a spike is visible with a negative polarity and a duration and amplitude of 10 ms and 25–50 µV, respectively. Then a positive transient phase with a duration of 100–150 ms appears, followed by a second spike. The main negative component of this complex lasts for 30–60 ms and is followed by a dome-shaped surface negative wave lasting for 150–200 ms.
Generalised 3 Hz spike-wave complexes are seen in patients with clinical absence seizures and last longer than 4s (). The most common inter-ictal findings are spikes and spike-wave complexes. The frequency of sharp wave-slow wave complexes and spike-and-wave complexes are 2 Hz and 2–3 Hz, respectively. Their amplitude is 100–300 µV and occur in fixed rhythmic recurring combinations. Generalised polyspikes are spike-wave complexes which have a frequency above 3–4 Hz () and are classified as generalised inter-ictal epileptiform discharges (Engel Citation2005). They are often seen in patients diagnosed with juvenile myoclonic epilepsy (JME) (Noachtar and Rémi Citation2009). Other inter-ictal activity is intermittent rhythmic delta activity with a frequency of maximally 4 Hz and an amplitude of 50–100 µV, and seen as a burst of rhythmic sinusoidal delta activity on the occipital electrodes (Seneviratne et al. Citation2012). Slow delta waves (1–2 Hz) can be observed as a result of intracranial pathology, for example traumatic-induced brain oedema ().
Figure 4. Example of delta wave in humans due to, for example, traumatic induced brain oedema. (source: this figure is based on information obtained from Section 3, Chapters 3–10, pp. 27–128 and Chapters 13 and 14, pp. 143–184 of Engel (2005)).
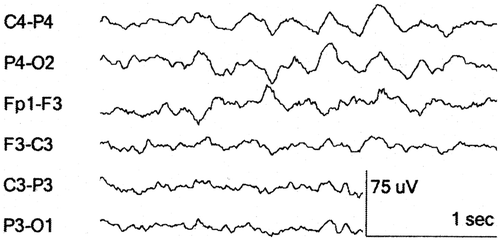
The frequency and amplitude of multi-spikes are 15–30 Hz and above 100 µV, respectively. They are seen during the tonic stage of a seizure. Their amplitude increases during the attack. During the clonic stage the spike-and-wave activity is visible, while the post-ictal phase shows slow activity (Barlow Citation1993c; Noachtar and Rémi Citation2009). Inter-ictal signals have a duration of a few seconds and occur solely or in bursts while ictal signals can consists of repetitive higher amplitude discharges () with an abrupt onset and termination with longer duration (Seneviratne et al. Citation2012).
4.7. EEG signals indicating pathology in horses for instance epilepsy
Also in horses, EEG is a sensitive tool with regard to diagnosis for intracranial disorders, for instance epilepsy (Lacombe et al. Citation2001; Aleman et al. Citation2006). Several abnormal brain waves are identified on the equine EEG. Spikes, sharp waves and spike-and-wave discharges have a frequency of <70 ms, 70–200 ms and of 6 Hz, respectively, and are recorded mostly on the central electrodes () (Aleman et al. Citation2006; Williams et al. Citation2008). Since movement artefacts are prominent in the horse EEG according to some authors and as horses are usually attached to a fixed computer, the animals often are sedated during the recordings (Lacombe et al. Citation2001; Aleman et al. Citation2006; Williams et al. Citation2008, Citation2012). Sedatives like xylazine, detomidine and other drugs such as guaifenesin, thiopental sodium, halothane and isoflurane result in drug-induced artefacts (Lacombe et al. Citation2001; Aleman et al. Citation2006).
For instance, horses sedated with xylazine show a hyper synchronous signal with a dominant frequency and amplitude of 1–3 Hz and 10–70 µV, respectively, and an overlying signal with a mixed frequency and amplitude of 5–10 Hz and 10–40 µV, respectively. Ketamine causes an increase of high-voltage slow activity with a dominant frequency and amplitude of 1–3 Hz and 20–100 µV, respectively, and a superimposed activity with a mixed irregular frequency and amplitude of 6–10 Hz and 10–50 µV, respectively (Purohit et al. Citation1981). The drug-induced background artefacts make it possible that some epileptic activity will be missed. As medicines as well as different dosages will create individual EEG outcomes, it will be hard to compare recordings.
