Abstract
This paper deals with electrochemical properties of copper in the presence of the non-toxic compound 2-amino- 5-ethyl- 1,3,4-thiadiazole (AETDA) in acidic chloride solution. Electrochemical techniques: open circuit potential measurements, linear potentiodynamic measurements, cyclic voltammetry measurements and chronoamperometric measurements in addition to SEM–EDS analysis were used during the work. Results show that inhibition efficiency of 2-amino-5-ethyl-1,3,4-thiadiazole depends on inhibitor concentration and immersion time of copper electrode in inhibitor solution. Cyclic voltammetry and linear potentiodynamic measurements beside chronoamperometric measurements indicate formation of protective layer on copper surface. Moreover, results obtained by electrochemical measurements point out that the stability of formed layer depends primarily on the concentration of inhibitor and of potential values at which protective film is formed. Also, potentiodynamic measurements show that AETDA in acidic chloride solutions acts as mixed-type inhibitor. Inhibition mechanism of 2-amino-5-ethyl-1,3,4-thiadiazole includes adsorption of inhibitor on active sites on electrode surface which was confirmed by SEM–EDS analysis of the electrode surface. Adsorption of the AETDA in HCl solution obeys Langmuir adsorption isotherm.
1. Introduction
Copper is one of the most important metals that is used in many industries due to its good electrical and thermal conductivity and relatively good stability in different environments.[Citation1–5] Nowadays, copper with its alloys is widely used in the electronics industry as a conductor, as well as in the preparation of parts for heat exchangers and cooling systems. Further, copper is widely used in information technology and microelectronics industry.[Citation1,2,6] However, numerous literature data indicate that under certain conditions copper may become susceptible to corrosion.[Citation4,7–11] This is especially evident in chloride solutions, so the inhibition of corrosion in such environments was under examination in some earlier works.[Citation1,3,7,12] A special focus was on examination of the structure and electrochemical properties of the film formed on the copper surface, its stability, solubility and inhibition efficiency (IE).[Citation1,13] Influence of temperature and pH values of solutions on inhibition action of examined compounds was intensively investigated.[Citation7,14] Further, the effect of the inhibitor concentration and duration of pretreatment in inhibitor solution on electrochemical dissolution of copper and its alloys was examined.[Citation1–3,6,8,15–18] According to literature data copper corrosion testing was performed in a variety of environments such as: artificial seawater,[Citation6,19,20] NaCl,[Citation1,2,7,9,21] acetate,[Citation16] chloride,[Citation22–25] sulphate[Citation10,11,26,27] and nitrate solutions.[Citation28,29] Products which are formed by dissolving the metal may pose a threat to the environment, so the corrosion prevention has extreme importance. Use of organic compounds which contain hetero atoms (N, S, O) is one of the most efficient and economical ways to achieve efficient corrosion protection. Existence of hetero atoms in the structure of inhibitor’s molecule is one of the main precondition for the inhibitory action.[Citation30–32]
Compounds with hetero atoms have a strong affinity for copper. It contributes to good inhibitory effect which is usually attributed to their interactions with the surface of the copper through adsorption.[Citation1–4,6,8,10,11,16,29,33–38] The polar functional groups that are included in the composition of the inhibitors may be considered as centres of the reaction process which stabilizes the adsorption.[Citation1,2] However, organic compounds that are toxic and hazardous were often used as inhibitors.[Citation8,11,17,18,28,29,33,39] Therefore, in the last few years, non-toxic and biodegradable compounds are tested intensively as potentially good corrosion inhibitors.[Citation10,11,28,29,34,40]
Azole derivatives such as: benzotriazole, mercaptobenzotriazole, benzimidazole, thiadiazole and imidazole have been carefully examined as copper corrosion inhibitors over the years.[Citation1–4,12,15,24–26,37,41] These compounds contain one or more nitrogen atoms in their structure. Nitrogen atom with lone electrone pairs facilitates the adsorption of molecules of inhibitor and formation of protective film on the copper surface. According to the literature data, the efficiency of azole compounds as copper corrosion inhibitors is correlated with the semiconductor characteristics of the copper (I) oxide that is formed in the tested conditions.[Citation1,2]
In this paper, inhibitory effect of 2-amino-5-ethyl-1,3,4-thiadiazole (AETDA) on the copper corrosion in a 0.05-M HCl solution (pH ~ 2) was examined. During the work, primarily, impact of AETDA concentrations on decrease of corrosion processes in the hydrochloric acid solutions was followed. Moreover, pretreatment of copper electrode in AETDA solution for different time periods was performed in order to obtain more complete insight into the inhibition activity of AETDA. 2-amino-5-ethyl-1,3,4-thiadiazole was chosen as a potentially good inhibitor due to the existence of heteroatoms (N and S) in the structure of the molecule (Figure ). Besides, AETDA is non-toxic and inexpensive compound.[Citation1]
2. Experimental
2.1. Electrochemical measurements
Electrochemical measurements were performed using a potentiostat (IVIUMSTAT XRe, IVIUM Technologies) with supporting software. Working copper electrode was prepared by the method of casting upward and it was sealed in a mixture based on methyl methacrylate. Electrochemical measurements were performed in a three-electrode system where working electrode was already described as copper electrode, saturated calomel electrode (SCE) was the reference electrode and the platinum was auxiliary electrode. Prior to each measurement, copper electrode was polished with SiC paper (800 and 2000) and alumina paste (0.3 μm Al2O3, Buehler USA) then rinsed with distilled water and dried afterwards. Electrochemical methods applied during the experimental work were: open circuit potential (OCP) measurements during 10 min, linear potentiodynamic measurements, cyclic voltammetry and chronoamperometry. Cyclic voltammetry were carried out from –1.0 to 1.2 V vs. SCE, whereas linear potentiodynamic measurements were performed from OCP to −0.5 V vs. SCE in cathodic and to 0.2 V vs. SCE in anodic direction. Scan rate during linear potentiodynamic measurements was 1 and 10 mV/s during cyclic voltammetry. Chronoamperometric measurements were conducted at two different potentials.
