Abstract
Purpose
Bronchopulmonary dysplasia (BPD) is a long-term respiratory condition. More than a quarter of extremely premature newborns are harmed by BPD. At present, there are no apparent effective drugs or treatments for the condition. In this study, we aimed to investigate the functional role and mechanism of lymphoid enhancer-binding factor 1 (Lef1) in BPD in vitro.
Materials and Methods
Blood samples from BPD patients and healthy volunteers were gathered, and an in vitro model of BPD was developed in alveolar epithelial cells (AECs) MLE-12 induced by hyperoxia. Then expression of krüppel-like factor 4 (KLF4/Klf4) and LEF1/Lef1 were evaluated. After Lef1 overexpressing plasmid and the vector were transfected into hyperoxia-induced MLE-12 cells, cell proliferation assays were carried out. Cell apoptosis was investigated by a flow cytometry assay, and apoptosis related proteins Bcl-2, cleaved-caspase 3 and 9 were analyzed by a western blot assay. The binding between Klf4 and Lef1 promoter predicted on the JASPAR website was verified using luciferase and ChIP assays. For further study of the mechanism of Klf4 and Lef1 in BPD, gain-of-function experiments were performed.
Results
The mRNA levels of KLF4/Klf4 and LEF1/Lef1 were diminished in clinical BPD serum samples and hyperoxia-induced MLE-12 cells. Overexpression of Lef1 stimulated AEC proliferation and suppressed AEC apoptosis induced by hyperoxia. Mechanically, Klf4 bound to Lef1’s promoter region and aids transcription. Moreover, the results of gain-of-function experiments supported that Klf4 could impede AEC damage induced by hyperoxia via stimulating Lef1.
Conclusion
Klf4 and Lef1 expression levels were declined in hyperoxia-induced AECs, and Lef1 could be transcriptionally activated by Klf4 and protect against hyperoxia-induced AEC injury in BPD. As a result, Lef1 might become a prospective therapeutic target for BPD.
Introduction
Bronchopulmonary dysplasia (BPD) is a long-term respiratory condition. As a complication of premature delivery, BPD is most frequent in premature infants whose gestational age is less than 30 weeks and endangers the survival and wellbeing of premature newborns.Citation1 According to statistics, more than a quarter of extremely premature newborns are harmed by BPD.Citation2 Many processes are involved in the pathophysiology of BPD, with hyperoxia being the leading cause of lung damage in BPD.Citation3 Many researches have now documented numerous BPD treatment techniques, such as prenatal glucocorticoid, surfactant therapy, and interleukin-1 receptor antagonist.Citation4,Citation5 The management of BPD has been continuously strengthened, but the probability of premature infants developing BPD and the mortality rate of premature infants with BPD remain significant difficulties.Citation6 At present, there are no apparent and effective drugs or treatments.Citation7 As a result, the current focus of research is on elucidating the mechanism and potential therapeutic targets for BPD.
Lymphoid enhancer-binding factor 1 (LEF1) is a transcription factor that belongs to the family of T cell factor.Citation8 As a transcription factor, Lef1 participates in the typical Wnt/β-catenin pathway.Citation9,Citation10 Meanwhile, LEF1 has been linked to the occurrence and development of a variety of diseases.Citation11,Citation12 Researches have demonstrated that Lef1 can enhance B cell proliferationCitation13 as well as differentiation.Citation14 Cai et al.Citation15 found that LEF1 expression declines with increasing BPD severity, and in that case, LEF1 is adversely linked with BPD development. In a recent work, we discovered that the expression of LEF1 in the blood of BPD preterm newborns reduced as the severity of the condition increased.Citation16 However, the role and mechanism of LEF1 in BPD remain unknown.
Krüppel-like factor 4 (KLF4) is a zinc finger proteinCitation17 and could participate in many processes of cells, such as proliferation, cell cycle, apoptosis, migration and differentiation.Citation18–21 Also, KLF4 is involved in a variety of illnesses, such as atherosclerosis,Citation22 acute myeloid leukemia,Citation23 and neonatal pneumonia.Citation24 Via affecting cell apoptosis and damage of inflammation, KLF4 is associated with pneumonia.Citation25 Alejandre Alcazar et al.Citation21 found that Klf4 expression was diminished in lung tissue after treating the neonatal mouse with mechanical ventilation with O2-rich gas. However, the role of KLF4 in BPD remain unclear. In this study, as per bioinformatics predictions, Klf4 might well be paired with Lef1 in the promoter.
With the foundation of the above findings, we hypothesize that Klf4 and Lef1 are downregulated in BPD, and that Klf4 activates Lef1 production by enriching in the Lef1 promoter, therefore preventing the harm of AECs caused by hyperoxia. This research seeks to afford a theoretical basis for Lef1 as a viable treatment target for BPD.
