ABSTRACT
This paper shares an early-career perspective on potential themes for the upcoming International Association of Hydrological Sciences (IAHS) Scientific Decade (SD). This opinion paper synthesizes six discussion sessions in western Europe identifying three themes that all offer a different perspective on the hydrological threats the world faces and could serve to direct the broader hydrological community: “Tipping points and thresholds in hydrology,” “Intensification of the water cycle,” and “Water services under pressure.” Additionally, four trends were distinguished concerning the way in which hydrological research is conducted: big data, bridging science and practice, open science, and inter- and multidisciplinarity. These themes and trends will provide valuable input for future discussions on the theme for the next IAHS SD. We encourage other early-career scientists to voice their opinion by organizing their own discussion sessions and commenting on this paper to make this initiative grow from a regional initiative to a global movement.
Editor A. Castellarin Associate Editor H. Kreibich
Introduction
The International Association of Hydrological Sciences (IAHS) Scientific Decades (SDs) aim to formulate science programmes and engage the scientific community to advance the hydrological sciences. The first International Hydrological Decade was formulated in 1965 by United Nations Educational, Scientific and Cultural Organization (UNESCO) (Nace Citation1965) to highlight the field of hydrology as an independent scientific discipline, but SDs have since grown to boost thematic advances in the field of hydrology. It is now a global movement initiated and coordinated by the IAHS. The past SDs have provided the foundation for scientific collaborations and have been vital in shaping hydrological research around specific themes. The last two SDs especially have shown that well-organized community efforts can shape the field of hydrology (Hrachowitz et al. Citation2013, McMillan et al. Citation2016, Kreibich et al. Citation2017). The two most recent decades focused on prediction in ungauged basins (PUB, 2002–2012; Sivapalan et al. Citation2003) and on change in hydrology and society (Panta Rhei, 2012–2022; Montanari et al. Citation2013). The results from the PUB decade have been summarized by Hrachowitz et al. (Citation2013), and several community papers on Panta Rhei research results have already been published (e.g. McMillan et al. Citation2016, Kreibich et al. Citation2017).
Because of increased cooperation between hydrologists, the next SD is likely to have an even bigger impact than the last one. Therefore, it is important to start the discussions on a theme for the next SD. The themes of the past two decades were developed through discussions during symposia, in online blogs, and in specific sessions at IAHS conferences (Sivapalan et al. Citation2003, Montanari et al. Citation2013). The discussions were open to all hydrologists. Due to the international orientation of the IAHS, people from all over the world were involved. However, the author list of the opinion papers predominantly involved well-established researchers. While established researchers are key in shaping research, early career scientists (ECSs) are important drivers of many research projects. Although they were invited and encouraged to participate in the discussion sessions, ECSs were rarely part of the author list of the resulting opinion papers (). Since the gender balance in hydrology differs between established researchers and ECSs (Popp et al. Citation2019), the diversity of the authors was also skewed (). We perceive the lower diversity as a major disadvantage of the adopted approach, because the outcomes of the discussions may not have reflected the perspectives of the full spectrum of hydrologists.
Figure 1. Gender (top) and career-stage (bottom) diversity in co-authors of initial publications of predictions in ungauged basins (15 co-authors, Sivapalan et al. Citation2003), Panta Rhei (34 co-authors, Montanari et al. Citation2013), and this initiative (49 co-authors). For the publications of Sivapalan et al. (Citation2003) and Montanari et al. (Citation2013), the numbers are based on publicly available, online information. Early career scientists in these charts are defined as having received their latest degree (BSc, MSc, PhD) less than five years before publication of the paper. This definition was chosen to enable an unambiguous classification.
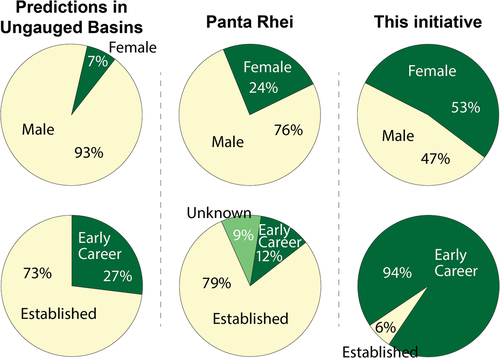
We believe that actively involving a more complete representation of hydrological researchers early on in the discussion could lead to an SD theme that is not necessarily different but at the very least supported by a larger part of the hydrological community. This broad backing of the theme will further increase the impact of the upcoming SD. To boost ECS involvement in SD discussions, we organized discussion sessions in western Europe targeting ECSs. This resulted in a gender-balanced group of co-authors consisting of mostly ECSs (). Due to the regional character of this initiative, a spatial bias is inherently present in the presented work. We therefore urge other groups of ECSs to actively share their own opinions, for example as comments on this paper or in future IAHS discussion sessions.
We present three potential themes for the upcoming SD that all offer a different perspective on the hydrological threats the world faces: “Tipping points and thresholds in hydrology,” “Intensification of the water cycle,” and “Water services under pressure.” We acknowledge that, even though the Panta Rhei decade has come to an end, change in hydrology and society is as important as it was 10 years ago (Blöschl et al. Citation2019). However, a new theme will boost hydrology and provide an opportunity to incorporate the knowledge gained in the last decade within a new focus. In addition, four key trends are presented: big data, bridging science and practice, open science, and inter- and multidisciplinarity. The trends are beyond the scope of a possible theme, as they concern the fashion in which hydrological research is or is expected to be conducted. These themes and trends can provide valuable input for future discussions on a theme for the next IAHS SD.
Methods
We aimed to involve a more diverse group of the hydrological scientific community, in particular ECSs, in the discussion on the new SD theme, for which we adopted a different approach than was applied for previous SDs. For this initiative, ECSs were not strictly defined by years since their last graduation; rather, we welcomed anyone identifying as an ECS to create an inclusive atmosphere. We organized ECS discussion sessions to identify potential themes for the upcoming SD in a joint effort led by early-career hydrologists from Wageningen University and Research (WUR). In the spring of 2022, six discussion sessions took place over the course of five weeks at WUR and five other institutes in four countries: the Karlsruhe Institute of Technology (KIT), the Luxembourg Institute of Science and Technology (LIST), the Delft University of Technology (TUD), the University of Freiburg (UoF), and the University of Zürich (UZH). Additionally, researchers from the Swiss Federal Institute of Technology in Zürich (ETH), and the Dutch branch of the Young Hydrologic Society (YHS-NL), were invited to join. Each session was attended by 10–30 participants. PhD candidates made up the majority of the participants, complemented by postdoctoral researchers and assistant professors. No master’s students joined the discussions. The participants were all either scientists or engineers focusing on sub-topics of hydrology and environmental hydraulics. In total, around 75 people attended at least one of the sessions, and 49 of those (65%) decided to stay involved in the project by co-authoring this paper.
While these sessions have greatly improved the influence of ECSs in such discussions (), the session’s geographic locations have inevitably led to a spatial bias towards high-income countries. Although the participants’ countries of origin were more diverse than the affiliated institutes (), future efforts should aim to further broaden the diversity by including a larger geographical region.
Figure 2. Regions of origin of the co-authors of this paper, according to the regions defined by the World Bank (Serajuddin et al. Citation2017).
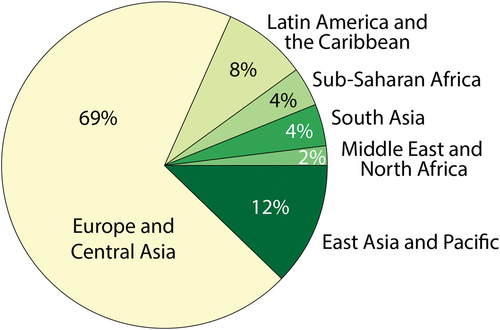
All discussion sessions lasted an hour and followed a similar format, but the content evolved during the series of discussions. Each session started with a short presentation of the history of the SDs and the aim of our initiative. Subsequently, the participants were split into groups of 4–6 people to broaden the discussion and involve all opinions. The division was targeted to create diverse groups mixing institutes and sub-disciplines of hydrology. These group conversations were guided by a set of questions that were prepared in advance. The questions developed over the sessions starting from a brainstorming level (i.e. “What do you expect to be key words for hydrology in the near future?”) towards more detailed questions in the later sessions (i.e. “What would be the research questions tackled in the proposed themes?”). All questions can be found in the Supplementary material. Finally, each group summarized their answers to the questions at the plenary discussion that followed. ECSs were encouraged to voice their opinion on the theme of the next SD in small groups of peers without their voices being unintentionally overshadowed by the presence of senior scientists.
Potential themes for the next IAHS Scientific Decade
Hydrological threats arise from pressures of the environment (e.g. climate change, ecosystem degradation, and biodiversity loss) and society (e.g. population, industrial, and economic growth). We see these threats as the central problem for hydrology in the coming decade. Hydrological threats thus should be studied, but this can be done starting from different perspectives. Three themes emerged from the discussion sessions that all postulate a perspective on how hydrology could tackle the hydrological threats faced by the environment and society. For the next IAHS Scientific Decade, we suggest that hydrological research could focus on one of the themes below:
Tipping points and thresholds in hydrology;
Intensification of the hydrological cycle;
Water services under pressure.
