ABSTRACT
Curbing water scarcity problems in semi-arid regions is a top priority for economic and social development. Alternative strategies for sustainable domestic water supply are therefore required to augment water supply with affordable cost and technology. Scant research findings in sub-Saharan Africa have, however, reported inefficiency in adapting to water scarcity problems in the region. Therefore, an investigation of domestic rainwater harvesting (RWH) reliability is important to identify factors affecting the system. We found that rainwater harvesting with the existing traditional RWH systems in Mekelle city was unreliable. Unreliability was attributed to inefficient design (the systems were installed without considering deterministically the stochastic nature of rainfall), family size, water demand, rooftop area and storage tank size.
Editor M.C. Acreman; Associate editor S. Kanae
1 Introduction
Having physical access to a sufficient, safe and affordable water supply is a basic human right (UN Citation1977, Pajankar and Rode Citation2012, Basnet Citation2013). Although the number of people with access to improved water supply sources in the world is estimated to have increased based on proxy indicators (WHO/UNICEF Citation2014), there are more than 748 million people with unimproved access to water supply. Forty three percent (321.64 million people) of them live in sub-Saharan Africa (percent of population with unimproved access to water supply: Ethiopia 48%, Chad 49%, Madagascar 50%, Mozambique 51% and Congo Democratic Republic 53%) (WHO/UNICEF Citation2014). In fact, there is rising concern about access to water supply in semi-arid regions of sub-Saharan Africa which has the largest share of low water access. Five of the bottom listed countries in the Joint Monitoring Programme (JMP) report are found in the semi-arid region of the African continent, except Papua New Guinea in Southeast Asia where 60% of people live without access to improved water sources. The increased demand for water is mainly associated with population growth and economic development, while environmental needs, climate change, water pollution and over abstraction of groundwater will worsen water shortages (Durham et al. Citation2003, Ngigi Citation2009).
Table 1. Descriptive statistics of households with complete rainwater harvesting in Mekelle city, 2014.
In sub-Saharan Africa, water demand surpasses available resources in a number of countries. Many of them face either water stress (<1700 m3 per capita per annum) or water scarcity (less than 1000 m3 per capita per annum), as reported by Ngigi (Citation2009). Moreover, water stress is predicted to affect about 50–250 million Africans by 2020 (Parry et al. Citation2007). Similarly, a water indicator, used to evaluate availability per capita per year threshold by Falkenmark et al. (Citation1989), defined Ethiopia as a water-stressed country. The list of water-scarce countries by the year 2025 is expected to include Comoros, Egypt, Haiti, Iran, Libya, Morocco, Oman, South Africa and Syria, according to UN population projections (Rijsberman and Frank Citation2006). Hence, all possible ways must be explored to mitigate the physical and economic scarcity of access to safe drinking water in sub-Saharan Africa. In this regard, rainwater harvesting (RWH) is gaining considerable momentum as part of the global effort to secure water for the future (Sazakli et al. Citation2007). RWH has been demonstrated to be a practical and effective supplement to potable water supply in areas where groundwater and/or surface water sources are limited (Liaw et al. Citation1997).
In Ethiopia, self-motivated, non-governmental organizations have recently introduced RWH technologies as a source of supplementary drinking water. Their interest suggests the importance of rainwater harvesting as one remedy for water scarcity. RWH systems will expand further as an adaptation strategy in the semi-arid regions of the country in the near future. The traditional experience in the area, however, requires a scientific base to use as a mitigation strategy. Therefore, the objectives of this research were (1) to analyse the long-term rainfall distribution, (2) to investigate the current RWH system and (3) to assess the reliability of existing RWH systems using Mekelle city as a case study. The results may serve as a stepping-stone in evaluating the success and failure of pre- and post-RWH projects. It could be also useful for the development of a low-cost RWH system and for its implementation in water-scarce regions nationwide.
2 Study area and method
2.1 Study area
The study was conducted in Mekelle city, the capital and commercial centre of the Tigray Regional State in northern Ethiopia (). The city is located at latitude 13°32′ N and longitude 39°33′ E and is situated in the extension of the central highlands of Ethiopia with an altitude range of 1965–2220 m a.s.l. It is bounded by mountain ranges to the east and north (EMA Citation1981). The area’s moisture index (P/ET) is 0.25–0.5, which makes it a moderately dry area, with annual rainfall of 600–700 mm (Gebremedhin Citation2002). The mean annual temperature ranges from 16°C to 20°C. The geological formation of the area is dominated by limestone and dolomite, a landscape characterized by sinkholes, caves and underground drainage systems (Frot et al. Citation2008). Based on a projection of the 2006 national census, the total population in 2014 was 298.736 × 103, with an annual growth rate of 4.7%.