The following compounds were used in preparation of the solution: hydrochloric acid (HCl, Zorka Pharmacy Šabac), 2-amino-5-ethyl-1,3,4-thiadiazole (Sigma Aldrich Germany) all of p.a. purity. Stock solution of 2-amino-5-ethyl-1,3,4-thiadiazole was 1 ∙ 10−2 M, while the set of working solutions (1 ∙ 10−3 – 1 ∙ 10−6 M) was obtained by diluting the stock solution.
Electrochemical behaviour of copper and inhibitory action of AETDA in hydrochloric acid solutions were tested as follows:
• | Cyclic voltammetry was carried out in 0.05-M HCl in the presence of different concentrations of inhibitor (1 · 10−6 – 1 · 10−2 M). | ||||
• | Linear potentiodynamic measurements were performed after OCP measurements in 0.05-M HCl in the presence of various concentrations of inhibitor (1 · 10−6 – 1 · 10−2 M). | ||||
• | Electrode was immersed for a certain time (5, 15, 30 and 60 min) in 1 · 10−2 M AETDA, then rinsed with distilled water and carried into the hydrochloric acid solution, wherein cyclic voltammetry was carried out. | ||||
• | Electrode was immersed for various periods of time (5, 15, 30 and 60 min) in 1 · 10−2 M AETDA solutions. Afterwards, copper electrode was rinsed with distilled water and carried into 0.05-M HCl solution in which, after measuring the OCP, cathodic and anodic linear potentiodynamic measurements were performed. | ||||
• | Chronoamperometric measurements were performed at potentials 0 V vs. SCE and 50 mV vs. SCE for a period of 45 min in 0.05-M HCl solution without and with an addition of various concentrations of inhibitor (1 · 10−6 – 1 · 10−2 M). |
All measurements were done in naturally aerated solutions at ambient temperature. Also, all measurements were repeated at least three times and presented curves represent the mean value of the measurements.
2.2. Surface analysis
SEM–EDS method was used to examine the surface morphology of the copper after sample polishing without any treatment and after immersion of Cu sample in 1 · 10−2 M 2-amino-5-ethyl-1,3,4-thiadiazole solution for 60 min. The SEM/EDS experiments were performed on Tescan VEGA 3 LM scanning electron microscope equipped with Oxford EDS X-act Inca 350 system.
3. Results and discussion
3.1. OCP measurements of copper in hydrochloric acid solution
OCP measurements were done for 10 min in 0.05-M HCl solutions with and without the presence of various concentrations of AETDA. The obtained average values for OCP are shown in Figure .
Figure 2. OCP of copper in 0.05-M HCl solution without and with the addition of different concentration of AETDA.
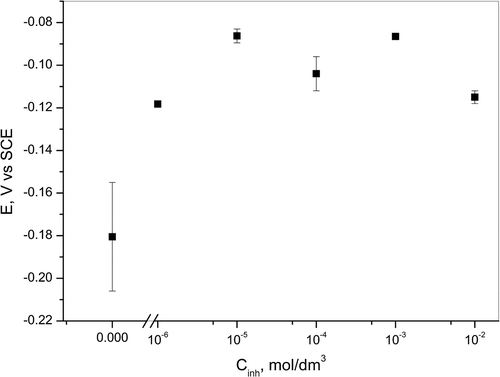
According to data presented in Figure , OCP values shift toward more positive direction in the presence of inhibitor, which may be associated with the adsorption of AETDA on the copper surface.[Citation42,43] Also, CuCl formation on metal surface in chloride-containing solutions and formation of Cu at the CuCl layer/electrolyte interface increase OCP values. According to literature data, CuCl diminishes surface coverage and is not able to protect the copper surface due to the active equilibrium between CuCl formation and dissolution through the formation of cuprous chloride complex.[Citation44]
In cases when copper electrode was immersed for certain times (5, 15, 30 and 60 min) in 1 ∙ 10−2 M solution of 2-amino-5-ethyl-1,3,4-thiadiazole OCP shifts to the more positive values with the increase in immersion time (Figure ), which also can be associated with adsorption of inhibitor on electrode surface. Changes of values of OCP indicate that AETDA acts like mixed-type inhibitor in acidic chloride solution after pretreatment in inhibitor solution, which will be discussed later. It is possible that displacement of OCP to more positive values is connected with the formation of a protective layer on copper surface that decreases cathodic and anodic reactions rate, which will also be discussed later.
3.2. Cyclic voltammetry in acidic chloride solutions
Cyclic voltammogram of copper obtained in a hydrochloric acid solutions without and with the addition of 2-amino-5-ethyl-1,3,4-thiadiazole is shown in Figure . According to CV data in 0.05-M HCl with and without different amount of AETDA first comes to Cu oxidation to Cu+ ions. Further, formed Cu+ ions oxidize to the Cu2+ ions and are indicated by the anodic current peak at 0.1 V vs. SCE. Copper dissolution in naturally aerated acidic chloride solutions may be interpreted by the following equations [Citation34]:
Figure 4. Cyclic voltammogram of copper recorded in 0.05-M HCl in the presence of various concentrations of 2-amino-5-ethyl-1,3,4-thiadiazole. Scan rate 10 mV/s.