Tipping points and thresholds in hydrology
Tipping points are critical thresholds in complex systems such as the hydrological system. Once critical thresholds are exceeded, the system’s state heavily changes; this is referred to as a regime shift. These regime shifts can be either reversible or irreversible. A reversible tipping point indicates that the system can be restored under the same environmental circumstances, whereas an irreversible tipping point indicates that the system can only be restored after circumstances have been reversed beyond the original point, known as hysteresis (Scheffer et al. Citation2009). Both reversible and irreversible tipping points occur in hydrology. Examples of reversible tipping points are the Horton and Dunne principles of overland flow generation (Horton Citation1945, Dunne and Black Citation1970a, Citation1970b), and an example of an irreversible tipping point is a landslide due to heavy rainfall (Keefer et al. Citation1987).
As mentioned before, the hydrological cycle is affected by climate change and human interventions. Therefore, hydrology needs to advance the understanding and prediction of systems under change (Ehret et al. Citation2014), with particular attention to tipping points and their critical thresholds (Blöschl et al. Citation2019). The concept of tipping points gained momentum over the past several decades, because hydrological threats have resulted in water systems being pushed beyond their sustainable level. For instance, deforestation has led to soil erosion and karstification (Gams and Gabrovec Citation1999). Recently, warnings have repeatedly been issued that deforestation in the Amazon is likely to hit a tipping point, greatly reducing precipitation (e.g. Lovejoy and Nobre Citation2018, Amigo Citation2020). Another example is groundwater abstraction that jeopardizes groundwater-dependent vegetation (Barron et al. Citation2013).
These examples show that tipping points link the hydrological system with landscapes as well as ecosystems. In related scientific fields, tipping points are already a well-established concept. They are fundamental to the Intergovernmental Panel on Climate Change (IPCC) reports and the Planetary Boundaries framework (Rockström et al. Citation2009, Steffen et al. Citation2015, IPCC Citation2021). Based on the IPCC report, the Planetary Boundaries framework and tipping point research, warnings are frequently issued stating that passing these tipping points poses risks and will have severe impacts (Steffen et al. Citation2018, Lenton et al. Citation2019, Otto et al. Citation2020). Given the complexity and connectivity of the entire Earth system, tipping points in other scientific areas will affect hydrology and vice versa.
Next to external tipping points affecting the hydrological cycle, tipping points have also been observed in different parts of the hydrological cycle itself. Hydrological disciplines in which tipping points have been identified include surface runoff (Horton Citation1945, Dunne and Black Citation1970a, Dijkstra et al. Citation2019), groundwater (Bailey Citation2011, Figura et al. Citation2011), hydrometeorology (Buitink et al. Citation2020, Denissen et al. Citation2020, Krishnamurthy et al. Citation2020), ecohydrology (Hirota et al. Citation2011, Mayor et al. Citation2019), and water quality (Dakos et al. Citation2019, Dijkstra et al. Citation2019). Moreover, these tipping points manifest themselves in all places: from arctic (Devoie et al. Citation2019, Rosier et al. Citation2021) to temperate climates (Kupec et al. Citation2021, van der Velde et al. Citation2021), from wet (Loverde-Oliveira et al. Citation2009, Verbesselt et al. Citation2016) to arid regions (Bailey Citation2011, Bernardino et al. Citation2020), and from hydrological source (Marty Citation2008) to sink (Kirwan and Megonigal Citation2013).
While tipping points have been found, they remain difficult to identify and are often not well represented in models. Predicting and identifying hydrological tipping points is particularly challenging since the positive feedbacks that induce regime shifts originate from complex interactions and occur in heterogeneous landscapes with high connectivity (Scheffer et al. Citation2012, Nijp et al. Citation2019). In addition, modelled tipping points can only be verified after they occur (Denissen et al. Citation2020, Krishnamurthy et al. Citation2020). The impossibility of verifying unobserved tipping points is problematic since their occurrence comes with the drastic consequences of irreversible tipping behaviour for hydrological systems (e.g. Drijfhout et al. Citation2015, Dakos et al. Citation2019). Unravelling how known tipping points cause hydrological regime shifts requires the integration of different research approaches. Experiments in a controlled setting can help to identify the underlying feedback mechanisms (Webster et al. Citation2016, van de Vijsel et al. Citation2021). With conceptual models capturing the key processes, it is possible to test whether this feedback mechanism indeed causes the observed regime shift (Bailey Citation2011, Dijkstra et al. Citation2019).
At the same time, high-complexity models capturing the processes as completely as possible can be used to reproduce the conceptual simulations in settings closer to physical reality (Drijfhout et al. Citation2015). These high-complexity simulations assist with interpreting field observations and extrapolating results to future climate scenarios. In practice, integrating these scientific approaches is not straightforward. Identifying tipping points in increasingly large amounts of data is tedious, and “scanning” for tipping points with models is computationally expensive. Efficiently integrating these approaches might greatly advance our scientific understanding of hydrological regime shifts and could help us to not only identify but also successfully predict tipping points.
Given the potentially catastrophic consequences of hydrological tipping points, improving our process understanding and predictive capacity should be a focal point of future hydrological research. This is summarized in the following research questions that the theme “Tipping points and thresholds in hydrology” would address:
How can hydrological tipping points and thresholds be identified?
At what scales are the identified tipping points and thresholds relevant, and how do these scales interact?
Which non-hydrological tipping points affect hydrological systems?
What needs to be included in hydrological models to simulate and predict tipping points and thresholds? How reliable are modelled tipping points and thresholds?
How can we use our knowledge of tipping points and complex systems to mitigate the impacts of environmental and climate change?
Intensification of the water cycle
As global warming directly influences water fluxes, the hydrological cycle is strongly affected by climate change (e.g. Kundzewicz Citation2008, Peleg et al. Citation2018, Madakumbura et al. Citation2019). Climate change intensifies the hydrological cycle, increasing (for instance) the frequency and intensity of droughts and floods (Gloor et al. Citation2013, Bertola et al. Citation2020, Wasko et al. Citation2021). More hydrological extremes make securing freshwater by, for example, reservoir management increasingly difficult (Carvalho-Santos et al. Citation2017). Combined with decreasing freshwater storage due to shrinking glaciers (Beniston and Stoffel Citation2014) and the depletion of high-quality groundwater aquifers (Rotzoll and Fletcher Citation2013), the intensification of the water cycle threatens water security.
Until now, studies have mainly focused on identifying drivers of the intensification (Ziegler et al. Citation2003, Huntington Citation2006). However, less is known about mitigation of the risks that the hydrological intensification poses for agricultural productivity, water availability, and water quality (Paprotny et al. Citation2018, Abram et al. Citation2021). We urgently need to explore this impact and potential mitigation strategies. In particular, we need to identify spatial and temporal trends of dry and wet extremes in the context of a rapidly changing climate to enable adaptations that store water for drier periods and redistribute it to drier areas (e.g. Dai et al. Citation2018). We need interdisciplinary collaborations that lead to adaptations such as hydraulic structures that can prevent flash floods and a guaranteed minimum flow discharge to protect river ecosystems.
In the past, the intensification of the hydrological cycle was often described according to the “dry gets dryer, wet gets wetter” paradigm (Held and Soden Citation2006, Kitoh et al. Citation2013). However, recent studies showed that this paradigm is too simple and not universally true (Allan Citation2014, Greve et al. Citation2014, Kumar et al. Citation2015, Christidis and Stott Citation2021). Hence, we need to understand local mechanisms and drivers to help mitigate the consequences of extreme events, thereby ensuring freshwater availability. This is especially important in the Global South, where water insecurity is a substantial issue (Vörösmarty et al. Citation2010).
Increased drought occurrence and severity is a key component of the intensification of the hydrological cycle. Droughts are driven by a series of complex feedback mechanisms between (amongst other things) precipitation, soil moisture, and evaporation. Drought events manifest themselves in the environment (i.e. low discharge), but their impacts include immense social, environmental, and economic ramifications (e.g. Nilson Citation2014). Monitoring drought events is complicated as they present themselves in different parts of the water cycle (i.e. soil moisture, groundwater, surface water) in different phases of the event (van Loon Citation2015, Buitink et al. Citation2021). Remote sensing data with increasing accuracy and spatiotemporal resolution provide opportunities to monitor different parts of the hydrological cycle simultaneously (West et al. Citation2019). Regardless, challenges remain in accurately predicting droughts (Sutanto et al. Citation2020), as well as predicting the impact of climate change on drought occurrence and intensity (Vicente-Serrano et al. Citation2020). We must resolve these challenges and find solutions to prevent large-scale drought impacts.
In addition to increasing the occurrence of dry extremes, the intensified water cycle increases the occurrence of wet extremes (Addo and Adeyemi Citation2013, Pendergrass et al. Citation2017, Ansah et al. Citation2020, De Luca et al. Citation2020). In the last 10 years, numerous extreme precipitation events have occurred with extensive impacts around the globe (e.g. Duan et al. Citation2014, Otto et al. Citation2018, Abram et al. Citation2021, Wasko et al. Citation2021). A recent example is the 2021 summer flood event that impacted a large part of northwestern Europe. Here, the connection with other disciplines was clearly visible as the impacts extended beyond hydrology: increased erosion led to large scour holes in the Meuse (Task Force Fact-finding hoogwater Citation2021, Barneveld et al. Citation2022). This extreme summer flood resulted from weather circumstances with a reoccurrence time of 400 years, illustrating the extreme nature of the event (Kreienkamp et al. Citation2021). Yet this was not an isolated event: the number of extreme rainfall events is increasing due to shifting global weather patterns and rising temperatures that enhance the atmospheric moisture-holding capacity (Held and Soden Citation2006, Lenderink and van Meijgaard Citation2008, Kennedy et al. Citation2016, Lenderink et al. Citation2017). More extreme rainfall events can result in floods with high socio-economic impacts, and can increase the risk of flash floods (Alfieri et al. Citation2015, Piper et al. Citation2016, Meyer et al. Citation2021). The risk of flash floods in urban areas is even higher due to their increasingly impervious surface (Cutter et al. Citation2018).