Mekelle, one of the fastest growing urban centres in the country, is facing severe constraints on services, among which water supply is the most critical. The city’s water supply is dependent on a highly mineralized and intermittent groundwater source and on water distribution using vacuum trucks. Use of surface water is not an option in the area due to the limestone and dolomite dominated geological formation. Very recently, RWH was introduced as an alternative water supply system in the city suburbs by a nongovernmental organization, Helvetas Swiss International Ethiopia, in collaboration with the regional government.
2.2 Method
2.2.1 Data collection
We conducted a primary assessment to identify households in the study area with complete RWH systems. Among the 67 households, we identified 35 systems that were not functional during the time of data collection (some were new and the households had not yet started using them, and others were out of use because they were malfunctioning). We included the remaining 32 households in the study and used them for the analysis. We collected data from these households related to daily water consumption, family size, tank size and roof area. We used a tape measure to measure roof area and tank size. We also obtained historical rainfall data for the period 1998–2012 from the Mekelle Branch Office of the Ethiopian Meteorological Agency.
2.2.2 Reliability analysis
The reliability of a RWH system has been defined as the measure of its ability to produce the water needed by its users. It is also defined as the percentage of days in a year when the rainwater tank is able to supply the intended partial demand for a particular condition (Imteaz et al. Citation2013). So far, several different models have been used to assess the reliability of rainwater harvesting (Lall et al. Citation1996, Gould and Nissen-Petersen Citation1999, Vaes and Berlamont Citation2001, Fewkes Citation2004, Kim and Han Citation2006, Roebuck and Ashley Citation2006, Ghisi et al. Citation2007, Cowden et al. Citation2008). For this study, we adapted a mass balance equation that is already tested and used by researchers (Ward et al. Citation2008) to investigate the reliability of the existing RWH systems in the semi-arid city of Mekelle, using the equations (1), (2) and (3).
where Vt is the volume of water in the tank at present, Vt−1 is the volume of water in the tank remaining from the previous time step, Qt is the rainwater captured at present, Dt is the total consumption per month and Vs is the volume of the tank.
where Qt is the rainwater captured at present, C is the roof runoff coefficient, which was assumed to be 0.8 for a corrugated iron sheet with a range of 0.75–0.85 (the literature indicated insignificant differences among the end results of the values (Yusuf Citation1999)), It is the average monthly rainfall in mm/month, Et is evaporation from the storage tank in mm/month (this was considered as zero because the rainwater reservoirs were closed) and A is the roof area in m2.
where r is the sum of all failure months (when the reservoir runs dry) and n is the total number of months in the simulation period.
The RWH systems were analysed using the mass balance approach. The model was executed in a spreadsheet environment using equations (1), (2) and (3) with consideration of roof area, monthly water consumption and storage. The model development included introduction of the input data and assumptions used in the equations using IF and COUNT IF functions in a spreadsheet environment, and reliability results were recorded for each household. In addition, water balance was calculated to account for deficit and surplus water in each month by modifying the equation used by different scientists (Ward et al. Citation2008).
3 Results
3.1 Rainfall distribution
Mekelle city has a unimodal rainfall distribution () with an average annual rainfall of 544.43 mm. Much of the rain occurs during the wet season of July and August, followed by April, May, June and September, while the remaining months are drier. According to the historical data we gathered from the Ethiopian Meteorological Agency, the highest annual rainfall in the study area between 1998 and 2012 was 755.8 mm, recorded in 2010, and the lowest was 296.7 mm in 2005. The minimum monthly average rainfall for the 15-year period was 0 mm, while the maximum was 300.7 mm.
