ABSTRACT
The aim of the study was to locate and describe groundwater outflows in a selected lake basin and measure the spring output of water and the physical characteristics of the studied waters (temperature and conductivity). The final aim was to gauge the effects of the spring output on the lake hydrology. The time scope of the work included the period from January 2011 to September 2012. The spatial scope of the work included the area of Lake Raduńskie Górne and its direct catchment located in the Kashubian Lake District in northern Poland. Four groundwater outflows were located in the course of the study. Their total output was 4.6 L s−1 and ranged between 0.5 and 2.5 L s−1. This produces an annual yield of about 145 000 m3 year−1. These results confirm that one of the forms of supply to the lakes are the under-lake springs. For lakes located in the area of young glacial accumulation this can be decisive.
Editor D. Koutsoyiannis Associate editor D. Gerten
1 Introduction
Lakes are important bodies of water as they are natural reservoirs which determine water circulation patterns in a drainage basin. Lakes may have both drainage and resupply characteristics. Unfortunately, the identification of water balance may pose a number of problems arising from a lack of information on lakes’ underground water supply. This is due to poor hydrogeological analysis of the geographic areas where the studied lakes are located as well as a lack of appropriate monitoring networks. Therefore, the most common method designed to evaluate the size of the groundwater contributing area of a lake consists of the assumption that underground inflow and outflow are equal, or an approximation of its value calculated from the water balance difference (Choiński Citation2007).
One means of underground lake recharge consists of concentrated surface outflow resulting from the merger of several streams flowing from their springs. Another means of recharge consists of water supplied by a spring located within the lake basin itself – some springs can be submerged at high water levels. Yet another form of lake groundwater supply is via the bottom of the lake basin (intersection of aquifers, springs) (Shanks and Callender Citation1992). There is little literature on the number and yield of groundwater outflows and springs in lake bottoms. The most important is the work of Harvey et al. (Citation2000), in which the authors estimate the volume of groundwater flow in large lakes. Another work on the subject is that of Choiński et al. (Citation2008), in which the authors discuss, using examples of lakes in Poland, recharge volume for lakes via springs located at the bottom of lake basins. In addition, Choiński and Ptak (Citation2012) discuss differences in the thickness of ice cover on Lake Samołęskie resulting from groundwater recharge supply. Yihdego and Becht (Citation2013) discuss using the DEM model and three-dimensional flow model to simulate the impact of manmade underground Lake Naivasha in Kenya. Yihdego and Webb (Citation2015) use a conceptual hydrogeological model to discuss changes in water supply and salinity in Lake Burrumbeet in southeastern Australia and model seasonal and long-term trends in lake salinity (Yihdego and Webb Citation2012).
Papers that cover underwater groundwater seepage also cover seepage exchange in floodplain lakes (Lesack Citation1995) as well as the characterization of lake water and groundwater movement in the littoral zone (Schuster et al. Citation2003). The latter concerns practical methods of analysing such seeps. The two papers discuss not only lakes but also seas and coastal areas. In addition, Mortimer et al. (Citation1999) discuss the use of a high-resolution pore-water gel profiler to measure groundwater fluxes at an underwater saline seepage site. Nyquist et al. (Citation2008) discuss stream bottom resistivity tomography designed to map groundwater discharge sites. Taniguchi et al. (Citation2002) discuss underwater groundwater discharge. Tcherepanov et al. (Citation2005) discuss Landsat thermal imagery as well as GIS for the identification of groundwater discharge. Duncan et al. (Citation2006) describe the adaptation of groundwater evaluation and sampling tools for underwater use. Finally, Sauter et al. (Citation2003) discuss AUV multi-technique surveying of underwater freshwater seeps.
The aim of this study was to locate and describe groundwater outflows in a selected ribbon lake basin. The second aim was to measure spring output and measure physical characteristics of the studied waters (temperature, conductivity). The second part of the paper makes an attempt to compare the research results with those for other lakes in the world. The final aim was to gauge the effects of the spring output on the hydrology of the studied lake. The main hypothesis assumed that the research literature contains information that one of the forms of lake water supply is through groundwater outflows. It was also assumed that the lakes of the Kashubian Lake District are characterized by such a form of lake water supply.