All in all, extreme events, both dry and wet, are expected to occur more frequently in the future (Wahl et al. Citation2015, Ward et al. Citation2018, Zscheischler et al. Citation2018). The same goes for compound events, where two extremes co-occur, such as a compound drought in which a precipitation deficit coincides with a heatwave (Seneviratne et al. Citation2010, Buras et al. Citation2020), or a compound flood in which precipitation excess coincides with a storm surge (Wahl et al. Citation2015). This requires improved early warning systems to limit the negative impacts of extreme events, and long-term strategies to mitigate and cope with any remaining detrimental effects (Pappenberger et al. Citation2015, Ward et al. Citation2018, Couasnon et al. Citation2020, Abram et al. Citation2021, Wasko et al. Citation2021). However, assumptions of climate stationarity on which many of the statistical approaches are based are no longer valid (Milly et al. Citation2008). Predicting the risks of these types of events has therefore become more difficult. Improving hydrological forecasts thus requires improving the entire forecasting chain. The chain starts with weather forecasts that are the input for hydrological simulations (Emerton et al. Citation2016). These hydrological simulations provide the basis for impact forecasts (e.g. Sutanto et al. Citation2019). Finally, the risks are disseminated (Sorensen Citation2000) together with suggested mitigation strategies.
To summarize, we propose that the focus of hydrological research should shift from identifying intensification to providing knowledge on how to mitigate its effects, from local to global scales. Research questions that need answering are the following:
What is the impact of an intensified hydrological cycle on the environment, ecosystem services, and society?
What areas are most at risk from the intensification of the hydrological cycle?
How reliable are extreme event predictions that are based on extrapolating relatively short data series, and how can this reliability be improved?
How can early-warning systems be improved so that extreme events can be accurately predicted?
What mitigation strategies are suitable in the context of ongoing intensification of the hydrological cycle?
Water services under pressure
To raise awareness of the crucial role of water for nature and society, we advocate for a broader use of the “ecosystem services” framework in hydrology. More specifically, the water cycle could be seen as the ecosystem under study: “water services” (e.g. Prasad Citation2006, Lele Citation2009, Ojea et al. Citation2012). Following Daily’s (Citation1997) definition of ecosystem services, water services, or hydrological services, describe the conditions and processes through which the water cycle sustains and fulfils human life (e.g. Underwood et al. Citation2018). We propose to extend this definition to include the vital role of water in the environment. By widely acknowledging and adopting water services as a concept in hydrology, scientific advances can help secure currently vulnerable water services in a dynamic natural and social environment.
Whereas “water services” indicate the services that water provides for the environment and society, society also greatly influences the water system (Linton and Budds Citation2014, Liu et al. Citation2014). This influence was studied extensively during the Panta Rhei decade, leading to a push in the field of socio-hydrology (e.g. Scott et al. Citation2014, McMillan et al. Citation2016, Di Baldassarre et al. Citation2018, Pijl et al. Citation2018). Essential eco- and social systems heavily depend on limited water resources for services such as drinking water, irrigation water, and hydropower. This dependence explains why the substantial population and economic growth over the last century caused a sharp increase in global domestic, industrial, and agricultural water demand (Vörösmarty and Sahagian Citation2000, Oberle et al. Citation2019). The growing water demand threatens the sustainability of water systems and increases their vulnerability (Krol et al. Citation2003, McCluney et al. Citation2012). This vulnerability is exacerbated by unpredictable changes in the water cycle (e.g. hydrological intensification, salt intrusion) due to climate change (Oki and Kanae Citation2006).
While society depends on water resources, anthropogenic activities have compromised the quality of these resources and related environmental systems. For instance, sea-level rise is threatening groundwater reservoirs (Rotzoll and Fletcher Citation2013), and all parts of the water cycle are contaminated by pollutants such as plastic (Liu et al. Citation2020, van Emmerik and Schwarz Citation2020), bilge water (Tiselius and Magnusson Citation2017), nutrients (Lintern et al. Citation2020), pesticides (Payraudeau Citation2012), road salt (Szklarek et al. Citation2022), and oil (Lucas and MacGregor Citation2006). Next to affecting water quality, anthropogenic activities such as canalization also interrupt natural hydrological processes, affecting water quantity (e.g. Owens et al. Citation2005). For example, ecosystem services such as flood protection and biodiversity are more likely to be lost from river deltas as a result of human activities upstream that interrupt natural sediment transport (Hoitink et al. Citation2020). Similarly, large-scaled drainage associated with land reclamation projects reduces the buffer function of wetlands and swamps (Nobis et al. Citation2020). Therefore, there has been a call in recent research to account for the dynamic impacts of anthropogenic activities in river transformation (Russell et al. Citation2021).
In the Sustainable Development Goals, the United Nations (Citation2015) recognize that sustainable water resource management is essential to ensure a sustainable future. Still, estimates suggest that water insecurity is threatening about 80% of the world’s population (Vörösmarty et al. Citation2010). Many of these people live in ecologically fragile, conflict-ridden, and violence-affected countries that suffer the most from poorly managed water resources (Anderson et al. Citation2021, World Bank Group Citation2021). The water–peace–security nexus is further impacted by the COVID-19 pandemic (Mukhtarov et al. Citation2022) and recent intensifications of geopolitical rivalry (De Falco and Fiorentino Citation2022). We believe scientific advances in hydrology could facilitate sustainable water resource management, especially for less resilient societies that are most threatened by water insecurity.
Hydrology has supported water resource management by generating and conveying understanding of water resources and hydrological extremes (Savenije and Van der Zaag Citation2008). This traditional hydrological support should be broadened to incorporate human–water interactions, to include the spatiotemporal scales of water, and to tackle managerial challenges for transboundary water systems (Blöschl et al. Citation2019). This involves a holistic management approach, where the entire water cycle is seen as one system (Cao and Warford Citation2006, Bakker Citation2012, Giupponi and Gain Citation2017). Implementation of this holistic approach can be supported by widely adopting the use of “water services” as a concept in hydrology. We suggest four key research questions for the theme “Water services under pressure” to advance the field of hydrology:
How can we assess quantitative and qualitative water availability for sustainable water services?
What hydrological knowledge is missing to provide solutions to support water services?
How can the development of pressures on water services be identified, monitored, and predicted?
What are the scales and spatiotemporal distributions of pressures on water services?
Current trends in hydrology
Next to the themes, we identified four important trends in hydrology. These trends are not included as a theme, since they concern the way of conducting research. We note that these trends have gained traction over the past years, and think that continuing and intensifying their application in the hydrological sciences can help make research more efficient, more reproducible, and easier to apply in practice. That is why we think these trends should be incorporated in the design of the upcoming SD. The following four trends are discussed here:
Big data;
Inter-and multidisciplinarity;
Bridging science and practice;
Open science.
Big data
In the early days of hydrology, hydrological data were limited to those collected in the field. Automated sensors greatly improved the availability of in situ data, but they are still characterized by high costs and limited spatial coverage. New technologies such as remote sensing have provided us with better spatiotemporal data coverage, as well as measurements covering a larger part of the hydrological cycle, including for instance precipitation, evapotranspiration, snow, soil moisture, and water storage (Arsenault et al. Citation2016, Addor et al. Citation2017, Cui et al. Citation2018, Almagro et al. Citation2021, Klingler et al. Citation2021). Due to the size of these datasets, big data is a big topic in the environmental sciences including hydrology (Chen and Wang Citation2018, Gaffoor et al. Citation2020). We recognize the value of big data in improving data-driven science on water resources. With higher data availability, questions arise on how to use these data efficiently and how to extract knowledge from different data sources simultaneously.
Big data in hydrology presents not only new opportunities but also challenges. First of all, data quality and uncertainty are pressing issues, as poor or inconsistent data quality can lead to inaccurate interpretations and unreliable conclusions (McMillan et al. Citation2018, Lawton Citation2021). To make big data robust, they need to be validated against in situ data. Thus, in situ data collection needs to be incentivized to sustain in situ validation efforts (Allen and Berghuijs Citation2020), while research should also focus on minimizing the spatial mismatch between the scales of in situ and big data (Loew et al. Citation2017). Another challenge is that big data analyses, such as machine learning, are often complex. This complexity makes results difficult to interpret, validate, and reproduce.
Secondly, despite the development of big data, data-sparse regions still exist (Wilby Citation2019), and hydrology is often still considered a data-limited science. Data availability is not evenly distributed over the globe or over the layers of the hydrological systems. In particular, data are missing on subsurface variables. We should therefore continue to develop affordable data collection, which can help the growth of citizen-science products that have the potential to increase observations in data-sparse regions (Buytaert et al. Citation2014). We should also continue performing reanalyses to fill temporal gaps in historical data.
Lastly, storing large datasets is challenging due to limited and/or expensive storage. Historical data is already being rapidly lost (Talke and Jay Citation2013, Benito et al. Citation2015), so besides ensuring that data we collect now will remain available for future generations, we should also focus on conserving the work of previous generations that have not (yet) been digitized.