4.8. Advantages of AEEG in human medicine
In humans, AEEG is performed routinely on neurophysiology departments to diagnose, locate and define possible abnormal brain signals (Marsan and Zivin Citation1970; Sheridan and Sato Citation1985; Ebersole Citation1987; Patel et al. Citation2009; Shih et al. Citation2009). The device can be worn for several days increasing the chances of recording pathologic electrical activity. Normal day activities and sleep episodes can be recorded enabling coupling the brain activity with behavioural changes. It also increases the chance to record inter-ictal epileptic signals (Marsan and Zivin Citation1970; Bridgers and Ebersole Citation1985; Sheridan and Sato Citation1985; Ebersole Citation1987). However, the electrical disturbance of the brain is not always continuously present (Reed et al. Citation2004). An AEEG recording lasting more than 24 hours instead of a routine EEG recording lasting 30 minutes increases the chance of capturing an actual seizure or inter-ictal activity (Marsan and Zivin Citation1970; Bridgers and Ebersole Citation1985; Sheridan and Sato Citation1985). Therefore, seizures are diagnosed more accurately with the help of AEEG (Sheridan and Sato Citation1985; Patel et al. Citation2009; Shih et al. Citation2009). In addition, it can be used to differentiate between partial and generalised epileptic seizures or slow rhythmic delta waves indicative of intracranial masses and to monitor the effect of treatment (Robinson et al. Citation2003). A 24-hour AEEG recording results in 64% increase in capturing inter-ictal epileptiform abnormalities and a 21-fold increase in seizure recording compared with the routine EEG (Ebersole Citation1987).
5. Discussion and conclusion
As described above, research has been performed on diagnosing epilepsy using EEG in equine medicine. EEG interpretation in horses is mostly based on information from human medicine (Lacombe et al. Citation2001; Aleman et al. Citation2006; Williams et al. Citation2008).
The electrode placement in horses is currently based on human systems and researchers describe their materials and methods not substantiated and extensively, while leaving out the temporal lobes. In human medicine, most inter-ictal signals are found on the temporal lobe of the brain (Beleza et al. Citation2009; Noachtar and Rémi Citation2009; Tassi et al. Citation2009). The sensitivity might potentially increase if a system based on the anatomical location of the brain in the horse's head would be used (Empson Citation1986a; Klem et al. Citation1999). When electrodes are placed suboptimal on the top of musculature of ears or temporal muscle, every muscle contraction is recorded. Especially during wakefulness this interferes with the EEG by increased background activity, according to some authors (Williams et al. Citation2008).
The duration of the conventional EEG recordings used in equine medicine is not always described (Aleman et al. Citation2006) or described recordings vary from 15 minutes or more without specifying the duration (Lacombe et al. Citation2001; Williams et al. Citation2008). Performing EEG using a system in which a horse is attached to the computer necessitates sedation or continuous monitoring (Rose and Hodgson Citation2000; Lacombe et al. Citation2001; Aleman et al. Citation2006; Williams et al. Citation2008). In AEEG, sedatives and anaesthetics are unnecessary after electrode placement (van der Ree Citation2011), which is beneficial since drugs influence the signals. Long lasting recordings can be obtained while normal activities and sleep are present. In humane medicine, this proved to be helpful in more accurately diagnosing epilepsy independently of the presence of an actual seizure (Marsan and Zivin Citation1970; Ebersole Citation1987; De Lahunta Citation2001).
With electrode placement based on the horse's anatomy and suitable equipment in the future, AEEG might become commercially available to record brain signals in awake horses. If recordings can be coupled with simultaneous film records of the patients (Williams et al. Citation2008), the ideal situation might be achieved since details on behavior can be coupled with the EEG recordings (Beleza et al. Citation2009; Noachtar and Rémi Citation2009). AEEG recordings might benefit both horses and owners in understanding and diagnosing the cause of the abnormal behaviour of their horse.
Acknowledgments
References
- Aleman , M , Gray , LC , Williams , DC , Holliday , TA , Madigan , JE , LeCouteur , RA and Magdesian , KG . 2006 . Juvenile idiopathic epilepsy in egyptian arabian foals: 22 cases (1985–2005) . J Vet Intern Med , 20 ( 6 ) : 1443 – 1449 .
- Barlow , JS . 1993a . “ The waking EEG ” . In The electroencephalogram. Its patterns and origins , Edited by: Barlow , JS . 53 – 69 . London : MIT Press .
- Barlow , JS . 1993b . “ The normal EEG during sleep ” . In The electroencephalogram. Its patterns and origins , Edited by: Barlow , JS . 71 – 83 . London : MIT Press .
- Barlow , JS . 1993c . “ Pathophysiology of the EEG ” . In The electroencephalogram. Its patterns and origins , Edited by: Barlow , JS . 178 – 187 . London : MIT Press .