The research was performed between January 2011 and September 2012. The study area consisted of the northeastern part of Lake Raduńskie Górne which is located in northern Poland in the Kashubian Lake District.
2 Methods
The first step consisted of consultation with individuals with potential knowledge of where to find under-lake springs.
In early February 2011, consultation with Dr. J. Dworniczak from the Department of Geomorphology and Quaternary Geology at the University of Gdańsk indicated that Lake Raduńskie Górne was being measured using a seismoacoustic probe. As a result, a seismoacoustic chart was obtained (), where at one point an irregularity in the bottom of the water body was recorded. It was considered that the layer of sediment at the bottom was discontinuous, which might be a potential place for a water outflow. This site is located on a slope at a depth of about 37 m. The location of this site makes it very difficult to study and thus its analysis was abandoned.
Underwater vegetation indicating the occurrence of springs at the bottom of the basin of Lake Raduńskie Górne was described by Dr. K. Bociąg from the Department of Plant Ecology at the University of Gdańsk in the following manner: “… at a depth of 6–8 m, the water was visibly clearer than anywhere else (less suspension, including plankton) and cooler. At this transect, underwater vegetation was recorded deeper than in other places of the lake. The substrate in the area was sandy-gravelly. The only reasonable explanation for this was groundwater supply in this area.
On the basis of the above information, Lake Raduńskie Górne was selected for further study. This lake was selected for analysis due to its significant morphological differentiation with a sequence of alternating permeable and impermeable deposits, as well as discontinuity of aquifers and variable aquifer thickness. Another key factor conducive to the occurrence of groundwater outflows is the presence of a perched water table. Another main consideration regarding the choice of this lake was its water balance calculated by Okulanis (Citation1981), in which he proved that the underground water supply in the case of this lake is very large. The significance of its underground water supply is also indicated by the relative stability of its water table. The lake’s water table fluctuates only a few centimetres per year.
Further studies on the selected lake were carried out in the field. First, a test polygon was selected (). Research methods aimed at gathering and studying source materials and maps. Cartographic materials were analysed with the use of MapInfo Professional 9.5. The purpose of fieldwork was to find groundwater outflows in the basin of Lake Raduńskie Górne. During the field research, diving was carried out in the lake. During each dive, audiovisual documentation was created using a camera (Nikon D90) with an underwater case (Ikelite Nikon D90) and a flash (Ikelite DS 161 movie) as well as a GoPro HD HERO 2 Outdoor camera. The first dives were performed on 28 and 29 January 2012. The next dives were performed on 23 March 2012, 18 April 2012 and 6 September 2012. A bathymetric map was generated using an Echosound FISHFINDER 300C made by the Garmin company as well as GPS ETRAX LEGEND, also made by Garmin.
Water temperature and conductivity were measured using a multiparameter 350 metre made by WTW in order to determine if a groundwater outflow at the bottom of the lake has been detected. These measurements were performed during the winter and summer. In addition, approximated measurements of spring yield were performed. This was done through the imposition of timbering on the spring and measurement of the amount of water flowing at a given time.
3 Surface water
Lake Raduńskie Górne is part of the complex of Raduńsko-Ostrzyckie Lakes, the total water surface area of which is 21.65 km2. Hydrographically, these lakes are located in the upper reaches of the catchment of the Radunia River. On the one hand, they serve as the main source of water supply for the Radunia and on the other, they create a natural complex of retention reservoirs (Okulanis Citation1981). The drainage structure of the upper Radunia catchment includes 15 water bodies with a total length of 48.1 km. The series of these water bodies is connected with short inter-lake links. The characteristic internal linkage of the area and line of bodies of water in a consistent surface water drainage system mean it can be defined as a fluvial-lacustrine system.
The surface water table of Lake Raduńskie Górne is 380.5 ha in area, of which 0.7 ha are occupied by two islands: one is located in the centre, while the other is in the northern part of the lake. The shoreline development ratio is 2.44.
The relief of the bottom of the entire lake is very diverse and has many shallow and deep parts. The largest deep reaches 43 m and is located in the middle of the lake. This depth makes Lake Raduńskie Górne the deepest water body in the catchment of the upper Radunia, also in terms of the average depth (15.5 m) ().