3.2 Rainwater harvesting system and reliability
The RWH project was implemented with 12 281 residents which accounts for only 4.11% of the total city population. Mekelle city, which is located in a semi-arid region of northern Ethiopia, is experiencing a serious water scarcity problem that forces inhabitants, working with the government and nongovernmental organizations, to use rainwater as an alternative water supply. In this study, we assessed for the first time the contribution of rainwater to the water supply and the reliability of existing RWH systems in the area. From the total of 67 RWH systems that were installed in the city, only 32 were functional during the data collection period (). We gathered social and physical data about all the functional systems and modelled them with 15 years’ worth of historical rainfall data using the mass balance equations. We found about 90.6% of the RWH systems in the study area unreliable, with reliability indexes of less than 70%.
The results revealed that the reliability of the rainwater harvesting systems was affected by family size and monthly water consumption, while storage tank size in the study area was only important in the main rainy season. The main determining factor for RWH reliability was rooftop area. The systems of households with a larger roof area were more reliable than those of households with a smaller roof area. Most households did not use all their available roof area due to the limited size of the gutters provided by project implementers. We found the RWH systems of only three households to have reliability of 70% or above. The difference in reliability between using the existing and potential roof areas is presented in .
Figure 3. Comparative analysis of potential and existing reliability of roof rainwater harvesting systems in Mekelle city, 2014.
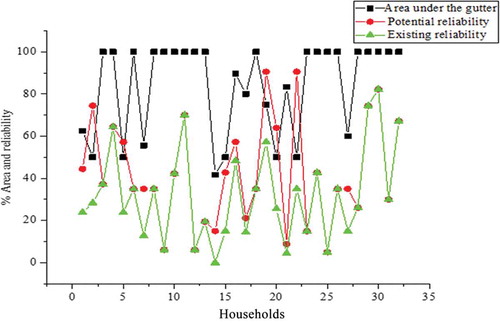
Irrespective of the storage sizes, we found the systems of households with larger roof areas and small average monthly water consumption more reliable than those of households with smaller roof areas and large monthly consumption. For instance, household numbers 4, 10 and 30 as shown in have higher reliability and larger roof areas than the others.
Except during the wet months of July and August and, to some extent, September, almost all analysed households with smaller roof areas had very little or no water in their storage tanks. For instance, the system of household number 1 in could supply only 25% of its water demand (for only three months), which means it needed to supply 75% of its demand from other sources each year.
Households with larger roof areas were better able to supply their demands, despite the low rainfall distribution in the area. For instance, household number 4, with a roof area of 77 m2, could supply sufficient water for its family members for about seven months a year, covering 64.44% of the household demand. Almost all the households need larger tanks to store surplus water from the wet season to cover their demand for the dry months (January–June) without any supplement from other sources ().
4 Discussion
Ethiopia’s safe water access problem claims millions of lives and may continue to claim more in the future. Paradoxically, its water potential is among the highest in sub-Saharan Africa as it has many major river basins with a total annual estimated flow of 122 billion m3 (MoWR Citation1999). According to the Ministry of Water Resources, 80% of the water resources are concentrated in the western region of the country where only 33% of the total population lives. Sixty percent of the population in the eastern part of the country has only 11% share of the total water resources in the country. In agreement with Pratt (Citation1999), the results of this study revealed that the unavailability of water resources is in areas of high demand. In such cases, new water source developments as a supply side is the only solution to meet the water demand. There is growing interest in Asia and Africa of using rainwater as part of an integrated water supply where rural and urban water supply is unreliable and good quality freshwater is lacking. For instance, East African mothers are travelling more than 1.5 km to fetch as little as 10 L of water per capita per day (Thomas Citation1998). In the UK, the average water usage per person per day is reported to be about 150 L (Diaper et al. Citation2001).
Rainwater harvesting is expected to have a significant role in reducing domestic water consumption in the future because of the increasing demand related to demographic and other factors (Diaper et al. Citation2001). In 2012, there were about 7500 RWH systems in sub-Saharan Africa intended to reduce the consumption of domestic water demand (Ward et al. Citation2012). Nevertheless, this was considered low compared to the 50 000 RWH systems installed each year in Germany (Nolde Citation2007). A similar situation exists in New Zealand where it is estimated more than 10% of the population uses RWH for their drinking water (Abbott et al. Citation2007). Rainwater harvesting systems are not yet optimally utilized in Mekelle city. All community members in the city apparently use rainwater as a supplement to the highly mineralized and intermittent groundwater during the wet season just using their roof or natural system. Yet only 67 households had complete RWH systems of traditional design and 47.8% (n = 32) of them were in use at the time of data collection; the rest were either new, not yet in operation or nonfunctional.