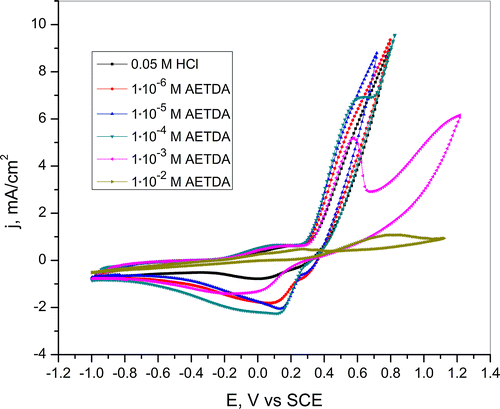
(1)
(2)
Increase in Cu2+ concentration according to previous reactions causes further dissolution of copper which is shown by the following equation:
(3)
Moreover, the presence of chloride ions in the examined solutions leads to the formation of CuCl species which are unstable in an acidic environment. Nonetheless, CuCl species in the presence of Cu+ ions easily converted into soluble complex that is indicated by a sharp increase in current density on CV curves. It can be shown by the following reactions [Citation41,45]:
(4)
(5)
(6)
In reverse sweep, the reduction peak corresponds to the reduction of soluble complex and CuCl layer on copper surface.[Citation46,47] Decrease of reduction peak intensity in the presence of inhibitor indicates the inhibitory effect of the 2-amino-5-ethyl-1,3,4-thiadiazole. Nevertheless, the partial dissolution of copper occurs in hydrochloric acid solutions in the presence of various amounts of inhibitor. However, according to polarization curves presented in Figure , in HCl solution with the addition of 1 ∙ 10–2 M AETDA copper dissolution significantly decreases. This occurs due to the formation of protective layer on the electrode surface which effectively prevents intense copper dissolution. The inhibition mechanism of azole compounds can be explained by the following reactions: [Citation41]
(7)
(8)
A formed Cu(inh)ads does not cover entire surface of the copper due to lower concentration of the inhibitor. Therefore, the dissolution of metals occurs on places on the copper surface that are not covered with Cu(inh)ads layer. Also, the decrease in current density was observed in the whole potential region which may be associated with reducing of the possibilities of Cl− ions to react with Cu on the electrode surface and formation of copper corrosion products due to inhibitor adsorption.[Citation41,48]
Cyclic voltammograms of copper obtained in 0.05-M HCl solution after pretreatment of Cu electrode for different periods of time (5, 15, 30 and 60 min) in 1 · 10−2 M AETDA solution are presented in Figure .
Figure 5. Cyclic voltammogram of copper in a 0.05-M HCl solution after immersion in 1 · 10−2 M AETDA solution for various periods of time (5, 15, 30 and 60 min). Scan rate 10 mV/s.
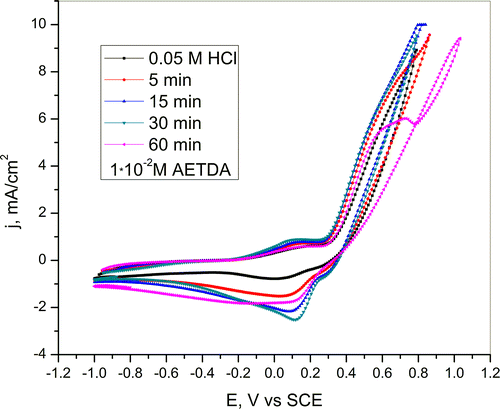
On the basis of CV curves obtained in 0.05-M HCl solution with the addition of inhibitor (Figure ) and after immersion in inhibitor solution for various periods of time (5, 15, 30 and 60 min) (Figure ) it can be seen that mechanism of copper dissolution remains unchanged. The only significant difference in the mechanism of copper dissolution, according to polarization curve was obtained in HCl solution after pretreatment of copper electrode in duration of 60 min in inhibitor solution, is the appearance of repassivation potential at 0.78 V vs. SCE.
In addition to the anodic corrosion processes in the observed system cathodic corrosion process occurs. It can be attributed to the following equation [Citation41,49]:
(9)
3.3. Linear potentiodynamic measurements in 0.05-M HCl solutions
Besides the cyclic voltammetry, for a complete understanding of corrosion processes and mechanisms of action of 2-amino-5-ethyl-1,3,4-thiadiazole linear potentiodynamic measurements were performed. Figure shows polarization curves of copper in hydrochloric acid solution in the presence of various concentrations of AETDA where it can be seen that the addition of the inhibitor diminishes cathodic and anodic corrosion processes. In the presence of inhibitor, corrosion potential shifts to more positive values maintaining similar values regardless of the change in the concentration of inhibitor. Presence of 2-amino-5-ethyl-1,3,4-thiadiazole significantly affects the decrease in anodic current density compared to the decrease in cathodic current density which indicates that AETDA acts like mixed-type inhibitor with a pronounced influence on the anodic corrosion processes in acidic chloride solutions. Decrease of current density in the presence of inhibitor may indicate formation of a protective layer that was also noticed during cyclic potentiodynamic measurements. Also, linear potentiodynamic measurements confirmed that the stability of the protective film increases with increasing AETDA concentration. Nonetheless, during the polarization measurements in the HCl solution with the addition of different amounts of inhibitor defects appear in protective layer structure, which leads to further dissolution of copper. Also dissolution of the protective film is unavoidable in the acidic chloride solution.[Citation50] It gives the possibility that aggressive ions get in contact with copper surface and cause further dissolution of metal.
Figure 6. Polarization curves for copper in a 0.05-M HCl solution in the presence of various concentrations of 2-amino-5-ethyl-1,3,4-thiadiazole. Scan rate 1 mV/s.