4 Groundwater
The shallowest level of groundwater in this area is in the river valleys and in the vicinity of water bodies. In general, its depth does not exceed 1 m. Shallow groundwater is also found in wetlands in depressions, underlain by poorly permeable sediments.
The only aquifer in the research area is a Quaternary aquifer (Polish Hydrogeological Map; scale 1: 50 000, Stężyca map sheet (52), Citation2000). Its outwash level is supplied directly by infiltration. It is drained by water bodies and small watercourses. The ceiling of the outwash level is found at depths from 10 m to 25 m. In depressions, this depth is less than 5 m. The average rate of infiltration is 43.2 m d−1, up to 99.4 m d−1. The free water table is tilted towards the south and stabilizes at an elevation of about 160 m a.s.l. (Polish Hydrogeological Map; scale 1: 50 000, Stężyca map sheet (52), Citation2000).
The first inter-moraine aquifer occurs in subglacial channels. In the lakes’ vicinity, it is badly isolated. In channels, the aquifer’s depth is less than 10 m, and in the plateau area, it is 50 m. This aquifer is characterized by a high rate of infiltration ranging from 20 to 40 m d−1, max, up to 84 m d−1. The depth of the second inter-moraine level depends on the ground surface elevation. In the area of moraine peaks, it is more than 100 m, and in other areas, it is in the range of 50–100 m (). The rate of infiltration is in the range of 5–25 m d−1. The thickness is from 15 to 40 m ().
Figure 4. Depth of occurrence of the main aquifer (Polish Hydrogeological Map; scale 1: 50 000, Stężyca map sheet (52)).
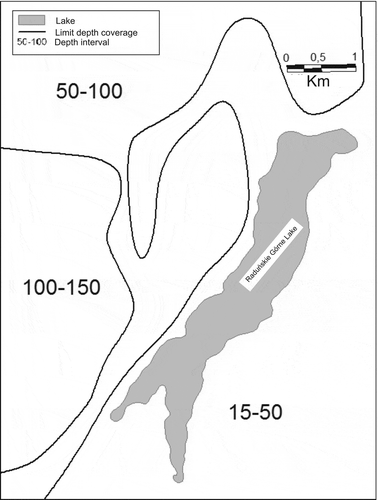
Figure 5. Thickness of the main aquifer (Polish Hydrogeological Map; scale 1: 50 000, Stężyca map sheet (52)).
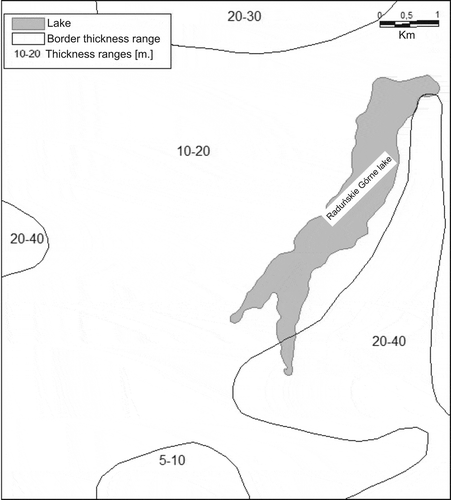
Analysis of local hydrogeological conditions has shown that between the outwash and inter-moraine levels there is local groundwater flow which directs shallow water circulation patterns. It is likely that water in the deeper layers of Aquifer II is indirectly involved in that movement of water. Almost all of the analysed area serves as a supply area for the regional water circulation system. These considerations indicate that in the catchment area of the upper Radunia, two of the mentioned aquifers are drained.
5 Results
During the dive on 29 January 2012, at a depth of about 6 m, a groundwater outflow was detected at the following coordinates: 54° 14' 45.9'' N, 17° 59' 06.6'' E ().
The bottom of the dive site was sandy and sandy-gravelly with scattered groups of different types of shells. The sand forming the bottom of the lake was dark because of the covering layer of sediment. The immediate vicinity of the outflow at the bottom of the lake, however, was characterized by white patches of fresh sand (). A few such points with lighter-coloured sand were found. These could be places of groundwater outflow, which in the winter are less productive and disappear until the groundwater level increases. As calculated by Okulanis (Citation1981) and other researchers, the underground supply is larger in summer than in winter. Such a situation may be suggested by the light colour of the sand extending from the cone at the water outflow towards the lake’s deep.