Methods
Clinical sample collection
This research, which was authorized by Hunan Children’s Hospital’s institutional review committee (approval number: KYSQ2022-132), involved twenty infants delivered at <32 weeks of pregnancy. Infants were followed up on until 36 weeks of pregnancy after conception, with the parents’ informed agreement, to see if they had BPD. All 20 infants fulfill the Ministry of Child Health and Human Development’s BPD diagnosis criteria. BPD illness is defined as any oxygen dependency that lasts more than 28 days (FiO2 > 21%). On the 28th day of birth, blood was obtained from the radial artery.Citation3,Citation21 Blood from healthy volunteers was collected as control. Then, the blood samples were centrifuged at 3500 rpm for 10 min to obtain serums for subsequent experiments. The basic characteristics of subjects and controls were listed in .
Table 1. Characteristics of enrolled infants.
Cell culture and transfection
American Type Culture Collection (ATCC, Manacas, Virginia, USA) supplied mouse type II AECs (MLE-12). Dulbecco’s modified Eagle’s medium (DMEM; Gibco, USA) supplied with two percent fetal bovine serum (FBS) was utilized to cultivate MLE-12 cells. MLE-12 cells were cultivated for 24 h under conditions of 5% CO2, 37 °C, followed by exposing to hyperoxia (95% O2+5% CO2) for 24 h.Citation6,Citation26 MLE-12 cells cultured under normoxia was used as control group.
For overexpression of Lef1 and Klf4, mouse Lef1 and Klf4 cDNA were PCR-amplified and then cloned into the expression vector pcDNA3.1 (RiboBio, Guangzhou, China). For silencing Lef1, short interfering RNA (siRNA) targeting Lef1 designed and synthesized by GenePharma (Shanghai, China). When the cell density met 70-80%, plasmids oe-Lef1 (overexpression of Lef1), oe-Klf4 (overexpression of Klf4), si-Lef1 (siRNA targeting Lef1; 5′-ATCATCACAAACTTTATTCTTGG-3′) and their controls vector; si-NC: 5′-UGUCUCCGAACGUGUCACGUC-3′) were transfected for 48 h following the manual of Lipofectamine 3000 (Invitrogen, Carlsbad, CA, USA).Citation6
Cell counting kit-8 (CCK-8) assay
Cells were seeded in 96-well plates at a density of 5 × 103 cells/well and cultured for 24 hours, followed by adding CCK-8 reagent (Beyotime, Shanghai, China). After constantly fostering 3 hours at 37 °C, a microplate reader (Bio-Rad, Hercules, CA, USA) was used to determine the optical density (OD) at 450 nm.Citation6,Citation22
5-Ethynyl-2’-deoxyuridine (EdU) assay
Cell proliferation was evaluated using Cell-Light EdU Apollo 567 kits (RiboBio). After adding 50 M of EdU labeling medium, the cells were cultured for 2 hours. Following that, 4% paraformaldehyde was used to fix the cells, and 0.5 percent TritonX-100/phosphate buffered saline (PBS) was used to permeabilize it. Then cells were dyed using Apollo staining reagent and 4′, 6-diamidino-2-phenylindole (DAPI). An IX73-AIZFL/PH inverted fluorescence microscope (OLYMPUS Corporation, Tokyo, Japan) was utilized to capture images.Citation27
Flow cytometry assay
To assess cell apoptosis, the Annexin V-fluorescein isothiocyanate (FITC)/Propidium iodide (PI) Apoptosis Detection Kit (Sigma-Aldrich, Louis, MO, USA) was utilized. After resuspending 1 × 105 cells in 500 μL binding buffer, cell suspension was well mixed with Annexin V-FITC reagent (5 μL) and PI solution (10 μL). After incubation in dark for 30 minutes, the apoptosis of MLE-12 was then assessed utilizing a flow cytometer (BD Biosciences, San Jose, CA, USA).Citation22
Quantitative real-time PCR (qRT-PCR) assay
RNA extraction from serum samples or MLE-12 cells utilizing RNAiso Plus reagent (Takara, Shiga, Japan) and cDNA reverse transcription utilizing Omniscript Reverse-Transcription Kit (Qiagen, Hilden, Germany) were conducted as previously Reported.Citation28 qRT-PCR was carried out utilizing Applied Biosystems 7500 instrument and SYBR Green Kit (Takara, Dalian, China).Citation6,Citation28 The sequences of primers are listed in . β-actin was employed as endogenous controls. The relative gene expression was calculated using the 2-ΔΔCT method.Citation22,Citation28
Table 2. Primer sequences for qRT-PCR.