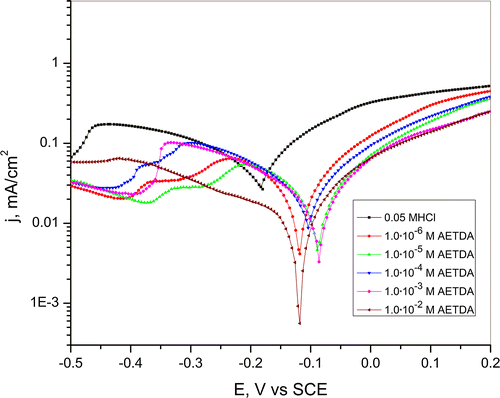
Polarization curves for copper obtained in hydrochloric acid solution with previous immersion of copper electrode for various periods of time (5, 15, 30 and 60 min) in 1 · 10−2 M AETDA solution are shown in Figure . Corrosion potential obtained in HCl solution, after copper pretreatment in inhibitor solution, shifts to more positive values with regard to corrosion potential obtained in HCl solution without immersion of copper electrode in AETDA solution. During pretreatment in inhibitor solution comes to adsorption of inhibitor molecules on cathodic and anodic active sites on copper surface. Adsorption of inhibitor molecules leads to inhibition of cathodic and anodic corrosion processes in hydrochloric acid solution. According to that, it can be noticed that 2-amino-5-ethyl-1,3,4-thiadiazole acts like mixed-type inhibitor with a pronounced influence on the anodic corrosion processes. Electrochemical parameters of copper oxidation in the 0.05-M HCl solution in the presence of various concentration of AETDA and after immersion in AETDA solution were calculated on the basis of linear potentiodynamic curves and obtained values for corrosion potential (Ecorr), corrosion current density jcorr, as well as the anodic (ba) and cathodic (bc) Tafel slopes are shown in Table . Values of IE and degree of coverage (θ) are also presented in Table in addition to the electrochemical parameters. The IE is calculated according to the following equation:
Figure 7. Polarization curves for copper in 0.05-M HCl solution after immersion in 1 · 10−2 M AETDA solution for various periods of time (5, 15, 30 and 60 min). Scan rate 1 mV/s.
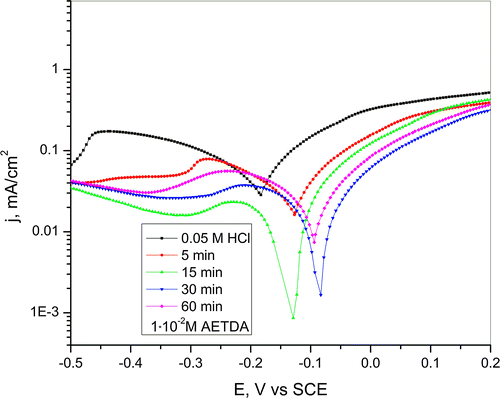
Table 1. Electrochemical parameters of copper in 0.05-M HCl in the presence of various concentration of inhibitor and after immersion in 1 · 10−2 M AETDA.
(10)
where jcorr and jinh represent corrosion current density in the absence and presence of the inhibitor. It is clear from Table that the IE increases with increasing concentration of 2-amino-5-ethyl-1,3,4-thiadiazole. Moreover, pretreatment of copper electrode in AETDA solution also had strong influence on increase of IE. IE increases with the increase in immersion time of copper electrode in inhibitor solution up to 30 min reaching higher values than in the case when the electrode was not previously exposed to the inhibitor solution.
Degree of coverage can be calculated according to equation:
(11)
Table contains values of the surface coverage for different inhibitor concentrations and after copper treatment in AETDA solution, which were determined from polarization curves present in Figures and .
Changes in values of Tafel slope in the presence of various concentration of inhibitor in regards to bare hydrochloric acid solution indicate formation of the protective film on electrode surface in the presence of AETDA.[Citation1–3,12,51] After the electrode was immersed in AETDA solution for certain period of time (15, 30 and 60 min), the anodic Tafel slope (ba) remained almost constant indicating that there is no modification in anodic dissolution process of copper due to the adsorption of inhibitor molecules.[Citation41]
According to values of degree of coverage, increase in pretreatment duration leads to saturation of the electrode surface with inhibitor molecules. Also, results indicate that maximum saturation was achieved after immersion period of 30 min. Moreover, stability of formed film increases with increasing exposure time up to 30 min of copper electrode in inhibitor solution. Further the increase in pretreatment period up to 60 min leads to a slight decrease in inhibition efficiencies which is attributed to dissolution of the adsorbed film after immersion of copper electrode in hydrochloric acid solution. Also, the decrease in IE may be caused by the ‘steric effect’, when the number of adsorbed molecules reaches a certain value and starts to rearrange on the surface which makes it easy for the chloride ions to attack the electrode through the interspaces.[Citation36,52] These results also show that in acidic chloride solutions besides selection of the optimum concentration of inhibitor, good results in the inhibition of copper corrosion can be achieved by proper selection of the immersion time of electrode in inhibitor solution.
3.4. Chronoamperometric measurements in 0.05-M HCl solutions in the presence of AETDA
Potentiostatic polarization measurements were carried out for 45 min in HCl solution with and without the addition of inhibitor at different potential values (0 and 50 mV) and obtained curves are shown in Figures and .
Figure 8. Chronoamperometric curves for copper recorded at 0 V vs. SCE in a 0.05-M HCl solution in the presence of various concentrations of 2-amino-5-ethyl-1,3,4-thiadiazole.