The site of the water outflow is a characteristic cone of light sand () with a depression in the middle. Such a cone was located on a steep slope. The force of gravity was likely to have caused the extension of the cone and the increase in the area covered with light sand in the direction of the slope. The approximate dimensions of the cone were: length 70 cm, width 25 cm, height 7 cm, length of the cone’s dome 25 cm, length at which sand is sprinkled 45 cm and the diameter of the hole from which the groundwater flows out 8 cm.
On 18 April 2012, yet another field study in the research area was undertaken. Measurements were conducted to create a bathymetric map of the research area (). It shows a dense network of lake bottom contours which reflect steep underwater slopes.
Figure 8. Bathymetry of the research area with location of all lake floor springs (I-IV) and an unidentified depression, A (lines indicate contours in metres).
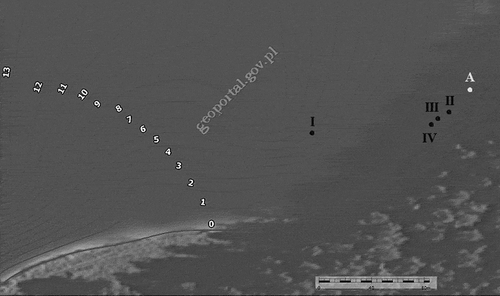
Other groundwater outflow sites were found in the course of the dive in the study area. As before, outflow sites had light (fresh) sand. Due to a lack of equipment for underwater exploration, only photographic documentation from the water level was created. Three unique groundwater outflows were observed. In terms of their structure, they resembled the first groundwater outflow, but in these additional cases, outflow cones were posed symmetrically around the point from where the groundwater was coming out, which was due to the small depth at which these outflows were located and, as a result of, a flat lake floor. The outflows were located at a depth of 0.5–0.7 m, and the bottom slope began at around 1 m of depth. Due to the different characteristics of the location of these outflows, different names for this group of three outflows were suggested. For example, these outflows can be designated “under-lake offshore springs”. These sites are located at the coordinates of 54° 14' 46.8'' N, 17° 59' 08.6'' E. Similarly to the first site, these three offshore outflows display light-coloured sand, which proves its “freshness”, and a distinctive round shape of the cone (). The approximate dimensions of the first of the offshore outflows were: 45 cm wide and 45 cm long. The next two outflows were slightly smaller. The second was 12 cm wide and 20 cm long. The third outflow was 20 cm in width and 30 cm in length. The heights of the outflow cones were about 5 cm. The sites of all three outflows are shown on the bathymetric map (I-IV) ().
All the four studied springs have low yields and small range of several metres. The yield of spring no. 1 is about 2.5 L s−1. The yield of the other three springs ranges from 0.5 to 1.0 L s−1 (research performed 29 January 2012). Water temperature in the springs ranges from 6.8 to 7.4°C. This results in a warming of the neighbouring zone; the surface temperature was between 0.4 and 0.7°C. In turn, in the summer, it caused cooling of the neighbouring zone. At this time, the temperature of the outflowing water varied from 6.5 to 7.0°C, while that of the surface water was between 18.2 and 19.3°C. Variable results were also observed in terms of the value of conductivity. In the outflow zones, its value ranged from 300 to 320 µS cm−1 and with growing distance from the springs, it decreased to a range of 250–280 µS cm−1. A summary of yields and the physical properties of water are shown in .
Table 1. Yield and physical properties of groundwater flowing out in the bottom of the basin of Lake Raduńskie Górne.
6 Discussion
The research literature clearly states that many bodies of water including lakes with a varied basin evolution and located across a variety of landscapes are recharged underground, which often has an effect on the recharge total. In regional water systems, complex interactions between groundwater and surface water have been detected regardless of lake topographic location. The main causes of complex and seasonally quite dynamic changes in groundwater recharge are hydrologic processes observed at the lake surface including changes in lake water level, rate of evaporation, and rate of transpiration (Winter Citation1999).