- Beleza , P , Bilgin , O and Noachtar , S . 2009 . Inter-ictal rhythmical midline theta differentiates frontal from temporal lobe epilepsies . Epilepsia , 50 ( 3 ) : 550 – 555 .
- Berger , H . 1929 . Über das elektrenkephalogramm des menschen . Archiv f Psychiatrie , 87 ( 1 ) : 527 – 570 .
- Bridgers , SL and Ebersole , JS . 1985 . The clinical utility of ambulatory cassette EEG . Neurology , 35 ( 2 ) : 166 – 173 .
- Capovilla , G , Beccaria , F , Bianchi , A , Canevini , MP , Giordano , L , Gobbi , G , Mastrangelo , M , Peruzzi , C , Pisano , T Striano , P . 2011 . Ictal EEG patterns in epilepsy with centro-temporal spikes . Brain Dev , 33 ( 4 ) : 301 – 309 .
- Colahan , TP , Mayhew , IG , Merrit , MA , Moore , NJ , King , C and McCann , EM . 1999 . “ Nervous system ” . In Manual of equine medicine and surgery , Edited by: Colahan , TP . 262 – 263 . Missouri : Mosby .
- De Lahunta , A . 2001 . “ Seizures-convulsions ” . In In: Veterinary neuroanatomy and clinical neurology , 2nd , Edited by: De Lahunta , A . 326 – 332 . Philadelphia : Saunders .
- Dement , W and Kleitman , N . 1957 . Cyclic variations in EEG during sleep and their relation to eye movements, body motility, and dreaming . Electroencephalogr Clin Neurophysiol , 9 ( 4 ) : 673 – 690 .
- Ebersole , JS . 1987 . Ambulatory cassette EEG in epilepsy diagnosis . Yale J Biol Med , 60 ( 2 ) : 85 – 91 .
- Empson , JAC . 1986a . “ The history and origin of the EEG ” . In Human brainwaves, the psychological significance of the electroencephalogram , Edited by: Empson , JAC . 5 – 10 . Hampshire : MacMillan .
- Empson , JAC . 1986b . “ Sleep and dreaming ” . In Human brainwaves, the psychological significance of the electroencephalogram , Edited by: Empson , JAC . 69 – 71 . Hampshire : MacMillan .
- Engel J, editor. 2005. Atlas of EEG Patterns. Philadelphia: Lippincott Williams & Wilkins
- Klem , GH , Lüders , HO , Jasper , HH and Elger , C . 1999 . The ten–twenty electrode system of the international federation: the international federation of clinical neurophysiology . Electroencephalogr Clin Neurophysiol Suppl , 52 : 3 – 6 .
- Lacombe , VA , Mayes , M , Mosseri , S , Reed , SM , Fenner , WR and Ou , HT . 2012 . Epilepsy in horses: aetiological classification and predictive factors . Equine Vet J , 44 ( 6 ) : 646 – 651 .
- Lacombe , VA , Podell , M , Furr , M , Reed , SM , Oglesbee , MJ , Hinchcliff , KW and Kohn , CW . 2001 . Diagnostic validity of electroencephalography in equine intracranial disorders . J Vet Intern Med , 15 ( 4 ) : 385 – 393 .
- Lewin , W and Tönhardt , H . 1998 . Non-invasive EEG-leading in the awake standing horse . Pferdeheilkunde , 14 ( 4 ) : 285 – 294 .
- Liasis , A , Bamiou , DE , Boyd , S and Towell , A . 2006 . Evidence for a neurophysiologic auditory deficit in children with benign epilepsy with centro-temporal spikes . J Neural Transm , 113 ( 7 ) : 939 – 949 .
- Loomis , AL , Harvey , EN and Hobart , G . 1936 . Electrical potentials of the human brain . J Exp Psychol , 19 ( 3 ) : 249 – 279 .
- Marsan , CA and Zivin , LS . 1970 . Factors related to the occurrence of typical paroxysmal abnormalities in the EEG records of epileptic patients . Epilepsia , 11 ( 4 ) : 361 – 381 .
- Mayhew , IG . 1989 . “ Chapter 6 seizures ” . In Large animal neurology: a handbook for veterinary clinicians , Edited by: Mayhew , IG . 113 – 125 . Philadelphia : Lea & Febiger .
- Mittel , L . 1987 . Seizures in the horse . Vet Clin North Am Equine Pract , 3 ( 2 ) : 323 – 332 .
- Mysinger , W , Redding , RW and Vaughan , JT . 1985 . Electroencephalographic patterns of clinically normal, sedated, and tranquilized newborn foals and adult horses . Am J Vet Res , 46 ( 1 ) : 36 – 41 .