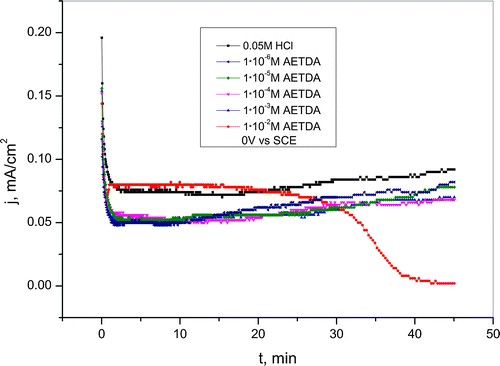
Figure 9. Chronoamperometric curves for copper recorded at 50 mV vs. SCE in a 0.05-M HCl solution in the presence of various concentrations of 2-amino-5-ethyl-1,3,4-thiadiazole.
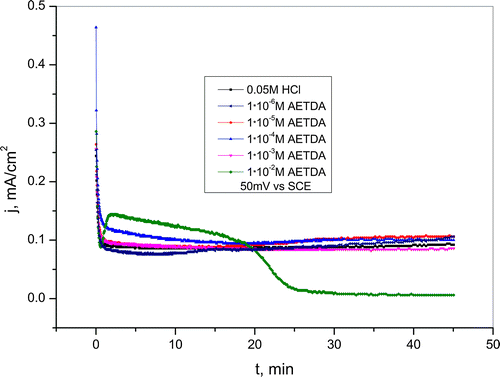
From Figure , it can be seen that in examined solutions at 0 V comes to monotonic decrease in current density values in bare hydrochloric acid solution as well as in HCl solutions with the addition of AETDA in a wide concentration range (1 · 10−6 – 1 · 10−3 M). Current density decrease in HCl solution without addition of inhibitor indicates formation of copper corrosion products which are in accordance with the results obtained by potentiodynamic measurements. Also, current density decrease is more pronounced in the presence of 2-amino-5-ethyl-1,3,4-thiadiazole compared to the current density decrease in the bare HCl solution. It points to adsorption of the AETDA molecules on the copper surface and the formation of the protective layer.[Citation53,54] Further, during chronoamperometric measurements, current density slightly increase which is related to desorption of inhibitor and dissolution of protective layer. On the active sites on copper surface after desorption of AETDA molecules surface becomes stripped and available to aggressive ions which cause further oxidation of copper.
Moreover, during potentiostatic polarization measurements at 50 mV in 0.05-M HCl solution, the addition of inhibitor in concentration range from 1 · 10−6 to 1 · 10−4 M causes a slight increase in current density which points to the activation effect of 2-amino-5-ethyl-1,3,4-thiadiazole (Figure ).
In the presence of inhibitor in an amount of 1 · 10−2 M initially came to copper dissolution at 0 V as well as at 50 mV vs. SCE. Further, during chronoamperometric measurements, current density sharply and significantly decrease due to the formation of a stable protective layer. Current density decrease depends on the applied potential and occurs earlier at the potential of 50 mV vs. SCE. This indicates that at the potential of 50 mV, protective layer was formed faster than at lower potential, whereby the higher stability of the protective film is achieved if the film is formed on the 0 V vs. SCE. Chronoamperometric curves present in Figures and indicate that in addition to potential, also concentration of inhibitor has significant role in the formation of protective layer on copper surface. Besides that, the presence of copper and chloride ions which can be built into the structure of the film and create more compact layer also significantly contributes to increase the stability of the protective film. Current density values in HCl solutions without and with the addition of 2-amino-5-ethyl-1,3,4-thiadiazole at examined potentials were shown in Table ; and it can be seen that when concentration of inhibitor increases, percentage of reduction in current density also increases. The percentage of reduction in current density can also be thought of as the IE.[Citation55]
Table 2. Current density and percentage of reduction in current density in a 0.05-M HCl solution without and with the addition of AETDA at 0 and 50 mV vs. SCE.
3.5. Surface analysis
According to Figure , it can be seen that after pretreatment in 2-amino-5-ethyl-1,3,4-thiadiazole electrode surface is changed. Moreover, on the copper surface which is present in Figure (B) exists adsorbed species that are not found on clean copper surface (Figure (A)). EDS spectrograms show that sulphur atoms are detected on copper surface after treatment of electrode in AETDA solution. Furthermore, sulphur atoms are derived from inhibitor molecules and their presence indicates that adsorption of the AETDA occurs on the active sites on the copper surface.
3.6. Adsorption isotherms
Adsorption of inhibitor on metal surface is the first and one of the most important steps in the mechanism of corrosion inhibition. The inhibitory effect may be connected with physical adsorption or chemisorption of inhibitor.[Citation11,17] Metal surface must be electrically charged in physical adsorption process and charged species should be present in the bulk of the solution.[Citation56] Nonetheless, chemical adsorption requires existence of vacant low-energy electron orbital in metal structure and inhibitors with relatively loosely bonded electrons in molecules or heteroatom with lone pair electrons.[Citation56] Besides, adsorption of inhibitor depends on several factors, such as the nature of the metal surface, the distribution of charge in the inhibitor molecule, the chemical structure of the inhibitor and the type of interaction between inhibitor and metal surface.[Citation56–59] Furthermore, it can be said that the adsorption isotherms provide useful information regarding the nature of the interaction between inhibitor molecules and metal. The mechanism of adsorption of 2-amino-5-ethyl-1,3,4-thiadiazole on copper surface was examined using a number of different adsorption isotherms (Table ).
Table 3. List of adsorption isotherms.
Figure shows adsorption isotherms applied for the investigated system. Adsorption isotherms curves and values of factor of regression (R2) for each depicted isotherm presented in Table indicate that the Langmuir isotherm best describes process of adsorption of the inhibitor molecules on the copper surface.
Table 4. Langmuir, Freundlich, Temkin, Flory-Huggins and Dubinin–Radushkevich adsorption parameters and free energy of adsorption of AETD.