Of the residential units with RWH in use in Mekelle city, we found only 3.1% had high reliability, 28.1% medium reliability and 68.7% low reliability, based on the classifications used by Ndomba and Wambura (Citation2010). The household with the highest reliability had a large roof area relative to the size of the family dwelling, whereas the households with the lowest reliability had small roof areas under the gutters for rainwater delivery and relatively high monthly water demand associated with larger family size. A reliability analysis carried out in Sydney peri-urban region has suggested that RWH systems should be designed to achieve high reliability in peri-urban regions to satisfy the required demand by users. Besides, the study stressed design criteria should fit to different locations and household characteristics (Hajani and Rahman Citation2014). However, RWH systems in Mekelle city were designed traditionally, assuming a daily water demand of 20 L/household/day, a family size of five people per household and a storage capacity of 2.5–7.2 m3, while the average family size of the study area is 6.8 and the average daily water consumption is 103.89 L/household/day which is far from the design criteria used by the project implementers.
This finding highlights the great uncertainties over how an adaptation option will work (Adger et al. Citation2005). Hence, projects being implemented must be assessed and noted as the effectiveness will likely depend on an unidentified set of factors (Adger et al. Citation2005). In this instance, roof area was found to be a major factor affecting the reliability of the RWH systems in the study area, with 40.6% of the households having extra roof area not covered under the gutter. This leads to inefficient rainwater harvesting. In agreement with our findings, a water balance analysis in sub-Saharan Africa identified the under-utilization of RWH schemes (Gwenzi and Nyamadzawo Citation2014). This gives rise to a call for water policies in sub-Saharan Africa to be like those in developed countries, so that people will recognize the role of RWH and use it effectively. In Iran, Mehrabadi et al. (Citation2013) assessed the performance of RWH for non-potable water and they estimated the potential of supplying up to 75% of the demand in households having larger roof areas. Hence, if households use the available roof area under the gutter, the percentage of RWH systems with high reliability would increase from 3% to 9.3% and those with medium reliability to 37.5% without considering the other factors.
Although increased storage size was strongly recommended by researchers (Ndomba and Wambura Citation2010, Hajani and Rahman Citation2014) for greater reliability of rainwater harvesting systems, in this study it was a major factor only during wet or rainy periods. This could be due to the low rainfall distribution in the study area, with 544.43–755.8 mm average annual rainfall occurring unimodally during the wet season. We found storage to be important only during the wet season. Based on the water balance calculation, surplus water was recorded for the months of July–September in most households.
In general, to increase the reliability of rainwater harvesting systems, either the roof area should be increased to harvest enough water during the dry season, or the tank size should be increased to store the surplus rainwater from the wet season for the coming dry season. A case study which was conducted on RWH systems also showed that increasing surface area resulted in better reliability (Aladenola and Adeboye Citation2010). Arguably, the role of RWH remains ambiguous in the semi-arid areas of sub-Saharan Africa, such as Ethiopia, where research outputs and means of communication facilities are in their infancy.
5 Conclusion
In developed countries, rainwater harvesting (RWH) is mostly used to save water and as a main water supply for households in the water-scarce region. Rainwater harvesting systems are not yet well developed in sub-Saharan Africa, but are mainly used as a main water supply source because of the poor infrastructure and the physical inaccessibility of the existing water sources. For effective and efficient use of RWH, we investigated the reliability of complete roof rainwater harvesting systems used by households in the semi-arid city of Mekelle using a mass balance approach. We used 15 years’ historical monthly average rainfall as inputs. The analysis revealed that the design of the roof RWH systems in the city failed to take into account family size, water demand, rooftop area, and volume of storage tank, and the current traditional RWH system could not meet the average water demand of households. Percent potential reliability analysis of roof RWH systems showed achievable reliability of 82.2% using the existing system in a given household. The reliability could be increased to a maximum of 90.56% if households used all their roof area under the gutter without changing the volume of the tank. To make RWH system projects reliable and sustainable, the rainfall, physical and social factors of the area should be considered and investigated in detail at pilot study scale before large-scale implementation. Some of the households can increase the reliability of their existing systems if they include gutters for the entire roof. The cost would be about US$ 12.40 for 6 m of PVC gutter. As an alternative, increasing the storage tank size would enable the surplus water to be stored during the wet season so that the existing systems can supply enough water in the dry seasons.
