ABSTRACT
We studied novice gymnasts (N = 25) learning to form the longswing movement coordination pattern. The focus was the emerging behavioural organisation of centre of mass (CM) dynamics and the relative phase of the bar–CM angular motion. Seven novices learned a complete longswing by the end of the study, 8 novices showed no improvement in proportion of circle completed, and the remainder produced modest but persistent increments of final swing height without achieving a full circle. The radial angular velocity generated in the free fall phase and the circle location of bar-CM relative phase progressively and predominantly predicted circle completion. Bar-CM relative phase produced a consistent qualitative relation within- and between-subjects characteristic of a collective variable with the bar leading the CM on the initial downward antiphase segment and the CM leading on the upward antiphase segment. The ratio of these phases was related by the last practice session to degree of circle completion. The findings showed strong individual differences in the effect of practice on the early steps of learning the movement coordination where the progressive emergence of the longswing circle is driven by exploiting the positive- and minimising the negative-influence of gravity on the bar–CM coordination dynamics (candidate collective variable).
Introduction
It is a long-held perspective across a number of theoretical and practical viewpoints that learning a perceptual-motor skill through practice necessarily requires the participant to progress through a sequence of steps or stages in the search for a predictable and efficient task-relevant outcome (Fitts, Citation1964; Gentile, Citation1972). This progressive qualitative and quantitative change in behaviour is taken to map to an enhanced performance outcome over practice. It is from the relatively persistent change in behaviour over practice time that inferences about motor skill learning are advanced. In general, the frameworks for the qualitative organisation structure of the perceptual-motor output are associated with quantitative advances in movement outcome as reflected in the prevalent exponential and power-law accounts of learning/performance curves (Newell et al., Citation2006).
The role of a sequential order in the progression of perceptual-motor skill learning has a place in the coordinative structure theory of motor learning and control (Kugler et al., Citation1980; Newell, Citation1985) and the complementary framework of coordination dynamics (Kelso, Citation1995). In this approach, an essential feature of the initial stage of learning is forming a task-relevant coordination pattern as defined by its topological dynamics – the qualitative space-time properties of the movement pattern (Beek & Van Santvoord, Citation1992; Bernstein, Citation1967; Mitra et al., Citation1998; Newell, Citation1985; Turvey, Citation1990). This qualitative structure is reflected in the macroscopic framework of an order parameter(s) or collective variable(s) (Haken et al., Citation1985; Kelso, Citation2021; Mitra et al., Citation1998; Newell & Liu, Citation2020). The subsequent embedded stages of learning in a dynamical framework are the continued stabilisation of the collective variable and enhancement of the efficiency (work/energy expenditure ratio) of the movement organisation. Thus, one can view perceptual-motor skill learning across the improvement of performance outcome as the embedded processes of coordination, control and skill (Newell, Citation1985).
In the systems-oriented approach of coordination dynamics a central issue is the identification of the relevant variables for movement coordination and control (Kelso, Citation1995; Kugler et al., Citation1980). This approach to task analysis reveals that empirical examination of the initial stage of perceptual-motor skill learning has largely been bypassed by the choice of experimental task for study (Newell, Citation1985). The typical laboratory and everyday skills studied have used tasks that the participant can already produce the relevant qualitative properties of the coordination pattern. In effect, the tasks have primarily required the participant to rescale in force, time or space a relatively stable established coordination mode. This has led the study of motor skill acquisition to have been restricted to the processes of nonoptimal control in what is more realistically the intermediate stage of learning (Mitra et al., Citation1998; Newell, Citation1985).
There are relatively few experimental examinations of adults learning a new movement coordination pattern (for reviews see Button et al., Citation2021; Newell & Liu, Citation2020; Newell & McDonald, Citation1994; Wu & Latash, Citation2014). There are even fewer experimental papers in the narrower context of learning a gymnastics skill (Williams et al., Citation2012, Citation2015a, b, Lee et al., Citation2016; Busquets et al., Citation2011, Citation2013a, b) and in particular determining if an associated collective variable captures the change in the macroscopic qualitative organisation of the movement dynamics (Haken et al., Citation1985; Kelso, Citation1995). Zanone and Kelso (Citation1992) showed that subjects through practice can learn to form a new stable relative phase in the bimanual two degree of freedom (DF) coordination task at a previously unstable relative phase value of 90 degrees. Transitions in learning the coordination dynamics of a motor task have also been reported for a number of tasks including: creeping on a treadmill (Sparrow & Irizarry-Lopez, Citation1987); rollerball (Liu et al., Citation2019); and kicking a soccer ball (Anderson & Sidaway, Citation1994).