While big data has the potential to advance our understanding of hydrology, there is a strong need to develop universal data collection protocols to improve the foundations of reproducible data analysis and predictions. We should aim to use the full potential of all available data together, without subjectively selecting and rejecting data sources. We suggest increasing the cooperation between hydrologists and data scientists to jointly tackle the challenges defined here.
Inter- and multidisciplinarity
Seventeen Sustainable Development Goals were posed by the United Nations that all ascend beyond boundaries of separate scientific disciplines (United Nations Citation2015). Thus, to attain these goals, scientists need to adopt a more inter- and multidisciplinary approach. They can focus on their own discipline and share knowledge (multidisciplinarity) or combine the disciplines into a coherent whole (interdisciplinarity; Annan-Diab and Molinari Citation2017). Hydrology can be more intertwined with closely related fields of research, such as meteorology (Sene Citation2010), sedimentology (Waldschläger et al. Citation2022), and plant sciences (Konkol et al. Citation2022).
The complex themes of past and future SDs require efforts to bridge the divide between the environmental and social sciences (transdisciplinarity). In line with hydrology’s collaborative history, the non-solitary research style was also recognized as a key pillar to the success of the Panta Rhei decade (Montanari et al. Citation2013) and is gaining traction in other scientific disciplines as well (Van Noorden Citation2015). Thus, we should critically evaluate what and how scientific expertise outside of hydrology could be integrated into hydrology (Seidl and Barthel Citation2017). However, practical difficulties arise when conducting multi-, inter-, or transdisciplinary research (e.g. Lélé and Norgaard Citation2005, Strober Citation2006, Lang et al. Citation2012, Brown et al. Citation2015). Such collaborations are often characterized by considerable differences in scientific culture, potentially impeding their success. For example, environmental researchers may experience social sciences as subjective, while it may frustrate social scientists if environmental researchers do not recognize social implications (Brown et al. Citation2015). Familiarizing oneself with such cultural differences facilitates effective multi-, inter-, and transdisciplinary research.
We argue that education on these collaborative approaches as well as on related disciplines will pave the way for more successful collaborations. Funding agencies, educators, institutions, publishers, and researchers should continue to promote collaborations between disciplines to incentivize, streamline, and disseminate multi-, inter-, and transdisciplinary research to drive global sustainable development.
Bridging science and practice
One of the research questions posed in Panta Rhei was: “How can we support societies to adapt to changing conditions by considering the uncertainties and feedbacks between natural and human-induced hydrological changes?” (Montanari et al. Citation2013). This question is part of the attention that has been given to closing the gap between science and practice. We distinguish the gap between hydrology and water management and between science and the general public, and will start by discussing the first. Stakeholders are increasingly incorporated in research through collaborations between scientists, companies, and governments, often stimulated by funding agencies. For example, Cortes Arevalo et al. (Citation2020) use visual storytelling to strengthen the science–practice interface. Additionally, working groups that stimulate the bridge between science and practice have also been set up, such as the IAHS CANDHY working group. They aim to “stimulate discussion, sharing of knowledge, information, data, ideas fostering scientific and professional exchange of academic, institutional and citizen communities interested in the ‘Citizen AND HYdrology’ topic” (Montanari Citation2021, p. 1) We endorse these efforts and see them as the first part of the bridge, but we argue both gaps should be reduced even further.
In order to decrease the gap, we should overcome the difficulties that are encountered when aiming to bridge science and practice. For one, clear communication is impeded by different interpretation of water-related words such as river and dike (Venhuizen et al. Citation2019). On top of this, stakeholders may hesitate to implement scientific knowledge due to a lack of trust, contradictory findings, or high costs (Raška et al. Citation2022). Overcoming these challenges would enable the use of state-of-the-art knowledge in decision-making (McMillan et al. Citation2016) and requires clear and open communication between scientists, stakeholders, and policymakers, as well as a reflection on governance strategies based on scientific output. We acknowledge the debate on the role of science in society (Higgins et al. Citation2006), but we believe science should benefit society. Therefore, stakeholders and policymakers need to address what knowledge is needed in practice, and scientists need to clearly address the limitations of their research.
Science and the general public are brought closer by science communication. Scientists communicate their findings, because they want to be transparent to the general public (Kirchner Citation2017), to reduce scepticism (Hamilton et al. Citation2015), and to inform and educate (Dudo and Besley Citation2016). However, science communication is not easy. Scientists sharing their results have to translate their research into intriguing stories with a clear narrative about potentially controversial topics. In doing so, they may run into miscommunication, misinterpretation, and exaggeration (Lutz et al. Citation2018). We propose to empower the future generation of scientists by incorporating science communication in their curricula.
Open science
Publishing scientific work in open access (OA) format has become increasingly common, with many funding agencies requiring research to be published OA. However, open science (OS) does not end at publishing OA. OS includes opening all parts of the research process: ideation, data collection and analysis, and dissemination of the results to peers as well as the public. Science can be made more open and reproducible by sharing data on public repositories, using open software, sharing preprints and negative results, and having an open peer-review process. OS increases accessibility to fellow scientists and the public, improves reproducibility, transparency, and collaboration, and credits original ideas and work properly (Gil et al. Citation2016, van Emmerik et al. Citation2018, Hall et al. Citation2022). Moreover, OS can bridge the Global North–South research divide, leading to increased inclusivity in science practices (Adcock and Fottrell Citation2008, Tennant et al. Citation2016).
Publishers and scientists already widely acknowledge the importance of OS. Some journals require both data and code to be findable, accessible, interoperable, and reusable (FAIR standards; Wilkinson et al. Citation2016, Stall et al. Citation2017). In turn, hydrological researchers are raising awareness by sharing guidelines like the “Open Hydrology Practical Guide” (Hall et al. Citation2022).
While science as a whole is becoming increasingly open, some challenges still need to be tackled. First, OS is more expensive for the researchers, both financially and timewise. Financially, OA involves fees, and storing research data is expensive. Timewise, publishing reproducible code and data is more labour-intensive than storing code and data for personal use (Hall et al. Citation2022). Moreover, not all observations are quantifiable and transferable (Blume et al. Citation2018). Publishing code and data requires experience with (for example) version control, which is often lacking (Hall et al. Citation2022). A second challenge is that publishing data is sometimes prevented due to privacy, commercial, political, and economic concerns (Zipper et al. Citation2020). Third, preprints are often criticized for their poor scientific quality due to lacking prior peer review.
A fully open and transparent way of doing science can lead to faster advances in hydrology and is therefore, in our opinion, the only way forward. We believe that the three challenges discussed here can and should be tackled to promote OS in hydrological research. On top of that, OS should be included in education and additional efforts to practice OS should be better rewarded in the academic system. Since these efforts cannot stand on their own, it is important that funding agencies also see the value of OS. Additional funding is required to fully incorporate OS in education and to support any additional efforts scientists make to publish their research OS.
Synthesis and outlook
During the past two IAHS SDs, strong advances in the field of hydrology were made. In the first, PUB (Sivapalan et al. Citation2003), work was done on reducing predictive uncertainty in hydrology. During the second, Panta Rhei (Montanari et al. Citation2013), the interaction between hydrology and society was studied. Thanks to these decades, hydrological models and predictions have improved, as has our understanding of vital hydrological processes. The gained knowledge and improved hydrological tools allow us to tackle different problems in hydrology that we previously could not. For the upcoming SD, we therefore propose to use this enhanced toolbox to tackle hydrological threats caused by climate change and population growth. This can be approached from different perspectives. We identified three perspectives that could be selected as the theme for the upcoming IAHS SD: “Tipping points and thresholds in hydrology,” “Intensification of the water cycle,” and “Water services under pressure” (). We also identified four trends that concern the way in which hydrological research is conducted: big data, bridging science and practice, open science, and inter- and multidisciplinarity. If future research is executed according to these guidelines, it could more efficiently benefit the entire hydrological community and more effectively alleviate the hydrological threats.
The three themes and four trends are presented separately in this paper, but it should be noted that they are highly connected. The themes outline possible pathways of future hydrological research, and the trends have the potential to improve the speed, applicability, and reproducibility of hydrological research. The connectivity between themes is seen in, for instance, the co-occurrence of tipping points with the intensification of the hydrological cycle. Impact identification, mitigation strategies, and reliable implementation in hydrological models are overlapping focal points in the themes. Connectivity between trends is visible in, for instance, the fact that using big data in combination with open science could lead to quicker advances in the field, as well as a more inclusive research community. If this is further combined with effective science communication, the knowledge can be directly applied by policymakers and the public to alleviate some of the threats we are currently facing as a society.
We offered an ECS perspective in the discussion on the theme of the new IAHS SD. We synthesized the outcome of six discussion sessions in western Europe in the spring of 2022. Along with the themes, we highlighted a number of research questions that, in our view, should be addressed in the next SD. We acknowledge that the logistical limitations of our initiative have led to a spatial bias. This may have caused certain topics that are vital to the future in hydrology, especially in regions not represented by the authors, to be overlooked. To overcome the limitations posed by this bias, we encourage ECSs around the world to share their opinion, get involved in the IAHS SD discussions, and organize their own ECS discussion sessions. These sessions could be organized according to the guidelines provided in the Supplementary material, which are also available online with the possibility to post comments (https://github.com/tvhat/ECSdiscussion-IAHSSD). By targeting currently underrepresented groups with this type of sessions, inclusivity is actively pursued, which we deem necessary as a passive open invitation will not automatically lead to diversity. We hope to see a lively discussion as a result of this opinion paper and are confident that the presented themes, research questions, and trends will feed into the larger debate on the next IAHS SD.