Acknowledgements
The authors are indebted to Joy Larson, the coordinator of UCONN and Addis Ababa University link, for all her support. Mekelle City Administration also deserves acknowledgment for the letter of support given during the time of data collection.
Disclosure statement
No potential conflict of interest was reported by the authors.
Additional information
Funding
References
- Abbott, S., Caughley, B., and Douwes, J., 2007. The microbiological quality of roof-collected rainwater of private dwellings in New Zealand. In: Rainwater and Urban Design Conference, 21–23 August, Sydney.
- Adger, W.N., Arnell, N.W., and Tompkins, E.L., 2005. Successful adaptation to climate change across scales. Global Environmental Change, 15 (2), 77–86. doi:10.1016/j.gloenvcha.2004.12.005
- Aladenola, O.O. and Adeboye, O.B., 2010. Assessing the potential for rainwater harvesting. Water Resources Management, 24 (10), 2129–2137. doi:10.1007/s11269-009-9542-y
- Basnet, G., 2013. Human rights. Asian Journal of International Law, 3 (1), 193–194. doi:10.1017/S2044251312000227
- Cowden, J.R., Watkins, D.W., and Mihelcic, J.R., 2008. Stochastic rainfall modeling in West Africa: Parsimonious approaches for domestic rainwater harvesting assessment. Journal of Hydrology, 361 (1–2), 64–77. doi:10.1016/j.jhydrol.2008.07.025
- Diaper, C., et al., 2001. Water-Recycling Technologies in the UK. Water and Environment Journal, 15 (4), 282–286. doi:10.1111/j.1747-6593.2001.tb00355.x
- Durham, B., Rinck-Pfeiffer, S., and Guendert, D., 2003. Integrated water resource management — through reuse and aquifer recharge. Desalination, 152 (1–3), 333–338. doi:10.1016/S0011-9164(02)01081-0
- EMA, 1981. Atlas of Ethiopia. Addis Ababa, Ethiopia: Ethiopian Mapping Agency (EMA).
- Falkenmark, M., Lundqvist, J., and Widstrand, C., 1989. Macro-scale water scarcity requires micro-scale approaches. Aspects of vulnerability in semi-arid development. Natural Resources Forum, 13 (4), 258–267. doi:10.1111/narf.1989.13.issue-4
- Fewkes, A., 2004. The Modelling and Testing of a Rainwater Catchment System in the UK. Thesis (PhD). The Nottingham Trent University.
- Frot, E., et al., 2008. Water harvesting potential in function of hillslope characteristics: A case study from the Sierra de Gador (Almeria province, south-east Spain). Journal of Arid Environments, 72 (7), 1213–1231. doi:10.1016/j.jaridenv.2007.12.009
- Gebremedhin, B., 2002. Engineering Geological Investigation of Mekelle area, Tigray, Northern Ethiopia. Thesis (MSc). Addis Ababa University.
- Ghisi, E., Bressan, D.L., and Martini, M., 2007. Rainwater tank capacity and potential for potable water savings by using rainwater in the residential sector of southeastern Brazil. Building and Environment, 42 (4), 1654–1666. doi:10.1016/j.buildenv.2006.02.007
- Gould, J. and Nissen-Petersen, E., 1999. Rainwater catchment systems for domestic supply: design, construction and implementation. London, UK: Practical Action.
- Gwenzi, W. and Nyamadzawo, G., 2014. Hydrological impacts of urbanization and urban roof water harvesting in water-limited catchments: a review. Environmental Processes, 1 (4), 573–593. doi:10.1007/s40710-014-0037-3
- Hajani, E. and Rahman, A., 2014. Reliability and cost analysis of a rainwater harvesting system in peri-urban regions of greater Sydney, Australia. Water, 6 (4), 945–960. doi:10.3390/w6040945
- Imteaz, M.A., Ahsan, A., and Shanableh, A., 2013. Reliability analysis of rainwater tanks using daily water balance model: Variations within a large city. Resources, Conservation and Recycling, 77 (0), 37–43. doi:10.1016/j.resconrec.2013.05.006
- Kim, Y. and Han, M., 2006. A Rainfall-Storage-Runoff (RSR) model for the design of a rainwater tank effective for flow control in urban drainage pipes. In: Proceedings of the 2nd IWA international rainwater harvesting workshop, 11 September, Beijing.