- Noachtar , S and Rémi , J . 2009 . The role of EEG in epilepsy: a critical review . Epilepsy Behav , 15 ( 1 ) : 22 – 33 .
- Overvliet , GM , Besseling , RMH , Vles , JSH , Hofman , PAM , Backes , WH , van Hall , MHJA , Klinkenberg , S , Hendriksen , J and Aldenkamp , AP . 2010 . Nocturnal epileptiform EEG discharges, nocturnal epileptic seizures, and language impairments in children: review of the literature . Epilepsy Behav , 19 ( 4 ) : 550 – 558 .
- Patel K, Chua C-P, Faul S, Bleakley CJ. 2009. Low power real-time seizure detection for ambulatory EEG. 3rd International Conference on Pervasive Computing Technologies for Healthcare, PCTHealth 2009; 1–3 April 2009; London
- Priyadharsini , SS and Rajan , SE . 2012 . An efficient soft-computing technique for extraction of EEG signal from tainted EEG signal . Appl Soft Comput J , 12 ( 3 ) : 1131 – 1137 .
- Purohit , RC , Mysinger , PW and Redding , RW . 1981 . Effects of xylazine and ketamine hydrochloride on the electroencephalogram and the electrocardiogram in the horse . Am J Vet Res , 42 ( 4 ) : 615 – 619 .
- Reed , SM , Bayly , MW and Sellon , CD . 2004 . “ Seizures, narcolepsy and cataplexy ” . In Equine internal medicine , 2nd , Edited by: Reed , SM and Andrews , FM . 560 – 563 . Missouri : Saunders .
- Robinson , NE , Wilson , RM and Trogdon Hines , M . 2003 . “ Changes in mentation, seizures and narcolepsy ” . In Current therapy in equine medicine , 5th , Edited by: Robinson , NE . 764 – 771 . Missouri : Saunders .
- Rose , JR and Hodgson , RD . 2000 . “ Neurology ” . In Manual of equine practice , 2nd , Edited by: Rose , JR . 522 – 539 . Philadelphia : Saunders .
- Seneviratne , U , Cook , M and D'Souza , W . 2012 . The electroencephalogram of idiopathic generalized epilepsy . Epilepsia , 53 ( 2 ) : 234 – 248 .
- Sheridan , PH and Sato , S . 1985 . Applications of intensive monitoring in epilepsy . J Clin Neurophysiol , 2 ( 3 ) : 221 – 229 .
- Shih , EI , Shoeb , AH and Guttag , JV . 2009 . Sensor selection for energy-efficient ambulatory medical monitoring . 7th ACM International Conference on Mobile Systems, Applications and Services . 2009 . MobiSys’09; 22–25 June 2009; Krakow. 347pp
- Tassi , L , Meroni , A , Deleo , F , Villani , F , Mai , R , Russo , GL , Colombo , N , Avanzini , G , Falcone , C Bramerio , M . 2009 . Temporal lobe epilepsy: neuropathological and clinical correlations in 243 surgically treated patients . Epileptic Disord , 11 ( 4 ) : 281 – 292 .
- Tassinari , CA , Cantalupo , G , Rios-Pohl , L , Giustina , ED and Rubboli , G . 2009 . Encephalopathy with status epilepticus during slow sleep: ‘the penelope syndrome’ . Epilepsia , 50 ( Suppl. 7 ) : 4 – 8 .
- van der Ree M. 2011. AEEG used as a diagnostic tool for epilepsy in horses. European Veterinary Conference Voorjaarsdagen; 27 April; Amsterdam, the Netherlands. 370pp
- Walter , WG . 1936 . The location of cerebral tumours by electro-encephalography . Lancet , 228 ( 5893 ) : 305 – 308 .
- Waterhouse , E . 2003 . New horizons in ambulatory electroencephalography . IEEE Eng Med Biol Mag , 22 ( 3 ) : 74 – 80 .
- Williams , DC , Aleman , M , Tharp , B , Fletcher , DJ , Kass , PH , Steffey , EP , Lecouteur , RA and Holliday , TA . 2012 . Qualitative and quantitative characteristics of the electroencephalogram in normal horses after sedation . J Vet Intern Med , 26 ( 3 ) : 645 – 653 .
- Williams , DC , Aleman , M , Holliday , TA , Fletcher , DJ , Tharp , B , Kass , PH , Steffey , EP and LeCouteur , RA . 2008 . Qualitative and quantitative characteristics of the electroencephalogram in normal horses during spontaneous drowsiness and sleep . J Vet Intern Med , 22 ( 3 ) : 630 – 638 .