Supplemental Material
Download MS Word (17.1 KB)Acknowledgements
Theresa C. van Hateren and Harro J. Jongen contributed equally to this work.This project was funded by the Hydrology and Quantitative Water management group, the Wageningen Institute for Environment and Climate Research, and the LEB Foundation. We thank all participants of the discussion sessions for their input. We also thank Danyka Byrnes, Moctar Dembélé, Nilay Dogulu, Pankaj Kumar, Daniel Myers, Albert Nkwasa, Bertil Nlend, Lina Stein, Anatoly Tsyplenkov, and Florentine Weber for their feedback that helped improve this manuscript and our vision on the continuation of including more diversity.
Disclosure statement
No potential conflict of interest was reported by the authors.
Supplementary material
Supplemental data for this article can be accessed online at https://doi.org/10.1080/02626667.2023.2170754
Additional information
Funding
References
- Abram, N.J., et al., 2021. Connections of climate change and variability to large and extreme forest fires in southeast Australia. Communications Earth & Environment, 2, 1–17. doi:10.1038/s43247-020-00065-8
- Adcock, J. and Fottrell, E., 2008. The North-South information highway: case studies of publication access among health researchers in resource-poor countries. Global Health Action. 1. doi:10.3402/gha.v1i0.1865
- Addo, K.A. and Adeyemi, M., 2013. Assessing the impact of sea-level rise on a vulnerable coastal community in Accra, Ghana. Jàmbá Journal Disaster Risk Stud, 5, 8. doi:10.4102/jamba.v5i1.60
- Addor, N., et al., 2017. The CAMELS data set: catchment attributes and meteorology for large-sample studies. Hydrology and Earth System Sciences, 21, 5293–5313. doi:10.5194/hess-21-5293-2017
- Alfieri, L., et al., 2015. Global warming increases the frequency of river floods in Europe. Hydrology and Earth System Sciences, 19, 2247–2260. doi:10.5194/hess-19-2247-2015
- Allan, R.P., 2014. Dichotomy of drought and deluge. Nature Geoscience, 7, 700–701. doi:10.1038/ngeo2243
- Allen, S.T. and Berghuijs, W.R., 2020. A need for incentivizing field hydrology, especially in an era of open data. Discussion of “The Role of Experimental Work in Hydrological Sciences – Insights from a Community Survey”*. Hydrological Sciences Journal, 65, 1262–1265. doi:10.1080/02626667.2018.1495837
- Almagro, A., et al., 2021. CABra: a novel large-sample dataset for Brazilian catchments. Hydrology and Earth System Sciences, 25, 3105–3135. doi:10.5194/hess-25-3105-2021
- Amigo, I., 2020. When will the Amazon hit a tipping point? Nature, 578, 505–507. doi:10.1038/d41586-020-00508-4
- Anderson, W., et al., 2021. Violent conflict exacerbated drought-related food insecurity between 2009 and 2019 in sub-Saharan Africa. Nature Food, 2, 603–615. doi:10.1038/s43016-021-00327-4
- Annan-Diab, F. and Molinari, C., 2017. Interdisciplinarity: practical approach to advancing education for sustainability and for the Sustainable Development Goals. Int. J. Manag. Educ. Principles for Responsible Management Education, 15, 73–83. doi:10.1016/j.ijme.2017.03.006
- Ansah, S.O., et al., 2020. Meteorological Analysis of Floods in Ghana. Advances in Meteorology, 2020, e4230627. doi:10.1155/2020/4230627
- Arsenault, R., et al., 2016. CANOPEX: a Canadian hydrometeorological watershed database. Hydrological Processes, 30, 2734–2736. doi:10.1002/hyp.10880
- Bailey, R.M., 2011. Spatial and temporal signatures of fragility and threshold proximity in modelled semi-arid vegetation. Proceedings of the Royal Society B: Biological Sciences, 278, 1064–1071. doi:10.1098/rspb.2010.1750
- Bakker, K., 2012. Water security: research challenges and opportunities. Science, 337, 914–915. doi:10.1126/science.1226337
- Barneveld, H., Frings, R., and Hoitink, T., 2022. Massive morphological changes during the 2021 summer flood in the River Meuse (No. EGU22-11253). Presented at the EGU22, Copernicus Meetings, Vienna, Austria. doi:10.5194/egusphere-egu22-11253
- Barron, O., et al., 2013. Projected risks to groundwater-dependent terrestrial vegetation caused by changing climate and groundwater abstraction in the Central Perth Basin, Western Australia. Resolution Outputs. doi:10.1002/hyp.10014
- Beniston, M. and Stoffel, M., 2014. Assessing the impacts of climatic change on mountain water resources. The Science of the Total Environment, 493, 1129–1137. doi:10.1016/j.scitotenv.2013.11.122
- Benito, G., et al., 2015. Quantitative historical hydrology in Europe. Hydrology and Earth System Sciences, 19, 3517–3539. doi:10.5194/hess-19-3517-2015
- Bernardino, P.N., et al., 2020. Global-scale characterization of turning points in arid and semi-arid ecosystem functioning. Global Ecology and Biogeography, 29, 1230–1245. doi:10.1111/geb.13099
- Bertola, M., et al., 2020. Flood trends in Europe: are changes in small and big floods different? Hydrol. Earth System Science Data, 24, 1805–1822. doi:10.5194/hess-24-1805-2020
- Blöschl, G., et al., 2019. Twenty-three unsolved problems in hydrology (UPH) – a community perspective. Hydrological Sciences Journal, 64, 1141–1158. doi:10.1080/02626667.2019.1620507
- Blume, T., van Meerveld, I., and Weiler, M., 2018. Incentives for field hydrology and data sharing: collaboration and compensation: reply to “A need for incentivizing field hydrology, especially in an era of open data”*. Hydrological Sciences Journal, 63, 1266–1268. doi:10.1080/02626667.2018.1495839
- Brown, R.R., Deletic, A., and Wong, T.H.F., 2015. Interdisciplinarity: how to catalyse collaboration. Nature, 525, 315–317. doi:10.1038/525315a
- Buitink, J., et al., 2020. Anatomy of the 2018 agricultural drought in The Netherlands using in situ soil moisture and satellite vegetation indices. Hydrology and Earth System Sciences, 1–17. doi:10.5194/hess-2020-358
- Buitink, J., van Hateren, T.C., and Teuling, A.J., 2021. Hydrological system complexity induces a drought frequency paradox. Frontiers in Water. 3. doi:10.3389/frwa.2021.640976
- Buras, A., Rammig, A., and Zang, C.S., 2020. Quantifying impacts of the 2018 drought on European ecosystems in comparison to 2003. Biogeosciences, 17, 1655–1672. doi:10.5194/bg-17-1655-2020
- Buytaert, W., et al., 2014. Citizen science in hydrology and water resources: opportunities for knowledge generation, ecosystem service management, and sustainable development. Frontiers in Earth Science, 2.
- Cao, Y.S. and Warford, J., 2006. Evolution of integrated approaches to water resource management in Europe and the United States: some lessons from experience.
- Carvalho-Santos, C., et al., 2017. Climate change impacts on water resources and reservoir management: uncertainty and adaptation for a mountain catchment in Northeast Portugal. Water Resource Management, 31, 3355–3370. doi:10.1007/s11269-017-1672-z
- Chen, L. and Wang, L., 2018. Recent Advance in Earth Observation Big Data for Hydrology. Big Earth Data, 2 (1), 86–107. doi:10.1080/20964471.2018.1435072.
- Christidis, N. and Stott, P.A., 2021. The influence of anthropogenic climate change on wet and dry summers in Europe. Science Bulletin, 66, 813–823. doi:10.1016/j.scib.2021.01.020
- Cortes Arevalo, V.J., et al., 2020. Storylines for practice: a visual storytelling approach to strengthen the science-practice interface. Sustainability Science, 15, 1013–1032. doi:10.1007/s11625-020-00793-y
- Couasnon, A., et al., 2020. Measuring compound flood potential from river discharge and storm surge extremes at the global scale. Natural Hazards and Earth System Sciences, 20, 489–504. doi:10.5194/nhess-20-489-2020
- Cui, Y., et al., 2018. Global water cycle and remote sensing big data: overview, challenge, and opportunities. Big Earth Data, 2, 282–297. doi:10.1080/20964471.2018.1548052
- Cutter, S.L., et al., 2018. Flash flood risk and the paradox of urban development. Natural Hazards Review. 19. doi:10.1061/(ASCE)NH.1527-6996.0000268
- Daily, G.C., 1997. Introduction: what are ecosystem services? In: G. Daily, ed. Nature’s services: societal dependence on natural ecosystems. Washington, DC: Island Press, 3.