Western blot assay
Total proteins from MLE-12 cells were obtained utilizing RIPA buffer (Beyotime, Shanghai, China) and western blot assay was carried as reported before.Citation6,Citation7 The primary antibodies used were KLF4 (1:1000, ab214666, Abcam, Cambridge, UK), LEF1 (1:1000, ab137872, Abcam), Bcl-2 (1:2000, ab182858, Abcam), cleaved-caspase 3 (1:5000, ab214430, Abcam), cleaved-caspase 9 (1:1000, ab184768, Abcam), and GAPDH (1:10000, ab181602, Abcam). Goat anti-rabbit IgG (1:10000, ab6721, Abcam) were used as secondary antibody.
Dual luciferase reporter assay
The luciferase reporter vectors containing Klf4 binding site (Lef1-WT) or binding site mutation (Lef1-MUT) gene sequences of Lef1 promoter were constructed, and co-transfected with oe-Klf4 into MLE-12 cells. Following 24 h transfection, the luciferase reporter experiment was carried out employing a dual-luciferase assay kit (Promega, Madison, WI, USA) in accordance with the manufacturer’s instructions. The intensity of fluorescence was evaluated utilizing a Multiskan Spectrum (Thermo Fisher Scientific, Waltham, MA, USA).Citation29,Citation30
Chromatin immunoprecipitation (ChIP)
MLE-12 cells (2 × 106) were processed for ChIP assay utilizing a ChIP assay kit (Millipore, MA, USA) as per the manufacturer’s instructions. The precipitated DNA samples were gathered, and fragments of the Lef1 promoter region were amplified and evaluated using qRT-PCR.Citation30 The primer sequences for the Lef1 promoter were as follows: forward, 5′-GGAGGAGGGCATCA GATCTTATTGTGAGTGGTTG‐3′; reverse: 5′-TTAGGAGGCAAGGTACATA TTTAAGGGGGAGAG‐3′. Anti-KLF4 (11880-1-AP, 0.5 μg) antibody was purchased from Proteintech (USA).
Statistical analysis
SPSS 22.0 statistical software (IBM Corp. Armonk, NY, USA) was employed to evaluate all of the data. The data from at least 3 separate tests was presented as mean ± standard deviation (SD). Statistical comparisons were made employing the student t-test for two groups, or Tukey’s test-corrected one-way analysis of variance (ANOVA) for multiple groups. With P < 0.05 deemed statistically significant.
Results
The expression of KLF4/Klf4 and LEF1/lef1 are diminished in clinical BPD serum samples and hyperoxia-induced MLE-12 cells
Serum samples from BPD patients and healthy volunteers were gathered and the expression of KLF4 and LEF1 were evaluated utilizing qRT-PCR. The data documented that KLF4 and LEF1 expression had apparently declined by 38% and 44% in the BPD group in comparison with the healthy group (). An in vitro model of BPD was developed in MLE-12 cells induced by hyperoxia, and the expression of Klf4 and Lef1 was evaluated by qRT-PCR assay. It elucidated that compared with the normoxia group, hyperoxia induced low expression of Klf4 and Lef1 (). Also, KLF4 and LEF1 levels were downregulated by 41% and 45% in hyperoxia-induced cells measured by western blot ().
Figure 1. The expression of KLF4/Klf4 and LEF1/Lef1 are diminished in Clinical BPD serum samples and hyperoxia-induced MLE-12 cells. A-B. The levels of KLF4 and LEF1 in serum samples from BPD patients (n = 20) and healthy volunteers (n = 20) were evaluated utilizing qRT-PCR, male: grey, female: black; C. Klf4 and Lef1 expression were measured by qRT-PCR; D. The levels of KLF4 and LEF1 in MLE-12 cells induced by hyperoxia were evaluated utilizing western blot. Data were displayed as mean ± SD (n = 3), and analyzed utilizing t test; **, p < 0.01; ***, p < 0.001.