Lake groundwater recharge is most often determined based on the water balance and changes in lake water levels in comparison with a fixed rate of output and surface area of the water table. Many lakes obtain most of their water from atmospheric precipitation and lose the most water via evaporation. However, groundwater influx is significant in the case of some lakes. For example, Lake Purrumbete in southern Australia obtains about 17% of its water from underground aquifers (Yihdego et al. Citation2014a). A lake can also lose up to 30% of its water balance via underground outflow, one example of which is Lake Burrumbeet in southern Australia (Yihdego et al. 2015). Okulanis (Citation1981) estimates that Lake Raduńskie Górne obtains about 28% of its water from underground sources and loses about 10% in the same way.
One outcome of significant under-lake recharge is fewer fluctuations in lake water level in relation to lakes with negligible water influx from under-lake sources. Another outcome is control over lake salinity levels by groundwater and surface water sources, which is observed in Lake Purrumbete. The low salinity of the lake reflects a significant loss of salt content via groundwater and surface water outflow (Yihdego et al. Citation2014b).
What is very important in geographic areas characterized by a balance of atmospheric precipitation and evaporation or precipitation exceeding evaporation is that the period with evaporation from a lake’s surface is also the period triggering under-lake groundwater influx. Lake Raduńskie Górne is located in this type of geographic area. Influx is also determined by the size of the lake and its ambient climate zone (Smerdon et al. Citation2005). It appears that there exists a very dynamic relationship between atmospheric precipitation and groundwater influx to lakes. The interactions between these two recharge mechanisms potentially conceal the role of human impact behind the veil of natural variances (Smerdon et al. Citation2005).
While there are numerous papers on recharge, there exist few papers on the number of lake floor springs and their output. Researchers who identify springs focus on those located above the water line or in areas only periodically covered by water. Smerdon et al. (Citation2005) write about springs of this type for a shallow lake located in Canada and fed by 16 permanent springs. It is the case that 15 such outflows are to be found around Lake Raduńskie Górne and their total output is 13.8 L s−1. There is a dearth of papers on springs found across the lake floor and covered with water year-round. This is due to the difficulty in studying such springs, which are often found at depths of more than ten metres, and due to the need for specialized equipment required to study these types of springs.
A lake with several lake floor springs is Lake Kobyleckie in Poland. Choiński (Citation2007) established that one of these springs yields several litres per second. Another lake with floor springs is Lake Kielskie, also in Poland. Its springs yield two litres per second. A third lake with lake floor springs is Lake Wapienne in Poland with spring output of one litre per second (Choiński et al. Citation2008). The total output of floor springs in Lake Raduńskie Górne is 4.6 litres per second. This translates into 145 000 m3 of water per year or about 0.24% of total volume given that the total volume of the lake is 82.5 million m3. On the other hand, the share of the lake floor springs in total recharge in Lake Raduńskie Górne is about 0.15%.
Total groundwater influx to Lake Raduńskie Górne is close to 97 million m3. Hence, the contribution of lake floor springs is negligible in relation with total groundwater influx. It has to be assumed that the number of lake floor springs is actually much larger than what has been discovered in the course of this study. In effect, the contribution made by such springs is actually much larger. Hypothetically speaking, if all groundwater recharge originated in lake floor springs and spring output were similar to that noted in this study, then the number of lake floor springs would be closer to 2500.
However, this is not a viable scenario, as springs located around the edge of the lake also contribute water to the lake. A small incision in the water-bearing horizons found below the floor of the lake also contributes water to the lake. In addition, another key determinant of groundwater recharge is soil permeability in a lake’s drainage basin.
San Perez (Citation1996) notes that highly permeable formations are the key determinant in Spain, determining more than 94% of groundwater recharge. Lake size and geometry are two other relevant factors (Townley and Trefry Citation2000). The morphometry of the direct drainage basin is also important (Zecharias and Brutsaert Citation1988). On the other hand, according to Smith and Townley (Citation2002), interactions between surface water and groundwater depend on a lake’s position in the regional lake and river system. Changes in groundwater recharge of lakes yield changes in the water balance, shifts in rates of change in the total water supply, fluctuations in surface water levels, changes in the way bodies of water are connected (Sophocleous Citation2002) and changes in water quality (Li and Wang Citation2007, Dash et al. Citation2008).