Adsorption parameters calculated according to the Langmuir adsorption isotherm indicate that at one active site on the electrode surface one molecule of inhibitor is adsorbed.[Citation61] Adsorption of 2-amino-5-ethyl-1,3,4-thiadiazole in the hydrochloric acid solution can be regarded as substitution process between the inhibitor in the aqueous phase and water molecules on the copper surface:
(12)
Gibbs free energy of adsorption is calculated using the following equation:
(13)
where K is the constant of the adsorption process (dm3/mol), ΔG is the adsorption energy (J/mol), R is the universal gas constant (J/K·mol) and T is the thermodynamic temperature (295 K). Gibbs free energy of adsorption calculated according to the above equation for Langmuir adsorption isotherm indicates strong adsorption of inhibitor on copper surface.[Citation11,56]
4. Conclusion
In this work, the corrosion and corrosion inhibition of copper in 0.05-M HCl solutions by 2-amino-5-ethyl-1,3,4-thiadiazole has been reported. According to experimental results obtained during OCP measurements, potentiodynamic and chronoamperometric polarization measurements following conclusions can be obtained:
Shift of OCP values in hydrochloric acid solution with and without the presence of AETDA indicates adsorption of inhibitor molecules on copper surface.
Immersion of copper electrode in AETDA solution also leads to adsorption of 2-amino-5-ethyl-1,3,4-thiadiazole and formation of protective layer on metal surface that become more compact and stable with increasing immersion time.
Cyclic voltammetry and linear potentiodynamic measurements also indicate formation of protective layer on copper surface. Stability of protective layer increases with increasing inhibitor concentration in HCl solution and increasing duration of pretreatment of electrode in AETDA solution. Potentiodynamic measurements show that the highest IE was achieved after 30 min immersion of copper electrode in AETDA solution. Longer exposure of the electrode in the 2-amino-5-ethyl-1,3,4-thiadiazole solution leads to a slight decrease in the inhibition efficiencies which is attributed to the dissolution of the adsorbed film on copper surface. Moreover, potentiodynamic measurements have shown that AETDA in acidic chloride solutions acts as mixed-type inhibitor.
Chronoamperometric measurements have shown that at examined potentials in the presence of inhibitor protective layer formed on copper surface. Nonetheless, the stability of formed layer depends primarily on the concentration of the inhibitor but also on potential values at which the protective film is formed.
Adsorption of 2-amino-5-ethyl-1,3,4-thiadiazole on copper surface in hydrochloric acid solution obeys the Langmuir adsorption isotherm.
Disclosure statement
No potential conflict of interest was reported by the authors.
Funding
This work was supported by the Ministry of Education and Science of Republic of Serbia through the [Project Number 172031].
References
- Sherif EM, Park SM. 2-Amino-5-ethyl-1,3,4-thiadiazole as a corrosion inhibitor for copper in 3.0% NaCl solutions. Corros. Sci. 2006;48:4065–4079.10.1016/j.corsci.2006.03.011
- Sherif EM. Effects of 2-amino-5-(ethylthio)-1,3,4-thiadiazole on copper corrosion as a corrosion inhibitor in 3% NaCl solutions. Appl. Surf. Sci. 2006;252:8615–8623.10.1016/j.apsusc.2005.11.082
- Sherif EM, Park SM. Effects of 2-amino-5-ethylthio-1,3,4-thiadiazole on copper corrosion as a corrosion inhibitor in aerated acidic pickling solutions. Electrochim. Acta. 2006;51:6556–6562.10.1016/j.electacta.2006.04.047
- Milić SM, Antonijević MM. Some aspects of copper corrosion in presence of benzotriazole and chloride ions. Corros. Sci. 2009;51:28–34.10.1016/j.corsci.2008.10.007
- Milić SM, Antonijević MM, Šerbula SM, et al. Influence of benzotriazole on corrosion behaviour of CuAlNiSi alloy in alkaline medium. Corros. Eng. Sci. Technol. 2008;43:30–37.10.1179/174327808X286329
- Sherif EM, Erasmus RM, Comins JD. Corrosion of copper in aerated synthetic sea water solutions and its inhibition by 3-amino-1,2,4-triazole. J. Colloid Interface Sci. 2007;309:470–477.10.1016/j.jcis.2007.01.003
- Hazzazi OA. Corrosion inhibition studies of copper in highly concentrated NaCl solutions. J. Appl. Electrochem. 2007;37:933–940.10.1007/s10800-007-9332-2
- Simonović AT, Petrović MB, Radovanović MB, et al. Inhibition of copper corrosion in acidic sulphate media by eco-friendly amino acid compound. Chem. Pap. 2014;68:362–371.
- Khaled KF. Studies of the corrosion inhibition of copper in sodium chloride solutions using chemical and electrochemical measurements. Mater. Chem. Phys. 2011;125:427–433.10.1016/j.matchemphys.2010.10.037
- Petrović MB, Simonović AT, Radovanović MB, et al. Influence of purine on copper behavior in neutral and alkaline sulfate solutions. Chem. Pap. 2012;66:664–676.
- Petrović MB, Radovanović MB, Simonović AT, et al. The effect of cysteine on the behaviour of copper in neutral and alkaline sulphate solutions. Int. J. Electrochem. Sci. 2012;7:9043–9057.
- Antonijević MM, Milić SM, Šerbula SM, et al. The influence of chloride ions and benzotriazole on the corrosion behavior of Cu37Zn brass in alkaline medium. Electrochim. Acta. 2005;50:3693–3701.10.1016/j.electacta.2005.01.023
- Antonijević MM, Bogdanović GD, Radovanović MB, et al. Influence of pH and chloride ions on electrochemical behavior of brass in alkaline solution. Int. J. Electrochem. Sci. 2009;4:654–661.