- Dai, L., Wörner, R., and van Rijswick, H.F.M.W., 2018. Rainproof cities in the Netherlands: approaches in Dutch water governance to climate-adaptive urban planning. International Journal of Water Resources Development, 34, 652–674. doi:10.1080/07900627.2017.1372273
- Dakos, V., et al., 2019. Ecosystem tipping points in an evolving world. Nature Ecology and Evolution, 3, 355–362. doi:10.1038/s41559-019-0797-2
- De Falco, S. and Fiorentino, G., 2022. The GERD dam in the water dispute between Ethiopia, Sudan and Egypt. A Scenario Analysis in an Ecosystem Approach between Physical and Geopolitical Geography. AIMS Geosci, 8, 233–253. doi:10.3934/geosci.2022014
- De Luca, P., et al., 2020. Concurrent wet and dry hydrological extremes at the global scale. Earth System Dynamics, 11, 251–266. doi:10.5194/esd-11-251-2020
- Denissen, J.M.C., et al., 2020. Critical soil moisture derived from satellite observations over Europe. Journal of Geophysical Research: Atmospheres. 125. doi:10.1029/2019JD031672
- Devoie, É.G., et al., 2019. Taliks: a tipping point in discontinuous permafrost degradation in peatlands. Water Resources Research, 55, 9838–9857. doi:10.1029/2018WR024488
- Di Baldassarre, G., et al., 2018. Water shortages worsened by reservoir effects. Nature Sustainability, 1, 617–622. doi:10.1038/s41893-018-0159-0
- Dijkstra, Y.M., Schuttelaars, H.M., and Schramkowski, G.P., 2019. A regime shift from low to high sediment concentrations in a tide-dominated estuary. Geophysical Research Letters, 46, 4338–4345. doi:10.1029/2019GL082302
- Drijfhout, S., et al., 2015. Catalogue of abrupt shifts in intergovernmental panel on climate change climate models. Proceedings of the National Academy of Sciences, 112, E5777–E5786. doi:10.1073/pnas.1511451112
- Duan, W., et al., 2014. Anomalous atmospheric events leading to Kyushu’s flash floods, July 11 –14,2012. Natural Hazards, 73, 1255–1267. doi:10.1007/s11069-014-1134-3
- Dudo, A. and Besley, J.C., 2016. Scientists’ prioritization of communication objectives for public engagement. PLOS ONE, 11, e0148867. doi:10.1371/journal.pone.0148867
- Dunne, T. and Black, R.D., 1970a. An experimental investigation of runoff production in permeable soils. Water Resources Research, 6, 478–490. doi:10.1029/WR006i002p00478
- Dunne, T. and Black, R.D., 1970b. Partial area contributions to storm runoff in a small New England Watershed. Water Resources Research, 6, 1296–1311. doi:10.1029/WR006i005p01296
- Ehret, U., et al., 2014. Advancing catchment hydrology to deal with predictions under change. Hydrology and Earth System Sciences, 18, 649–671. doi:10.5194/hess-18-649-2014
- Emerton, R.E., et al., 2016. Continental and global scale flood forecasting systems. WIREs Water, 3, 391–418. doi:10.1002/wat2.1137
- Figura, S., et al., 2011. Regime shift in groundwater temperature triggered by the Arctic Oscillation. Geophysical Research Letters. 38. doi:10.1029/2011GL049749
- Gaffoor, Z., et al., 2020. Big Data Analytics and Its Role to Support Groundwater Management in the Southern African Development Community. Water, 12 (10), 2796. doi:10.3390/w12102796.
- Gams, I. and Gabrovec, M., 1999. Land use and human impact in the Dinaric karst. International Journal of Speleology, 28, 55–70. doi:10.5038/1827-806X.28.1.4
- Gil, Y., et al., 2016. Toward the geoscience paper of the future: best practices for documenting and sharing research from data to software to provenance. Earth Space Science, 3, 388–415. doi:10.1002/2015EA000136
- Giupponi, C. and Gain, A.K., 2017. Integrated water resources management (IWRM) for climate change adaptation. Regional Environmental Change, 17, 1865–1867. doi:10.1007/s10113-017-1173-x
- Gloor, M., et al., 2013. Intensification of the Amazon hydrological cycle over the last two decades. Geophysical Research Letters, 40, 1729–1733. doi:10.1002/grl.50377
- Greve, P., et al., 2014. Global assessment of trends in wetting and drying over land. Nature Geoscience, 7, 716–721. doi:10.1038/ngeo2247
- Hall, C.A., et al., 2022. A hydrologist’s guide to open science. Hydrology and Earth System Sciences, 26, 647–664. doi:10.5194/hess-26-647-2022
- Hamilton, L.C., Hartter, J., and Saito, K., 2015. Trust in scientists on climate change and vaccines. SAGE Open, 5, 2158244015602752. doi:10.1177/2158244015602752
- Held, I.M. and Soden, B.J., 2006. Robust responses of the hydrological cycle to global warming. Journal of Climate, 19, 5686–5699. doi:10.1175/JCLI3990.1
- Higgins, P.A.T., Chan, K.M.A., and Porder, S., 2006. Bridge over a philosophical divide. Evidence & Policy, 2, 249–255. doi:10.1332/174426406777068920
- Hirota, M., et al., 2011. Global resilience of tropical forest and savanna to critical transitions. Science, 334, 232–235. doi:10.1126/science.1210657
- Hoitink, A.J.F., et al., 2020. Resilience of river deltas in the anthropocene. Journal of Geophysical Research: Earth Surface, 125, e2019JF005201. doi:10.1029/2019JF005201
- Horton, R.E., 1945. Erosional development of streams and their drainage basins; hydrophysical approach to quantitative morphology. Geological Society of America Bulletin, 56, 275. doi:10.1130/0016-7606(1945)56[275:
- Hrachowitz, M., et al., 2013. A decade of Predictions in Ungauged Basins (PUB)—a review. Hydrological Sciences Journal, 58, 1198–1255. doi:10.1080/02626667.2013.803183
- Huntington, T.G., 2006. Evidence for intensification of the global water cycle: review and synthesis. Journal of Hydrology, 319, 83–95. doi:10.1016/j.jhydrol.2005.07.003
- IPCC, 2021. Climate change 2021: the physical science basis. Contribution of working group i to the sixth assessment report of the intergovernmental panel on climate change. Cambridge University Press.
- Keefer, D.K., et al., 1987. Real-time landslide warning during heavy rainfall. Science, 238, 921–925. doi:10.1126/science.238.4829.921
- Kennedy, D., et al., 2016. The response of high-impact blocking weather systems to climate change. Geophysical Research Letters, 43, 7250–7258. doi:10.1002/2016GL069725
- Kirchner, J.W., 2017. Science, politics, and rationality in a partisan era. Water Resources Research, 53, 3545–3549. doi:10.1002/2017WR020882
- Kirwan, M.L. and Megonigal, J.P., 2013. Tidal wetland stability in the face of human impacts and sea-level rise. Nature, 504, 53–60. doi:10.1038/nature12856
- Kitoh, A., et al., 2013. Monsoons in a changing world: a regional perspective in a global context. Journal of Geophysical Research: Atmospheres, 118, 3053–3065. doi:10.1002/jgrd.50258
- Klingler, C., Schulz, K., and Herrnegger, M., 2021. LamaH-CE: lArge-SaMple data for hydrology and environmental sciences for Central Europe. Earth System Science Data, 13, 4529–4565. doi:10.5194/essd-13-4529-2021
- Konkol, A., et al., 2022. Interplay of river and tidal forcings promotes loops in coastal channel networks. Geophysical Research Letters, 49 (e2022GL098284). doi:10.1029/2022GL098284
- Kreibich, H., et al., 2017. Scientific debate of Panta Rhei research – how to advance our knowledge of changes in hydrology and society? Hydrol. Science Journal, 62, 331–333. doi:10.1080/02626667.2016.1209929
- Kreienkamp, F., et al., 2021. Rapid attribution of heavy rainfall events leading to the severe flooding in Western Europe during July 2021. World Weather Attribution. https://www.worldweatherattribution.org/wp-content/uploads/Scientific-report-Western-Europe-floods-2021-attribution.pdf
- Krishnamurthy, R.P.K., et al., 2020. Applying tipping point theory to remote sensing science to improve early warning drought signals for food security. Earths Future, 8, e2019EF001456. doi:10.1029/2019EF001456
- Krol, M.S., Jaeger, A.-K., and Bronstert, A., 2003. Integrated modeling of climate change impacts in Northeastern Brazil. In: T. Gaiser, et al., eds. Global change and regional impacts: water availability and vulnerability of ecosystems and society in the Semiarid Northeast of Brazil. Berlin, Heidelberg: Springer, 43–56. doi:10.1007/978-3-642-55659-3_3
- Kumar, S., et al., 2015. Revisiting trends in wetness and dryness in the presence of internal climate variability and water limitations over land. Geophysical Research Letters, 42 (10), 867–10, 875. doi:10.1002/2015GL066858
- Kundzewicz, Z., 2008. Climate change impacts on the hydrological cycle. Ecohydrology & Hydrobiology, 8, 195–203. doi:10.2478/v10104-009-0015-y
- Kupec, P., Deutscher, J., and Futter, M., 2021. Longer growing seasons cause hydrological regime shifts in Central European Forests. Forests, 12, 1656. doi:10.3390/f12121656
- Lang, D.J., et al., 2012. Transdisciplinary research in sustainability science: practice, principles, and challenges. Sustainability Science. 7. doi:10.1007/s11625-011-0149-x
- Lawton, G., 2021. Data quality for big data: why it’s a must and how to improve it. https://www.techtarget.com/searchdatamanagement/feature/Data-quality-for-big-data-Why-its-a-must-and-how-to-improve-it ( accessed 5 Nov 2022).