A number of studies conducted in the course of our research project made it possible to confirm our research hypothesis. One presumed form of lake recharge is the influx of groundwater. Lakes of the Kashubian Lake District show this kind of recharge. Underground water supply occurs through under-lake springs.
Four lake floor groundwater outflows were found in the course of the research. In order to examine these springs, audiovisual and photographic documentation was created. To systematize the typology of the discovered springs, new nomenclature was suggested, namely “under-lake springs” with two subtypes: (1) under-lake slope spring, (2) under-lake offshore spring ().
Table 2. Summary of all springs found under-lake in Lake Raduńskie Górne.
The study has confirmed the potential significance of groundwater outflows found across lake floors in lakes’ overall water supply. This is especially visible in young glacial areas affected by sheets of ice in the past as well as water resulting from the melting of ice. Unique geology and local hydrography favour the occurrence of such outflows. Under-lake water recharge does affect the supply of water in a lake as well as providing greater stability and new water that can refresh water chemistry in one part of the lake or throughout the given lake. In this study, this was measured using water conductivity. In short, groundwater outflows located on the lake floor, also known as under-lake springs, are significant in the functioning as well as the duration of many lakes around the world.
Disclosure statement
No potential conflict of interest was reported by the authors.
References
- Choiński, A., 2007. Physical limnology. Poznań: University of Poznań Publishing House (in Polish).
- Choiński, A., et al., 2008. Lakes supplied by springs: selected examples. Limnological Review, 8 (4), 145–150.
- Choiński, A. and Ptak, M., 2012. Variation in the ice cover thickness on Lake Samołęskie as a result of underground water supply. Limnological Review, 12 (3), 133–138. doi:10.2478/v10194-012-0053-5
- Dash, R.R., et al., 2008. Lake bank filtration at Nainital, India: water-quality evaluation. Hydrogeology Journal, 16, 1089–1099. doi:10.1007/s10040-008-0295-0
- Duncan, P.B., et al., 2006. Adaptation of groundwater evaluation and sampling tools for underwater deployment [ online]. American Academy of Underwater Sciences. Available from: http://www.aaus.org/ [Accessed 3 2012].
- Harvey, F.E., Rudolph, D.L., and Frape, S.K., 2000. Estimating groundwater flux into large lakes: Application in the Hamilton harbor, Western Lake Ontario. Groundwater, 38, 550–565. doi:10.1111/gwat.2000.38.issue-4
- Lesack, L.F.W., 1995. Seepage exchange in an Amazon floodplain lake. Limnology and Oceanography, 40 (3), 598–609. doi:10.4319/lo.1995.40.3.0598
- Li, Y. and Wang, C., 2007. Theoretical estimation of groundwater discharge and associated nutrient loading to a lake with gentle slope bottom. Journal of Hydrodynamics, 19 (1), 30–35. doi:10.1016/S1001-6058(07)60024-9
- Mortimer, R.J.G., et al., 1999. Use of a high-resolution pore-water gel profiler to measure groundwater fluxes at an underwater saline seepage site in Lake Kinneret, Israel. Limnology and Oceanography, 44 (7), 1802–1809. doi:10.4319/lo.1999.44.7.1802
- Nyquist, J.E., Freyer, P.A., and Toran, L., 2008. Stream bottom resistivity tomography to map ground water discharge. Groundwater, 46 (4), 561–569. doi:10.1111/gwat.2008.46.issue-4
- Okulanis, E., 1981. Limnological study of the Lake Raduńsko – Ostrzyckie. Gdańsk: GTN in Polish.
- Polish Hydrogeological Map in scale 1: 50 000, Stężyca map sheet (52), 2000. Gdańsk: PIG.
- San Perez, E., 1996. Springs in Spain: classification according to their flows and lithologies and their hydraulic contributions. Groundwater, 34 (6), 1033–1041. doi:10.1111/gwat.1996.34.issue-6
- Sauter, E., et al., 2003. AUV multi-technique surveying of submarine freshwater seeps - high and ultra-high resolution acoustic and CTD mapping of pockmark sites in Eckernförde Bay, Western Baltic Sea. Sea Technology, 44 (3), 49–52.