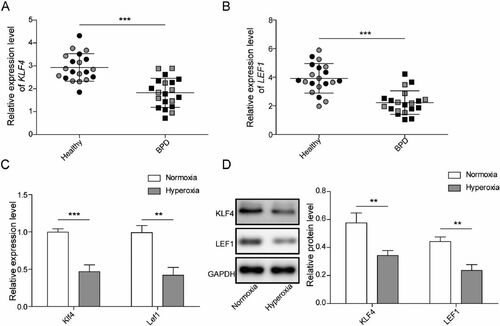
Overexpression of Lef1 suppresses hyperoxia-induced AEC injury
To explore the function of Lef1 in BPD, oe-Lef1 and the vector were transfected into hyperoxia-induced MLE-12 cells. The results elucidated that Lef1 mRNA expression and LEF1 protein levels (increased by 1.1 times) were higher in the hyperoxia + oe-Lef1 group than in the hyperoxia + vector group (). After that, CCK-8 and EdU assays were carried out to evaluate cell proliferation. The data documented that cell proliferation repressed by hyperoxia was dramatically stimulated by oe-Lef1 (). The effect of oe-Lef1 on cell apoptosis was investigated by flow cytometry assay, and the results revealed that cell apoptosis induced by hyperoxia was dramatically diminished by oe-Lef1 (). Moreover, Bcl-2, cleaved-caspase 3 and 9 were analyzed, and the results of western blot showed hyperoxic conditions decreased expression of Bcl-2 by 40% and elevated the expression of cleaved-caspase 3 and 9 by 2.4 and 1.2 times, but oe-Lef1 strongly reversed the expression of the aforementioned apoptosis related proteins in the presence of hyperoxia (). Collectively, these results revealed that Lef1 could suppress hyperoxia-induced AEC injury.
Figure 2. Overexpression of Lef1 suppresses hyperoxia-induced AEC injury. A. The expression of Lef1 was evaluated by qRT-PCR; B. Western blot assay for LEF1 level after transfecting oe-LEF1 into hyperoxia-induced MLE-12 cells; C. The cell viability was determined using CCK-8 assay; D. The cell proliferation was determined using EdU assay; E. Cell apoptosis was investigated by flow cytometry assay; F. The expression of Bcl-2, cleaved-caspase 3 and 9 were determined using western blot. Data were displayed as mean ± SD (n = 3) and analyzed utilizing one-way ANOVA, followed by Tukey’s multiple comparisons test; **, p < 0.01; ***, p < 0.001.
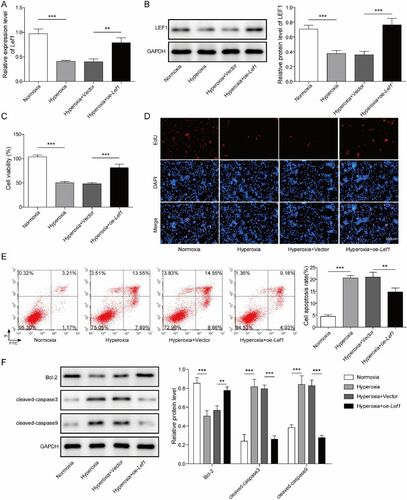
Klf4 activates the transcription of Lef1
Klf4 functions as a transcription factor, regulating the transcription of target genes. To evaluate the link between Klf4 and Lef1, we utilized JASPAR (https://jaspar.genereg.net/) to predict probable Klf4 binding sites in the Lef1 promoter (). The luciferase test was used to validate the prediction. After co-transfecting oe-Klf4 and Lef1-WT or Lef1-MUT into MLE-12 cells, oe-Klf4 increased Lef1-WT luciferase activity but had no impact on Lef1-MUT (). Moreover, the ChIP assay revealed that Klf4 was enriched in the promoter of Lef1 (). These results revealed that Klf4 could bind to the promoter of Lef1, and hyperoxia increased the combination (). After transfection with oe-Klf4 in normoxia or hyperoxia treated MLE-12 cells, and the findings revealed that oe-Klf4 significantly increased Lef1 expression () and LEF1 level (increased by 47% for normoxia condition and 1.1 times for hyperoxia condition; ). It was concluded from these findings that Klf4 binds to Lef1’s promoter region and aids transcription.
Figure 3. Klf4 activates the transcription of Lef1. A. JASPAR (https://jaspar.genereg.net/) was employed to predict probable Klf4 binding sites in the Lef1 promoter; B. The luciferase and C. ChIP assays were used to validate the prediction from JASPAR. D. Lef1 expression was determined by qRT-PCR; E. Western blot assay for LEF1 level after transfection in normoxia or hyperoxia treated MLE-12 cells with oe-Klf4. Data were displayed as mean ± SD (n = 3) and analyzed utilizing t test or one-way ANOVA followed by Tukey’s multiple comparisons test; ***, p < 0.001.