- Lele, S., 2009. Watershed services of tropical forests: from hydrology to economic valuation to integrated analysis. Current Opinion in Environmental Sustainability, 1, 148–155. doi:10.1016/j.cosust.2009.10.007
- Lélé, S. and Norgaard, R.B., 2005. Practicing Interdisciplinarity. BioScience, 55, 967–975. doi:10.1641/0006-3568(2005)055[0967:
- Lenderink, G., et al., 2017. Super-clausius–clapeyron scaling of extreme hourly convective precipitation and its relation to large-scale atmospheric conditions. Journal of Climate, 30, 6037–6052. doi:10.1175/JCLI-D-16-0808.1
- Lenderink, G. and van Meijgaard, E., 2008. Increase in hourly precipitation extremes beyond expectations from temperature changes. Nature Geoscience, 1, 511–514. doi:10.1038/ngeo262
- Lenton, T.M., et al., 2019. Climate tipping points — too risky to bet against. Nature, 575, 592–595. doi:10.1038/d41586-019-03595-0
- Lintern, A., et al., 2020. Best management practices for diffuse nutrient pollution: wicked problems across urban and agricultural watersheds. Environmental Science & Technology, 54, 9159–9174. doi:10.1021/acs.est.9b07511
- Linton, J. and Budds, J., 2014. The hydrosocial cycle: defining and mobilizing a relational-dialectical approach to water. Geoforum, 57, 170–180. doi:10.1016/j.geoforum.2013.10.008
- Liu, Y., et al., 2014. Socio-hydrologic perspectives of the co-evolution of humans and water in the Tarim River basin, Western China: the Taiji–Tire model. Hydrology and Earth System Sciences, 18, 1289–1303. doi:10.5194/hess-18-1289-2014
- Liu, K., et al., 2020. Elucidating the vertical transport of microplastics in the water column: a review of sampling methodologies and distributions. Water Research, 186, 116403. doi:10.1016/j.watres.2020.116403
- Loew, A., et al., 2017. Validation practices for satellite-based Earth observation data across communities. Reviews of Geophysics, 55, 779–817. doi:10.1002/2017RG000562
- Lovejoy, T.E. and Nobre, C., 2018. Amazon Tipping Point. Science Advances, 4 (eaat2340). doi:10.1126/sciadv.aat2340
- Loverde-Oliveira, S.M., et al., 2009. Hydrology-driven regime shifts in a shallow tropical lake. Ecosystems, 12, 807.
- Lucas, Z. and MacGregor, C., 2006. Characterization and source of oil contamination on the beaches and seabird corpses, Sable Island, Nova Scotia, 1996–2005. Marine Pollution Bulletin, 52, 778–789. doi:10.1016/j.marpolbul.2005.11.023
- Lutz, S.R., et al., 2018. HESS opinions: science in today’s media landscape – challenges and lessons from hydrologists and journalists. Hydrology and Earth System Sciences, 22, 3589–3599. doi:10.5194/hess-22-3589-2018
- Madakumbura, G.D., et al., 2019. Event-to-event intensification of the hydrologic cycle from 1.5 °C to a 2 °C warmer world. Scientific Reports, 9, 3483. doi:10.1038/s41598-019-39936-2
- Marty, C., 2008. Regime shift of snow days in Switzerland. Geophysical Research Letters. 35. doi:10.1029/2008GL033998
- Mayor, A.G., et al., 2019. Connectivity-mediated ecohydrological feedbacks and regime shifts in Drylands. Ecosystems, 22, 1497–1511. doi:10.1007/s10021-019-00366-w
- McCluney, K.E., et al., 2012. Shifting species interactions in terrestrial dryland ecosystems under altered water availability and climate change. Biological Reviews, 87, 563–582. doi:10.1111/j.1469-185X.2011.00209.x
- McMillan, H., et al., 2016. Panta Rhei 2013–2015: global perspectives on hydrology, society and change. Hydrological Sciences Journal, 61, 1174–1191. doi:10.1080/02626667.2016.1159308
- McMillan, H.K., Westerberg, I.K., and Krueger, T., 2018. Hydrological data uncertainty and its implications. WIREs Water, 5, e1319. doi:10.1002/wat2.1319
- Meyer, J., et al., 2021. More frequent flash flood events and extreme precipitation favouring atmospheric conditions in temperate regions of Europe. Hydrology and Earth System Sciences, 1–28. doi:10.5194/hess-2021-628
- Milly, P.C.D., et al., 2008. Stationarity is dead. Whither Water Management? Science, 319, 573–574. doi:10.1126/science.1151915
- Montanari, A., et al., 2013. “Panta rhei—everything flows”: change in hydrology and society—the IAHS scientific decade 2013–2022. Hydrological Sciences Journal, 58, 1256–1275. doi:10.1080/02626667.2013.809088
- Montanari, A., 2021. ‘International Association of Hydrological Sciences -IAHS Activities’ Report – Year 2020. Bologna. https://www.cnr.it/it/organismi-scientifici-internazionali/relazione/404/relazione-2020-eng-iahs.pdf.
- Mukhtarov, F., Papyrakis, E., Rieger, M., 2022. Covid-19 and Water. In: E. Papyrakis, ed. COVID-19 and international development. Berlin, Germany: Springer International Publishing, Cham, 157–173. doi:10.1007/978-3-030-82339-9_12
- Nace, R.L., 1965. Status of the international hydrological decade. Journal AWWA, 57, 819–823. doi:10.1002/j.1551-8833.1965.tb01468.x
- Nijp, J.J., et al., 2019. Spatial early warning signals for impending regime shifts: a practical framework for application in real-world landscapes. Global Change Biology, 25, 1905–1921. doi:10.1111/gcb.14591
- Nilson, E., 2014. Auswirkungen des Klimawandels auf das Abflussgeschehen und die Binnenschifffahrt in Deutschland: schlussbericht KLIWAS-Projekt 4.01, KLIWAS-Schriftenreihe/BfG – KLIWAS-43/2014. Bundesanstalt für Gewässerkunde, Koblenz. 10.5675/KLIWAS_43/2014_4.01
- Nobis, K., et al., 2020. Die Anwendung der ländlichen Bodenordnung bei der Renaturierung und naturnahen Entwicklung von Fließgewässern, 1. Aufl. 2020. Wiesbaden Heidelberg: Springer Spektrum.
- Oberle, B., et al., 2019. Global resources outlook: 2019. Paris, France: International Resource Panel, United Nations Envio.
- Ojea, E., Martin-Ortega, J., and Chiabai, A., 2012. Defining and classifying ecosystem services for economic valuation: the case of forest water services. Environmental Science & Policy, 19–20, 1–15. doi:10.1016/j.envsci.2012.02.002
- Oki, T. and Kanae, S., 2006. Global hydrological cycles and world water resources. Science, 313, 1068–1072. doi:10.1126/science.1128845
- Otto, F.E.L., et al., 2018. Anthropogenic influence on the drivers of the Western Cape drought 2015–2017. Environmental Research Letters, 13, 124010. doi:10.1088/1748-9326/aae9f9
- Otto, I.M., et al., 2020. Social tipping dynamics for stabilizing Earth’s climate by 2050. Proceedings of the National Academy of Sciences, 117, 2354–2365. doi:10.1073/pnas.1900577117
- Owens, P.N., et al., 2005. Fine-grained sediment in river systems: environmental significance and management issues. River Research and Applications, 21, 693–717. doi:10.1002/rra.878
- Pappenberger, F., et al., 2015. The monetary benefit of early flood warnings in Europe. Environmental Science & Policy, 51, 278–291. doi:10.1016/j.envsci.2015.04.016
- Paprotny, D., et al., 2018. Trends in flood losses in Europe over the past 150 years. Nature Communications, 9, 1985. doi:10.1038/s41467-018-04253-1
- Payraudeau, G., 2012. Modelling pesticides transfer to surface water at the catchment scale: a multi-criteria analysis. Agronomy for Sustainable Development, 32, 479–500. doi:10.1007/s13593-011-0023-3
- Peleg, N., et al., 2018. Intensification of convective rain cells at warmer temperatures observed from high-resolution weather radar data. Journal Hydrometeorol, 19, 715–726. doi:10.1175/JHM-D-17-0158.1
- Pendergrass, A.G., et al., 2017. Precipitation variability increases in a warmer climate. Scientific Reports, 7, 17966. doi:10.1038/s41598-017-17966-y
- Pijl, A., et al., 2018. Hydrologic impacts of changing land use and climate in the Veneto lowlands of Italy. Anthropocene, 22, 20–30. doi:10.1016/j.ancene.2018.04.001
- Piper, D., et al., 2016. Exceptional sequence of severe thunderstorms and related flash floods in May and June 2016 in Germany – part 1: meteorological background. Natural Hazards and Earth System Sciences, 16, 2835–2850. doi:10.5194/nhess-16-2835-2016
- Popp, A.L., et al., 2019. A global survey on the perceptions and impacts of gender inequality in the Earth and Space Sciences. Earth Space Science, 6, 1460–1468. doi:10.1029/2019EA000706
- Prasad, N., 2006. Privatisation results: private sector participation in water services after 15 years. Development Policy Review, 24, 669–692. doi:10.1111/j.1467-7679.2006.00353.x
- Raška, P., et al., 2022. Identifying barriers for nature-based solutions in flood risk management: an interdisciplinary overview using expert community approach. Journal of Environmental Management, 310, 114725. doi:10.1016/j.jenvman.2022.114725
- Rockström, J., et al., 2009. Planetary boundaries: exploring the safe operating space for humanity. Ecology and Society, 14 (art32). doi:10.5751/ES-03180-140232
- Rosier, S.H.R., et al., 2021. The tipping points and early warning indicators for Pine Island Glacier, West Antarctica. The Cryosphere, 15, 1501–1516. doi:10.5194/tc-15-1501-2021
- Rotzoll, K. and Fletcher, C.H., 2013. Assessment of groundwater inundation as a consequence of sea-level rise. Nature Climate Change, 3, 477–481. doi:10.1038/nclimate1725
- Russell, C., et al., 2021. Geological evolution of the mississippi river into the anthropocene. Anthropology Review, 8, 115–140. doi:10.1177/20530196211045527
- Savenije, H.H.G. and Van der Zaag, P., 2008. Integrated water resources management: concepts and issues. Physics Chemistry of the Earth Parts ABC, Integrated Water Resources Management in a Changing World, 33, 290–297. doi:10.1016/j.pce.2008.02.003
- Scheffer, M., et al., 2009. Early-warning signals for critical transitions. Nature, 461, 53–59. doi:10.1038/nature08227
- Scheffer, M., et al., 2012. Anticipating critical transitions. Science, 338, 344–348. doi:10.1126/science.1225244
- Scott, C.A., et al., 2014. Irrigation efficiency and water-policy implications for river basin resilience. Hydrology and Earth System Sciences, 18, 1339–1348. doi:10.5194/hess-18-1339-2014
- Seidl, R. and Barthel, R., 2017. Linking scientific disciplines: hydrology and social sciences. Journal of Hydrology, 550, 441–452. doi:10.1016/j.jhydrol.2017.05.008
- Sene, K., 2010. Hydrometeorology.