- Schuster, P.F., et al., 2003. Characterization of lake water and ground water movement in the littoral zone of Williams Lake, a closed-basin lake in north central Minnesota. Hydrological Processes, 17 (4), 823–838. doi:10.1002/hyp.1211
- Shanks III, W.C. and Callender, E., 1992. Thermal springs in Lake Baikal. Geology, 20, 495–497. doi:10.1130/0091-7613(1992)020<0495:TSILB>2.3.CO;2
- Smerdon, B.D., Devito, K.J., and Mendoza, C.A., 2005. Interaction of groundwater and shallow lakes on outwash sediments in the sub-humid Boreal Plains of Canada. Journal of Hydrology, 314 (1–4), 246–262. doi:10.1016/j.jhydrol.2005.04.001
- Smith, A.J. and Townley, L.R., 2002. Influence of regional setting on the interaction between shallow lakes and aquifers. Water Resources Research, 38 (9), 10–13. doi:10.1029/2001WR000781
- Sophocleous, M., 2002. Interactions between groundwater and surface water: the state of the science. Hydrogeology Journal, 10 (1), 52–67. doi:10.1007/s10040-001-0170-8
- Taniguchi, M., et al., 2002. Investigation of submarine groundwater discharge. Hydrological Processes, 16 (11), 2115–2129. doi:10.1002/(ISSN)1099-1085
- Tcherepanov, E.N., Zlotnik, V.A., and Henebry, G.M., 2005. Using Landsat thermal imagery and GIS for identification of groundwater discharge into shallow groundwater dominated lakes. International Journal of Remote Sensing, 26 (17), 3649–3661. doi:10.1080/01431160500177315
- Townley, L.R. and Trefry, M.G., 2000. Surface water-groundwater interaction near shallow circular lakes: flow geometry in three dimensions. Water Resources Research, 36 (4), 935–948. doi:10.1029/1999WR900304
- Winter, T.C., 1999. Relation of streams, lakes, and wetlands to groundwater flow systems. Hydrogeology Journal, 7 (1), 28–45. doi:10.1007/s100400050178
- Yihdego, Y. and Becht, R., 2013. Simulation of lake–aquifer interaction at Lake Naivasha, Kenya using a three-dimensional flow model with the high conductivity technique and a DEM with bathymetry. Journal of Hydrology, 503, 111–122. doi:10.1016/j.jhydrol.2013.08.034
- Yihdego, Y., Danis, C., and Paffard, A., 2015. 3–D numerical groundwater flow simulation for geological discontinuities in the Unkheltseg Basin, Mongolia. Environmental Earth Sciences. doi:10.1007/s12665-014-3697-4
- Yihdego, Y. and Webb, J., 2012. Modelling of seasonal and long-term trends in lake salinity in South-western Victoria, Australia. Journal of Environmental Management, 112, 149–159. doi:10.1016/j.jenvman.2012.07.002
- Yihdego, Y., Webb, J., and Leahy, P., 2014b. Modelling water and salt balances in a deep, groundwater-through flow lake - Lake Purrumbete, southeastern Australia. Hydrological Sciences Journal. doi:10.1080/02626667.2014.975132
- www.geoportal.gov.pl [Accessed 5 August 2013].
- Yihdego, Y. and Webb, J.A., 2015. Use of a conceptual hydrogeological model and a time variant water budget analysis to determine controls on salinity in Lake Burrumbeet in southeast Australia. Environmental Earth Sciences Journal, 73 (4), 1587–1600. doi:10.1007/s12665-014-3509-x
- Yihdego, Y., Webb, J.A., and Leahy, P., 2014a. Modelling of lake level under climate change conditions: Lake Purrumbete in southeastern Australia. Environmental Earth Sciences Journal. doi:10.1007/s12665-014-3669-8
- Zecharias, Y.B. and Brutsaert, W., 1988. The influence of basin morphology on groundwater outflow. Water Resources Research, 24 (10), 1645–1650. doi:10.1029/WR024i010p01645