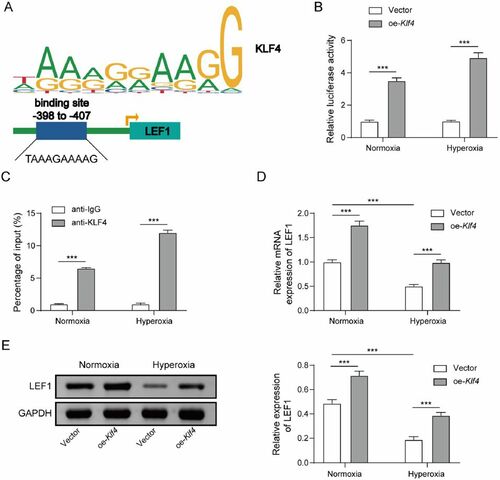
Klf4 impedes hyperoxia-induced AEC damage via stimulating Lef1
To further study the mechanism of Klf4 and Lef1 in BPD, oe-Klf4 and si-Lef1 were co-transfected into hyperoxia-induced MLE-12 cells. After that, CCK-8 and EdU assays were carried out to evaluate cell proliferation. The data documented that oe-Klf4 significantly enhanced cell proliferation that had been suppressed by hyperoxia, whereas si-Lef1 reversed the effect of oe-Klf4 (). Flow cytometry assay was applied to evaluate cell apoptosis, and the analysis revealed that oe-Klf4 significantly reduced hyperoxia-induced cell apoptosis whereas si-Lef1 enhanced it (). Finally, western blot analysis was used to determine the expression of Bcl-2, cleaved-caspase 3 and 9, and the findings indicated that oe-Klf4 greatly diminished hyperoxia-induced cleaved-caspase 3 and 9 by 42% and 52%, and increased those of Bcl-2 by 56%, but si-Lef1 exerted absolutely opposite effects on expression levels. (). In a word, Klf4 could impede AEC damage induced by hyperoxia via stimulating Lef1.
Figure 4. Klf4 impedes hyperoxia-induced alveolar epithelial cell damage via stimulating Lef1. A The cell viability was determined using CCK-8 assay after co-transfecting oe-Klf4 and si-Lef1 into hyperoxia-induced MLE-12 cells; B. The cell proliferation was determined using EdU assay; C. Cell apoptosis was investigated by flow cytometry assay; D. The expression of Bcl-2, cleaved-caspase 3 and 9 were determined using western blot. Data were displayed as mean ± SD (n = 3) and analyzed utilizing one-way ANOVA, followed by Tukey’s multiple comparisons test; *, p < 0.05; ***, p < 0.001.
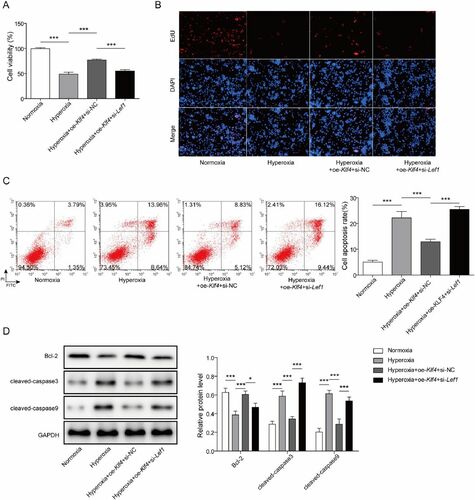
Discussion
BPD stunts children’s development and affects their respiratory, neurological, mobility, and hearing systems. The respiratory system’s influence is mainly seen in infancy and adulthood. Recurrent infections, pulmonary hypertension, and poor lung function are all elevated. Because BPD has such severe consequences, early management for preterm babies with BPD risk factors is critical.Citation2 At present, there are many researches on the molecular mechanism of BPD. Liang et al.Citation31 reported that Semaphorin 3 A (Sema3a) attenuated hyperoxia-induced apoptosis and inflammation in newborn rats via blocking the extracellular signal-regulated kinase (Erk)/c-Jun N-terminal kinase (Jnk) pathway. Huang et al.Citation32 found that blocking fatty acid-binding protein 4 (Fabp4) reduces hyperoxia-induced lung damage and fibrosis. Research conducted by Zhang et al.Citation33 reported that miR-214 decreases type II AEC apoptosis and fibrosis through blocking the placental growth factor (PlGF)-dependent signal transducer and activator of transcription 3 (Stat3) pathway. The type II AEC injury, which is regarded to be the pathological foundation of BPD, was the primary subject on which we concentrated in this research.Citation1
Lef1 is a transcription factor that is known to be involved in canonical Wnt signaling and to regulate cell proliferation during lung development.Citation34 Lef1 is found to be abundantly expressed in the alveolar epithelium and mesenchyme adjacent to the lung epithelium.Citation35 By recruiting to the promoter of vascular endothelial growth factor (Vegf), Lef1 elevates expression of Vegf and promotes epithelial and endothelial cell proliferation.Citation36 Additionally, LEF1 may interact directly with the miR-124 promoter region to influence the maturation of embryonic pulmonary epithelial cells.Citation37 To elucidate the regulatory mechanism underlying BPD, this work focused on LEF1/Lef1 and discovered that LEF1/Lef1 expression was diminished in clinical BPD serum samples and hyperoxia-induced MLE-12 cells. Additionally, we discovered that overexpression of Lef1 might protect AECs from hyperoxia-induced damage.