- Lall, U., Rajagopalan, B., and Tarboton, D.G., 1996. A nonparametric wet/dry spell model for resampling daily precipitation. Water Resources Research, 32 (9), 2803–2823. doi:10.1029/96WR00565
- Liaw, C.H., et al., 1997. Developmentof urban rainwater cistern systems technology. Engineering Science and Technology Bulletin NSC, (26), 75–78.
- Mehrabadi, M.H.R., Saghafian, B., and Fashi, F.H., 2013. Assessment of residential rainwater harvesting efficiency for meeting non-potable water demands in three climate conditions. Resources, Conservation and Recycling, 73, 86–93. doi:10.1016/j.resconrec.2013.01.015
- MoWR, 1999. Water sector development program. Addis Ababa, Ethiopia: Ministry of Water Resources (MoWR.
- Ndomba, P.M. and Wambura, F.J., 2010. Reliability of rainwater harvesting systems in suburbs. A case study of Changanyikeni in Dares Salaam, Tanzania. 11th WaterNet /WARFSA/ GWP-SA Symposium, Victoria Falls, Zimbabwe.
- Ngigi, S.N., 2009. Climate change adaptation strategies, water resources management options for smallholder farming systems in sub-Saharan Africa. New York: The MDG centre for east and Southern Africa of the Earth Institute at Columbia University.
- Nolde, E., 2007. Possibilities of rainwater utilisation in densely populated areas including precipitation runoffs from traffic surfaces. Desalination, 215 (1), 1–11. doi:10.1016/j.desal.2006.10.033
- Pajankar, S. and Rode, P., 2012. Right to water: a human rights perspective. Asia Pacific Journal of Management & Entrepreneurship Research, 1 (1), 156–164.
- Parry, M.L., et al., 2007. Contribution of working group II to the fourth assessment report of the intergovernmental panel on climate change, 2007. Cambridge, UK: Cambridge University Press.
- Pratt, C.J., 1999. Use of permeable, reservoir pavement constructions for storm water treatment and storage for re-use. Water Science and Technology, 39 (5), 145–151. doi:10.1016/S0273-1223(99)00096-7
- Rijsberman, and Frank, R., 2006. Water scarcity: fact or fiction? Agricultural Water Management, 80 (1–3), 5–22. doi:10.1016/j.agwat.2005.07.001
- Roebuck, R.M. and Ashley, R.M., 2006. Predicting the hydraulic and life-cycle cost performance of rainwater harvesting systems using a computer based modelling tool. In: A. Delectic and T. Fletcher, eds. Proceedings of the 7th international conference on urban drainage and 4th international conference on water sensitive urban design, Vol. 2, 2–7 April, Melbourne, 699–706.
- Sazakli, E., Alexopoulos, A., and Leotsinidis, M., 2007. Rainwater harvesting, quality assessment and utilization in Kefalonia Island, Greece. Water Research, 41 (9), 2039–2047. doi:10.1016/j.watres.2007.01.037
- Thomas, T., 1998. Domestic water supply using rainwater harvesting. Building Research & Information, 26 (2), 94–101. doi:10.1080/096132198370010
- UN, 1977. Report of the United Nations (UN) Water Conference, Mar del Plata. March 14-25, 1977. No. E.77.II.A.12. New York, USA.
- Vaes, G. and Berlamont, J., 2001. The effect of rainwater storage tanks on design storms. Urban Water, 3 (4), 303–307. doi:10.1016/S1462-0758(01)00044-9
- Ward, S., Memon, F.A., and Butler, D., 2008. Rainwater harvesting: model-based design evaluation. In: 11th international conference on urban drainage, 31 August–5 September, Edinburgh. HR Wallingford.
- Ward, S., Memon, F.A., and Butler, D., 2012. Performance of a large building rainwater harvesting system. Water Research, 46 (16), 5127–5134. doi:10.1016/j.watres.2012.06.043
- WHO/UNICEF, 2014. Joint monitoring programme for water supply and sanitation report of 2014 [online]. Switzerland: World Health Organization (WHO) & UNICEF. Available from: http://www.unicef.org/Progress_on_drinking_water_and_sanitation_2014_update.pdf [Accessed 11 August 2014].
- Yusuf, F.M., 1999. Rainwater Harvesting Potential in Bangladesh. Thesis (MSc). Bangladesh University of Engineering and Technology.