- Strehblow H, Maurice V, Marcus P. Initial and later stages of anodic oxide formation on Cu, chemical aspects, structure and electronic properties. Electrochim. Acta. 2001;46:3755–3766.10.1016/S0013-4686(01)00657-0
- Rafiquee MZA, Khan S, Saxena N, et al. Influence of some thiadiazole derivatives on corrosion inhibition of mild steel in formic and acetic acid media. Portugaliae Electrochim. Acta. 2007;25:419–434.10.4152/pea.200704419
- Antonijevic M, MPetrovic M. Copper corrosion inhibitors. A review. Int. J. Electrochem. Sci. 2008;3:1-28
- Radovanović MB, Petrović MB, Simonović AT, et al. Cysteine as a green corrosion inhibitor for Cu37Zn brass in neutral and weakly alkaline sulphate solutions. Environ. Sci. Pollut. Res. 2013;20:4370–4381.10.1007/s11356-012-1088-5
- Radovanović MB, Simonović AT, Petrović MB, et al. Influence of purine on brass behavior in neutral and alkaline sulphate solutions. Int. J. Electrochem. Sci. 2012;7:11796–11810.
- Huh JH, Kim SH, Chu JH, et al. Enhancement of seawater corrosion resistance in copper using acetone-derived graphene coating. Nanoscale. 2014;6:4379–4386.10.1039/C3NR05997A
- Núñez L, Reguera E, Corvo F, et al. Corrosion of copper in seawater and its aerosols in a tropical island. Corros. Sci. 2005;47:461–484.10.1016/j.corsci.2004.05.015
- Li Z, Jin L, Wang W, et al. Inhibition of the corrosion of copper in a sodium chloride solution using 4-((4-dodecylphenylimino)methyl)phenol. Int. J. Electrochem. Sci. 2013;8:6513–6523.
- Warraky AE, Shayeb HAE, Sherif EM. Pitting corrosion of copper in chloride solutions. Anti-Corros. Methods Mater. 2004;51:52–61.10.1108/00035590410512735
- Finšgara M, Merlb DK. 2-Mercaptobenzoxazole as a copper corrosion inhibitor in chloride solution: electrochemistry, 3D-profilometry, and XPS surface analysis. Corros. Sci. 2014;80:82–95.
- Zhang DQ, Gao LX, Zhou GD. Inhibition of copper corrosion in aerated hydrochloric acid solution by heterocyclic compounds containing a mercapto group. Corros. Sci. 2004;46:3031–3040.10.1016/j.corsci.2004.04.012
- Zhou GD, Hua S, Loo BH. A study of the copper electrode behavior in borax buffer solutions containing chloride ions and benzotriazole-type inhibitors by voltammetry and the photocurrent response method. J. Electroanal. Chem. 1997;421:129–135.10.1016/S0022-0728(96)04838-3
- Tantavichet N, Pritzker M. Copper electrodeposition in sulphate solutions in the presence of benzotriazole. J. Appl. Electrochem. 2006;36:49–61.10.1007/s10800-005-9000-3
- Zelinsky AG, Pirogov BY, Yurijev OA. Open circuit potential transients and electrochemical quartz crystal microgravimetry measurements of dissolution of copper in acidic sulfate solutions. Corros. Sci. 2004;46:1083–1093.10.1016/j.corsci.2003.09.008
- Scendo M. Inhibition of copper corrosion in sodium nitrate solutions with nontoxic inhibitors. Corros. Sci. 2008;50:1584–1592.10.1016/j.corsci.2008.02.015
- Khaled KF. Corrosion control of copper in nitric acid solutions using some amino acids – a combined experimental and theoretical study. Corros. Sci. 2010;52:3225–3234.10.1016/j.corsci.2010.05.039
- Tang Y, Yang X, Yang W, et al. Experimental and molecular dynamics studies on corrosion inhibition of mild steel by 2-amino-5-phenyl-1,3,4-thiadiazole. Corros. Sci. 2010;52:242–249.10.1016/j.corsci.2009.09.010
- Levin M, Wiklund P, Leygraf C. Bioorganic compounds as copper corrosion inhibitors in hydrocarbon media. Corros. Sci. 2012;58:104–114.10.1016/j.corsci.2012.01.009
- Wang YH, He JB. Corrosion inhibition of copper by sodium phytate in NaOH solution: cyclic voltabsorptometry for in situ monitoring of soluble corrosion products. Electrochim. Acta. 2012;66:45–51.10.1016/j.electacta.2012.01.063
- Ismail KM. Evaluation of cysteine as environmentally friendly corrosion inhibitor for copper in neutral and acidic chloride solutions. Electrochim. Acta. 2007;52:7811–7819.10.1016/j.electacta.2007.02.053
- Scendo M. Corrosion inhibition of copper by purine or adenine in sulphate solutions. Corros. Sci. 2007;49:3953–3968.10.1016/j.corsci.2007.03.037
- Ucán SLC, Atoche AC, Borges PC, et al. Inhibition effect of glycerol on the corrosion of copper in NaCl solutions at different pH values. J. Chem. 2014;2014:1–10.10.1155/2014/396405
- Hukovič MM, Babić R, Paić I. Copper corrosion at various pH values with and without the inhibitor. J. Appl. Electrochem. 2000;30:617–624.10.1023/A:1003956102631
- Subramanian R, Lakshminarayanan V. Effect of adsorption of some azoles on copper passivation in alkaline medium. Corros. Sci. 2002;44:535–554.10.1016/S0010-938X(01)00085-3
- Qin TT, Li J, Luo HQ, et al. Corrosion inhibition of copper by 2,5-dimercapto-1,3,4-thiadiazole monolayer in acidic solution. Corros. Sci. 2011;53:1072–1078.10.1016/j.corsci.2010.12.002
- Abelev E, Starosvetsky D, Eli YE. Potassium sorbate-a new aqueous copper corrosion inhibitor. Electrochim. Acta. 2007;52:1975–1982.10.1016/j.electacta.2006.08.012
- Petrović Mihajlović MB, Antonijević MM. Copper corrosion inhibitors. Period 2008–2014. A review. Int. J. Electrochem. Sci. 2015; 10:1027–1053.