Numerous researchers have depicted that transcription factors are critical for lung development, such as forkhead box F1 (Foxf1),Citation38 Yes-associated protein (Yap)Citation39 and SRY-related high-mobility group box 9 (SOX9).Citation40 As a transcription factor, KLF4 is required for cell differentiation and proliferation in a variety of disorders.Citation41 Klf4 is identified as the most substantially changed gene in the lung after birth,Citation42 and is found in the endothelial cells of the pulmonary arterial and venous of mice.Citation43 The data of this research revealed that KLF4/Klf4 expression was diminished in clinical BPD serum samples and hyperoxia-induced MLE-12 cells. In its function as a transcription factor, KLF4 transcriptionally modulates the expression of insulin receptor (INSR) and diminishes the development of osteoarthritis, according to research of Liu et al..Citation44 It may also attach to the C-X-C motif chemokine ligand 8 (CXCL8) promoter, which consequently promotes the proliferation of gastric cancer cells.Citation30 Besides, Wang et al.Citation45 revealed that by directly interacting to the tissue inhibitor of metalloproteinase 3 (TIMP3) promoter, KLF4 may stimulate the transcription of the lung tumor suppressor TIMP3. In this research, the data elucidated that Klf4 could bind to Lef1’s promoter region and aid its transcription. Moreover, the results of gain-of-function experiments supported that Klf4 had the potential to protect against AEC damage caused by hyperoxia through stimulating Lef1.
In conclusion, the Klf4-Lef1 axis played a crucial role in hyperoxia-induced AEC injury. KLF4/Klf4 and LEF1/Lef1 expression levels were declined in clinical BPD serum samples and hyperoxia-induced MLE-12 cells, respectively, and overexpression of Lef1 could protect AECs from hyperoxia-induced damage. As a target gene of Klf4, Lef1 could be transcriptionally activated by Klf4. Klf4 overexpression could protect against AEC damage caused by hyperoxia through stimulating Lef1. As a result, Lef1 might become a prospective therapeutic target for BPD.
Ethics statement
This research was authorized by Hunan Children’s Hospital’s institutional review committee (approval number: KYSQ2022-132). All patients were informed of the study and signed the written consent.
Acknowledgements
Thanks to the members of our laboratory for their contributions.
Disclosure statement
No potential conflict of interest was reported by the authors.
Data availability statement
The datasets used or analyzed during the current study are available from the corresponding author on reasonable request.
Additional information
Funding
References
- Hou A, Fu J, Shi Y, et al. Decreased ZONAB expression promotes excessive transdifferentiation of alveolar epithelial cells in hyperoxia-induced bronchopulmonary dysplasia. Int J Mol Med. 2018;41(4):2339–2349. doi:10.3892/ijmm.2018.3413.
- Zhu X, Wang F, Lei X, et al. Resveratrol alleviates alveolar epithelial cell injury induced by hyperoxia by reducing apoptosis and mitochondrial dysfunction. Exp Biol Med (Maywood). 2021;246(5):596–606. doi:10.1177/1535370220975106.
- Dong W, Zhu X, Liu X, et al. Role of the SENP1-SIRT1 pathway in hyperoxia-induced alveolar epithelial cell injury. Free Radic Biol Med. 2021;173:142–150. doi:10.1016/j.freeradbiomed.2021.07.027.
- El-Saie A, Shivanna B. Novel strategies to reduce pulmonary hypertension in infants with bronchopulmonary dysplasia. Front Pediatr. 2020;8:201.
- Principi N, Di Pietro GM, Esposito S. Bronchopulmonary dysplasia: clinical aspects and preventive and therapeutic strategies. J Transl Med. 2018;16(1):36. doi:10.1186/s12967-018-1417-7.
- Zhong Q, Wang L, Qi Z, et al. Long Non-coding RNA TUG1 modulates expression of elastin to relieve bronchopulmonary dysplasia via sponging miR-29a-3p. Front Pediatr. 2020;8:573099.
- Syed M, Das P, Pawar A, et al. Hyperoxia causes miR-34a-mediated injury via angiopoietin-1 in neonatal lungs. Nat Commun. 2017;8(1):1173. doi:10.1038/s41467-017-01349-y.
- Behrens J, von Kries JP, Kühl M, et al. Functional interaction of beta-catenin with the transcription factor LEF-1. Nature. 1996;382(6592):638–642. doi:10.1038/382638a0.
- Tan RJ, Zhou D, Zhou L, et al. Wnt/beta-catenin signaling and kidney fibrosis. Kidney Int Suppl (2011). 2014;4(1):84–90. doi:10.1038/kisup.2014.16.