The sport of gymnastics provides a structured and typically novel set of movement coordinative structures from those of everyday activities for a prospective gymnast to learn novel patterns of coordination (Fukushima & Russell, Citation1980; Readhead, Citation1997). Perhaps the most studied gymnastic skill is that of the longswing on the high bar that has been investigated from kinematic (Bauer, Citation1983; Irwin & Kerwin, Citation2005; Williams et al., Citation2012), kinetic (Irwin & Kerwin, Citation2007a; Okamoto et al., Citation1987; Williams et al., Citation2015a) and energetic (Arampatzis & Brüggemann, Citation2001; Williams et al., Citation2015b) frames of reference. The forces on the bar have also been determined for the longswing (Kerwin & Hiley, Citation2003; Okamoto, Sakurai, Ikegami, & Yabe, Citation1987; Sheets & Hubbard, Citation2009) but not their coupling to the motion of the body CM. In addition, Arampatzis and Brüggemann (Citation1998), Yeadon and Hiley (Citation2000), and Hiley and Yeadon (Citation2003) have provided biomechanical models of the longswing in elite gymnasts. More recently, Vicinanza et al. (Citation2018) characterised the high bar circle motion of CM angular velocity in terms of limit cycle dynamics that were modulated by skill level of the gymnast.
The high bar longswing fits the task category of an inherently unstable system that requires active stabilisation of the system dynamics by the participant (Newell et al., Citation2001; Warren, Citation2006), if a complete circle is to be produced. Biomechanical analyses have shown that the progressive sequential segments of the circle are constrained by a different confluence of internal and external generated forces on the motion of the CM (Irwin et al., Citation2019). The initial free fall phase from the inverted vertical is dominated by the largely positive influence of gravity whereas the upward second half segment motion is against gravity and depends on the angular momentum of the gymnast at around the lowest point of the circle, in addition to, the insert of energy from the gymnast on the bar in the upward swing (Irwin et al., Citation2019).
The task requires the performer to insert energy into the circle dynamics so as to maintain sufficient momentum in the last quarter segment of upward circular rotation of the body around the bar and return to the apex of the circle in preparation for the next longswing circle or related skill. Therefore, the performer interaction with the bar is likely key to understanding the collective dynamics of the longswing. Without the active insert by the gymnast of energy to the system dynamics the body motion would dampen to that of a stationary hanging pendulum. shows a schematic of the preliminary description of the sequential phases of the longswing around the circle (Irwin et al., Citation2019). Previous studies have shown that novice gymnasts tend to insert energy into the system by hip rather than shoulder motion as in elite gymnasts (Williams et al., Citation2015b). Here we examine the role of hip and shoulder kinematics in the upswing by the novice and the hypothesis that greater long swing circle completion is related to later energy insertion in the functional phase.
Figure 1. Diagrammatic representation of the longswing with the gymnast rotating anti clockwise about the bar. The angular velocity about the circle angle Vicinanza et al. (Citation2018) and angular position of the gymnasts during the functional phases of the shoulder and hip joints from Irwin and Kerwin (Citation2005) are highlighted
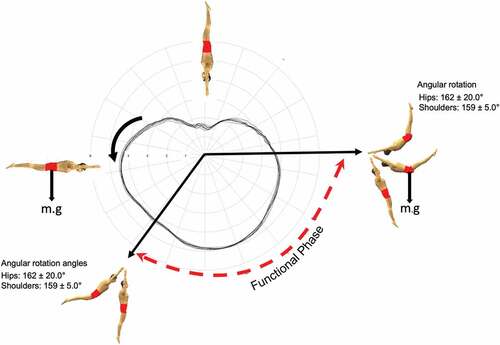
shows the long swing movement sequence and features the “functional phase”, a reference to the hip and shoulder joint actions during the lower vertical of the swing. The “functional” phase of the skill was considered to occur around maximum hip extension to flexion and maximum shoulder flexion to extension (Irwin & Kerwin, Citation2005). This phase, described by the joint angular kinematics has been highlighted to be a key phase in terms of generating the angular momentum required to perform the longswing successfully (Irwin & Kerwin, Citation2005, Citation2005, p. 2007ab).
Our focus here is the initial practice in the early stage of learning and, in particular, the acquisition of the qualitative dynamics of the coordination pattern of the longswing – that is, novice gymnasts learning to form for the first time the movement pattern of a complete longswing circle. We examined individual trials as a function of practice on the emergence of: a) the complete circle of the longswing dynamics; b) the relative phase of the CM in terms of its coupling to the motion of the high bar; and in the part 2 accompanying paper: c) the relation of energy input variables to success in forming the complete longswing cycle dynamics. The coupling of the bar and body dynamics was analysed as a function of different segments of the circle motion and viewed as a candidate collective variable for the high bar longswing task.