- Sudheer, Quraishi MM. Electrochemical and theoretical investigation of triazole derivatives on corrosion inhibition behavior of copper in hydrochloric acid medium. Corros. Sci. 2013;70:161–169.10.1016/j.corsci.2013.01.025
- Sherif ESM, Erasmus RM, Comins JD. In situ Raman spectroscopy and electrochemical techniques for studying corrosion and corrosion inhibition of iron in sodium chloride solutions. Electrochim. Acta. 2010;55:3657–3665.
- Oguzie EE, Li Y, Wang FH. Effect of surface nanocrystallization on corrosion and corrosion inhibition of low carbon steel: synergistic effect of methionine and iodide ion. Electrochim. Acta. 2007;52:6988–6996.10.1016/j.electacta.2007.05.023
- Cubillos M, Sancy M, Pavez J, et al. Influence of 8-aminoquinoline on the corrosion behaviour of copper in 0.1M NaCl. Electrochim. Acta. 2010;55:2782–2792.10.1016/j.electacta.2009.12.064
- Sherif ESM, Erasmus RM, Comins JD. Inhibition of copper corrosion in acidic chloride pickling solutions by 5-(3-aminophenyl)-tetrazole as a corrosion inhibitor. Corros. Sci. 2008;50:3439–3445.10.1016/j.corsci.2008.10.002
- Zhang DQ, Cai QR, He XM, et al. The corrosion inhibition of copper in hydrochloric acid solutions by a tripeptide compound. Corros. Sci. 2009;51:2349–2354.10.1016/j.corsci.2009.06.015
- Li D, Chen S, Zhao S, et al. The corrosion inhibition of the self-assembled Au and Ag nanoparticles films on the surface of copper. Colloids Surf., A. 2006;273:16–23.10.1016/j.colsurfa.2005.08.003
- Mobarak NAA, Khaled KF, Hamed MNH, et al. Employing electrochemical frequency modulation for studying corrosion and corrosion inhibition of copper in sodium chloride solutions. Arab. J. Chem. 2011;4:185–193.10.1016/j.arabjc.2010.06.036
- Scendo M. Corrosion inhibition of copper by potassium ethyl xanthate in acidic chloride solutions. Corros. Sci. 2005;47:2778–2791.10.1016/j.corsci.2004.12.001
- Mountassir Z, Srhiri A. Electrochemical behaviour of Cu–40Zn in 3% NaCl solution polluted by sulphides: effect of aminotriazole. Corros. Sci. 2007;49:1350–1361.10.1016/j.corsci.2006.07.001
- Khaled KF, Hackerman N. Ortho-substituted anilines to inhibit copper corrosion in aerated 0.5 M hydrochloric acid. Electrochim. Acta. 2004;49:485–495.10.1016/j.electacta.2003.09.005
- Guo W, Chen S, Ma H. A study of the inhibiton of copper corrosion by triethyl phosphate and triphenyl phosphate self-assembled monolayers. J. Serb. Chem. Soc. 2006;71:167–175.10.2298/JSC0602167G
- Sherif EM. Electrochemical and gravimetric study on the corrosion and corrosion inhibition of pure copper in sodium chloride solutions by two azole derivatives. Int. J. Electrochem. Sci. 2012;7:1482–1495.
- ELbakri M, Touir R, Ebntouhami M, et al. Electrosynthesis of adherent poly(3-amino-1,2,4-triazole) films on brass prepared in nonaqueous solvents. Corros. Sci. 2008;50:1538–1545.10.1016/j.corsci.2008.02.014
- Iannuzzi M, Frankel GS. Mechanisms of corrosion inhibition of AA2024-T3 by vanadates. Corros. Sci. 2007;49:2371–2391.10.1016/j.corsci.2006.10.027
- Scendo M, Trela J. Inhibition effect of N-(2-chlorobenzylidene)-4-acetylaniline on the corrosion of stainless steel. Int. J. Electrochem. Sci. 2013;8:11951–11971.
- Olivares O, Likhanova NV, Gómez B, et al. Electrochemical and XPS studies of decylamides of α-amino acids adsorption on carbon steel in acidic environment. Appl. Surf. Sci. 2006;252:2894–2909.10.1016/j.apsusc.2005.04.040
- El-Maksoud SAA. Some phthalazin derivatives as non toxic corrosion inhibitors for copper in sulphuric acid. Electrochim. Acta. 2004;49:4205–4212.10.1016/j.electacta.2004.04.015
- Bentiss F, Bouanis M, Mernari B, et al. Understanding the adsorption of 4H-1,2,4-triazole derivatives on mild steel surface in molar hydrochloric acid. Appl. Surf. Sci. 2007;253:3696–3704.
- Foo KY, Hameed BH. Insights into the modeling of adsorption isotherm systems. Chem. Eng. J. 2010;156:2–10.10.1016/j.cej.2009.09.013
- Anejjar A, Salghi R, Zarrouk A, et al. Adsorption and corrosion inhibition of steel in hydrochloric acid solution by 3-bromo-2-phenylimidazol[1,2-a] pyridine. Int. J. Electrochem. Sci. 2013;8:11512–11525.