- Clevers H. Wnt/beta-catenin signaling in development and disease. Cell. 2006;127(3):469–480. doi:10.1016/j.cell.2006.10.018.
- Du X, Liu H, Yang C, et al. LncRNA landscape analysis identified LncRNA LEF-AS1 as an oncogene that upregulates LEF1 and promotes survival in chronic lymphocytic leukemia. Leuk Res. 2021;110:106706.
- Fakhr E, Zare F, Azadmanesh K, et al. LEF1 silencing sensitizes colorectal cancer cells to oxaliplatin, 5-FU, and irinotecan. Biomed Pharmacother. 2021;143:112091.
- Wang SH, Nan KJ, Wang YC. Endothelial cells promote the proliferation of lymphocytes partly through the Wnt pathway via LEF-1. Biochem Biophys Res Commun. 2009;388(1):67–72. doi:10.1016/j.bbrc.2009.07.117.
- Jing Y, Kang D, Liu L, et al. Dedicator of cytokinesis protein 2 couples with lymphoid enhancer-binding factor 1 to regulate expression of CD21 and B-cell differentiation. J Allergy Clin Immunol. 2019;144(5):1377–1390 e4. doi:10.1016/j.jaci.2019.05.041.
- Cai Y, Ma F, Qu L, et al. Weighted gene co-expression network analysis of key biomarkers associated with bronchopulmonary dysplasia. Front Genet. 2020;11:539292.
- Yang M, Chen B-L, Huang J-B, et al. Angiogenesis-related genes may be a more important factor than matrix metalloproteinases in bronchopulmonary dysplasia development. Oncotarget. 2017;8(12):18670–18679. doi:10.18632/oncotarget.14722.
- Chen C, Mao X, Cheng C, et al. miR-135a reduces osteosarcoma pulmonary metastasis by targeting both BMI1 and KLF4. Front Oncol. 2021;11:620295.
- Qiaoli S, Yi S, Jie Z, et al. KLF2 and caveolin-1 as early indicators of acute lung injury induced by paraquat. Eur Rev Med Pharmacol Sci. 2016;20(1):138–145.
- Chen X, Whitney EM, Gao SY, et al. Transcriptional profiling of Kruppel-like factor 4 reveals a function in cell cycle regulation and epithelial differentiation. J Mol Biol. 2003;326(3):665–677. doi:10.1016/S0022-2836(02)01449-3.
- Ghaleb AM, McConnell BB, Kaestner KH, et al. Altered intestinal epithelial homeostasis in mice with intestine-specific deletion of the Kruppel-like factor 4 gene. Dev Biol. 2011;349(2):310–320. doi:10.1016/j.ydbio.2010.11.001.
- Alejandre Alcazar MA, Kaschwich M, Ertsey R, et al. Elafin treatment rescues EGFR-Klf4 signaling and lung cell survival in ventilated newborn mice. Am J Respir Cell Mol Biol. 2018;59(5):623–634. doi:10.1165/rcmb.2017-0332OC.
- Dong H, Jiang G, Zhang J, et al. MiR-506-3p promotes the proliferation and migration of vascular smooth muscle cells via targeting KLF4. Pathobiology. 2021;88(4):277–288. doi:10.1159/000513506.
- Lewis AH, Bridges CS, Punia VS, et al. Kruppel-like factor 4 promotes survival and expansion in acute myeloid leukemia cells. Oncotarget. 2021;12(4):255–267. doi:10.18632/oncotarget.27878.
- Zhang L, Yan H, Wang H, et al. MicroRNA (miR)-429 promotes inflammatory injury by targeting kruppel-like factor 4 (KLF4) in neonatal pneumonia. Curr Neurovasc Res. 2020;17(1):102–109.
- Wang Q, Zhang X, Chen D. circ_VMA21 protects WI-38 cells against LPS-induced apoptotic and inflammatory injury by acting on the miR-409-3p/KLF4 axis. Gen Physiol Biophys. 2021;40(4):275–287. doi:10.4149/gpb_2021011.
- Das KC. Hyperoxia decreases glycolytic capacity, glycolytic reserve and oxidative phosphorylation in MLE-12 cells and inhibits complex I and II function, but not complex IV in isolated mouse lung mitochondria. PLoS One. 2013;8(9):e73358. doi:10.1371/journal.pone.0073358.
- Li G-Q, Fang Y-X, Liu Y, et al. MicroRNA-21 from bone marrow mesenchymal stem cell-derived extracellular vesicles targets TET1 to suppress KLF4 and alleviate rheumatoid arthritis. Ther Adv Chronic Dis. 2021;12:20406223211007369. doi:10.1177/20406223211007369.