- Seneviratne, S.I., et al., 2010. Investigating soil moisture–climate interactions in a changing climate: a review. Earth-Science Review, 99, 125–161. doi:10.1016/j.earscirev.2010.02.004
- Serajuddin, U., et al., 2017. Atlas of sustainable development goals 2017: world development indicators. Disclosure, vi–ix.
- Sivapalan, M., et al., 2003. IAHS decade on Predictions in Ungauged Basins (PUB), 2003–2012: shaping an exciting future for the hydrological sciences. Hydrological Sciences Journal, 48, 857–880. doi:10.1623/hysj.48.6.857.51421
- Sorensen, J.H., 2000. Hazard warning systems: review of 20 years of progress. Natural Hazards Review, 1, 119–125. doi:10.1061/(ASCE)1527-6988(2000)1:2(119)
- Stall, S., et al., 2017. Enabling FAIR data across the Earth and Space Sciences. Eos. doi:10.1029/2017EO088425
- Steffen, W., et al., 2015. Planetary boundaries: guiding human development on a changing planet. Science, 347, 1259855. doi:10.1126/science.1259855
- Steffen, W., et al., 2018. Trajectories of the Earth System in the anthropocene. Proceedings of the National Academy of Sciences, 115, 8252–8259. doi:10.1073/pnas.1810141115
- Strober, M.H., 2006. Habits of the mind: challenges for multidisciplinary engagement. Social Epistemology, 20, 315–331. doi:10.1080/02691720600847324
- Sutanto, S.J., et al., 2019. Moving from drought hazard to impact forecasts. Nature Communications, 10, 4945. doi:10.1038/s41467-019-12840-z
- Sutanto, S.J., Wetterhall, F., and Lanen, H.A.J.V., 2020. Hydrological drought forecasts outperform meteorological drought forecasts. Environmental Research Letters, 15, 084010. doi:10.1088/1748-9326/ab8b13
- Szklarek, S., Górecka, A., and Wojtal-Frankiewicz, A., 2022. The effects of road salt on freshwater ecosystems and solutions for mitigating chloride pollution - A review. The Science of the Total Environment, 805, 150289. doi:10.1016/j.scitotenv.2021.150289
- Talke, S.A. and Jay, D.A., 2013. Nineteenth Century North American and Pacific tidal data: lost or just forgotten? Journal Coast Restaurant, 29, 118–127. doi:10.2112/JCOASTRES-D-12-00181.1
- Task Force Fact-finding hoogwater, 2021. Hoogwater 2021; Feiten en Duiding. Expertisenetwerk waterveiligheid.
- Tennant, J.P., et al., 2016. The academic, economic and societal impacts of open access: an evidence-based review. F1000Research, 5, 632. doi:10.12688/f1000research.8460.3
- Tiselius, P. and Magnusson, K., 2017. Toxicity of treated bilge water: the need for revised regulatory control. Marine Pollution Bulletin, 114, 860–866. doi:10.1016/j.marpolbul.2016.11.010
- Underwood, E.C., et al., 2018. Climate change impacts on hydrological services in Southern California. Environmental Research Letters 13, No, 12, 124019. doi:10.1088/1748-9326/aaeb59
- United Nations, 2015. Transforming our world: the 2030 agenda for sustainable development.
- van der Velde, Y., et al., 2021. Emerging forest-peatland bistability and resilience of European peatland carbon stores. Proceedings of the National Academy of Sciences of the United States of America, 118, e2101742118. doi:10.1073/pnas.2101742118
- van de Vijsel, R.C., et al., 2021. Algal-induced biogeomorphic feedbacks lay the groundwork for coastal wetland development. Journal of Geophysical Research Biogeosciences, 126 (e2021JG006515). doi:10.1029/2021JG006515
- van Emmerik, T., et al., 2018. Reporting negative results to stimulate experimental hydrology. Discussion of “The Role of Experimental Work in Hydrological Sciences – Insights from a Community Survey.” Hydrological Sciences Journal, 63, 1269–1272. doi:10.1080/02626667.2018.1493203
- van Emmerik, T. and Schwarz, A., 2020. Plastic debris in rivers. WIREs Water, 7, e1398. doi:10.1002/wat2.1398
- van Loon, A.F., 2015. Hydrological drought explained. WIREs Water, 2, 359–392. doi:10.1002/wat2.1085
- Van Noorden, R., 2015. Interdisciplinary research by the numbers. Nature News, 525, 306. doi:10.1038/525306a
- Venhuizen, G.J., et al., 2019. Flooded by jargon: how the interpretation of water-related terms differs between hydrology experts and the general audience. Hydrology and Earth System Sciences, 23, 393–403. doi:10.5194/hess-23-393-2019
- Verbesselt, J., et al., 2016. Remotely sensed resilience of tropical forests. Nature Climate Change, 6, 1028–1031.
- Vicente-Serrano, S.M., et al., 2020. Global characterization of hydrological and meteorological droughts under future climate change: the importance of timescales, vegetation-CO2 feedbacks and changes to distribution functions. International Journal of Climatology, 40, 2557–2567. doi:10.1002/joc.6350
- Vörösmarty, C.J., et al., 2010. Global threats to human water security and river biodiversity. Nature, 467, 555–561. doi:10.1038/nature09440
- Vörösmarty, C.J. and Sahagian, D., 2000. Anthropogenic disturbance of the terrestrial water cycle. BioScience, 50, 753–765. doi:10.1641/0006-3568(2000)050[0753:
- Wahl, T., et al., 2015. Increasing risk of compound flooding from storm surge and rainfall for major US cities. Nature Climate Change, 5, 1093–1097. doi:10.1038/nclimate2736
- Waldschläger, K., et al., 2022. Learning from natural sediments to tackle microplastics challenges: a multidisciplinary perspective. Earth-Science Review, 228, 104021. doi:10.1016/j.earscirev.2022.104021
- Ward, P.J., et al., 2018. Dependence between high sea-level and high river discharge increases flood hazard in global deltas and estuaries. Environmental Research Letters, 13, 084012. doi:10.1088/1748-9326/aad400
- Wasko, C., et al., 2021. Understanding trends in hydrologic extremes across Australia. Journal of Hydrology, 593, 125877. doi:10.1016/j.jhydrol.2020.125877
- Webster, J.R., et al., 2016. Evidence for a regime shift in nitrogen export from a forested watershed. Ecosystems, 19, 881–895. doi:10.1007/s10021-016-9974-1
- West, H., Quinn, N., and Horswell, M., 2019. Remote sensing for drought monitoring & impact assessment: progress, past challenges and future opportunities. Remote Sensing of Environment, 232, 111291. doi:10.1016/j.rse.2019.111291
- Wilby, R.L., 2019. A global hydrology research agenda fit for the 2030s. Hydrology Research, 50, 1464–1480. doi:10.2166/nh.2019.100
- Wilkinson, M.D., et al., 2016. The FAIR guiding principles for scientific data management and stewardship. Science Data, 3, 160018. doi:10.1038/sdata.2016.18
- World Bank Group, 2021. Global Water Security and Sanitation Partnership Annual Report 2021.
- Ziegler, A.D., et al., 2003. Detection of intensification in global- and continental-scale hydrological cycles: temporal scale of evaluation. Journal of Climate, 16, 535–547. doi:10.1175/1520-0442(2003)016<0535:
- Zipper, S.C., et al., 2020. Integrating the water planetary boundary with water management from local to global scales. Earths Future, 8 (e2019EF001377). doi:10.1029/2019EF001377
- Zscheischler, J., et al., 2018. Future climate risk from compound events. Nature Climate Change, 8, 469–477. doi:10.1038/s41558-018-0156-3