- Paik S, Jung HS, Lee S, et al. miR-449a regulates the chondrogenesis of human mesenchymal stem cells through direct targeting of lymphoid enhancer-binding factor-1. Stem Cells Dev. 2012;21(18):3298–3308. doi:10.1089/scd.2011.0732.
- Zhang X, Sun Z, Guo S, et al. E3 ubiquitin ligase march1 facilitates OX40L expression in allergen-stimulated dendritic cells through mediating the ubiquitination of HDAC11. J Asthma Allergy. 2021;14:955–966. doi:10.2147/JAA.S318104.
- Liu Z, Wu X, Tian Y, et al. H. pylori infection induces CXCL8 expression and promotes gastric cancer progress through downregulating KLF4. Mol Carcinog. 2021;60(8):524–537. doi:10.1002/mc.23309.
- Liang Z, Zhang X, Liu Y, et al. SEMA3A protects against hyperoxia-induced lung injury in a bronchopulmonary dysplasia model of newborn rat by inhibiting ERK pathway. Allergol Immunopathol (Madr). 2021;49(6):8–15. doi:10.15586/aei.v49i6.478.
- Huang LT, Chou HC, Chen CM. Inhibition of FABP4 attenuates hyperoxia-induced lung injury and fibrosis via inhibiting TGF-beta signaling in neonatal rats. J Cell Physiol. 2021;237(2):1509–1520. doi:10.1002/jcp.30622.
- Zhang Z-Q, Hong H, Li J, et al. MicroRNA-214 promotes alveolarization in neonatal rat models of bronchopulmonary dysplasia via the PlGF-dependent STAT3 pathway. Mol Med. 2021;27(1):109. doi:10.1186/s10020-021-00396-y.
- Tsuji M, Morishima M, Shimizu K, et al. Foxc2 influences alveolar epithelial cell differentiation during lung development. Dev Growth Differ. 2017;59(6):501–514. doi:10.1111/dgd.12368.
- Tebar M, Destrée O, de Vree WJ, et al. Expression of Tcf/Lef and sFrp and localization of beta-catenin in the developing mouse lung. Mech Dev. 2001;109(2):437–440. doi:10.1016/S0925-4773(01)00556-1.
- Ke H, Masoumi KC, Ahlqvist K, et al. Nemo-like kinase regulates the expression of vascular endothelial growth factor (VEGF) in alveolar epithelial cells. Sci Rep. 2016;6:23987.
- Wang Y, Huang C, Chintagari NR, et al. miR-124 regulates fetal pulmonary epithelial cell maturation. Am J Physiol Lung Cell Mol Physiol. 2015;309(4):L400–13. doi:10.1152/ajplung.00356.2014.
- Braeuer RR, et al. Transcription factor FOXF1 identifies compartmentally distinct mesenchymal cells with a role in lung allograft fibrogenesis. J Clin Invest. 2021;131(21):e147343. doi:10.1172/JCI147343.
- Gokey JJ, Snowball J, Sridharan A, et al. YAP regulates alveolar epithelial cell differentiation and AGER via NFIB/KLF5/NKX2-1. iScience. 2021;24(9):102967. doi:10.1016/j.isci.2021.102967.
- Li L, Feng J, Zhao S, et al. SOX9 inactivation affects the proliferation and differentiation of human lung organoids. Stem Cell Res Ther. 2021;12(1):343. doi:10.1186/s13287-021-02422-6.
- Lin L, Han Q, Xiong Y, et al. Krupple-like-factor 4 attenuates lung fibrosis via inhibiting epithelial-mesenchymal transition. Sci Rep. 2017;7(1):15847. doi:10.1038/s41598-017-14602-7.
- Jean J-C, George E, Kaestner KH, et al. Transcription factor Klf4, induced in the lung by oxygen at birth, regulates perinatal fibroblast and myofibroblast differentiation. PLoS One. 2013;8(1):e54806. doi:10.1371/journal.pone.0054806.
- Hamik A, Lin Z, Kumar A, et al. Kruppel-like factor 4 regulates endothelial inflammation. J Biol Chem. 2007;282(18):13769–13779. doi:10.1074/jbc.M700078200.
- Liu W, Chen Y, Zeng G, et al. INSR mediated by transcription factor KLF4 and DNA methylation ameliorates osteoarthritis progression via inactivation of JAK2/STAT3 signaling pathway. Am J Transl Res. 2020;12(12):7953–7967.
- Wang X, Xia S, Li H, et al. The deubiquitinase USP10 regulates KLF4 stability and suppresses lung tumorigenesis. Cell Death Differ. 2020;27(6):1747–1764. doi:10.1038/s41418-019-0458-7.