The primary hypotheses examined were: 1) There will be between-subject variation in the rate with which individuals learn through practice to produce their first complete longswing circle. Indeed, it is possible that not all subjects will complete a full circle over the month time course of training and testing; 2) the two-dimensional circle motion of the CM in a single longswing trial will become with practice progressively more complete and symmetrical with greater amplitude. Plotting the CM motion in a position-velocity frame of reference, however, will reveal asymmetries in the CM dynamics as a function of location in the circle; 3) the coupling between motion of CM and the bar vertical motion will become stronger with practice and the learning of a progressively more complete longswing circle; and 4) practice will lead to the later initiation of hip and/or shoulder joint motion for energy insertion in the functional phase of the longswing.
Methods
Participants
There were 25 male participants, with a mean ±SD age: 19.8 ± 1.0 yrs, mass: 75.8 ± 7.5 kg, and stature: 1.78 ± 0.7 m who volunteered to take part in this study. The Cardiff Metropolitan University Ethics Committee approved the project and the participant voluntarily signed a consent form prior to participation in the study. Prior to entry to the study each participant showed that they could undertake the recommendations of Readhead (Citation1997), this provided the recommended physical requirements to learn this skill, in addition a National level coach was present throughout to ensure safety.
Data collection
The data for this study came from the doctoral thesis of Irwin and Kerwin (Citation2005) on the biomechanics of the high bar longswing. The focus of the analysis here is distinct from the publications to date from the dissertation project (Irwin et al., Citation2004; Irwin & Kerwin, Citation2005, Citation2005, Citation2007a, Citation2007b). The relevance is that the original Irwin and Kerwin (Citation2005) project provided a substantial data base to examine in detail the initial trials of learning the dynamics of the longswing. Accordingly, all 25 participants were placed into a common practice and test group as shown in . Thus, there were 4 time points of testing (1 per week) spread over approximately a total time duration of 4 weeks and during each week there was also two training sessions.
Table 1. Testing and training schedule: Initial Assessment of subjects, ensuring physical ability and safety, followed by four Testing sessions interspersed by two training sessions over a four-week period
Practice Days: Each participant was taught the looped bar longswing on the high bar (), in a safe and controlled environment, on regulation equipment. The looped bar longswing is a common practice for the safe development of this skill (Readhead, Citation1997), where the participant is attached to a highly polished bar with nylon loops to ensure no falling. Each participant was provided with a practice period twice weekly for three weeks, during which time they practiced specific gymnastic progressions. Adequate rest between days was ensured. During the training period all participants were instructed not to perform any gymnastic type activity outside the designated days.
A qualified International level British Gymnastics coach carried out the coaching of the progressions. The coach had a maximum of 45 min x 2 sessions of progression development per week. During the 45 min session an equal amount of time was given to the practice of each progression and the order that each progression practiced was randomised. During the practice days the only feedback provided to the participants was related to the progressions themselves. It was emphasised that each progression was to be considered as an individual skill. Feedback was restricted to providing basic instruction, in terms of movement patterns and technique adjustments, thus providing both internal and external focus of attention (Readhead, Citation1997, and recommendations of the National Coach).
At the end of each day, each participant had the opportunity to attempt the full skill for the testing session. The progressions were all selected from those identified from the cohort of elite National coaches from previous studies (Irwin et al., Citation2004; Irwin & Kerwin, Citation2005, Citation2005). The longswing was taught from an “under the bar swinging” so the gymnast learned to interact with the apparatus and develop the skill in a safe fashion (Readhead, Citation1997).
Testing session: During all longswing attempts the performer started in the hang position and with the assistance of the National Gymnastics coach and was guided into the swing in a safe fashion (Readhead, Citation1997). The performer was then free to swing and generate as much angular motion as possible, and the highest swing during each trial was analysed. Training Day 1 provided a base line of each participant’s current ability. During the testing days each performer was recorded individually in isolation from the rest of the group.
For all trials across all testing days movement in the sagittal plane was recorded using a digital camcorder (Sony DSRPD1100AP, 3-CCD, Japan) placed approximately 40 m from the centre of the activity at a height of 5 m with its optical axis at 80° to the plane of motion (Irwin & Kerwin, Citation2005, Citation2007a, Citation2007b). The camera was operated at 50 Hz with the electronic shutter set to 1/300 s. Calibration of the performance area was achieved by placing a single calibration pole of height of 5.176 m, containing four 0.10 m spherical markers, at three pre-marked locations to form a plane of approximately 5 m x 5 m. The performer provided a verbal signal to indicate the onset of the actual longswing attempt.
The images of the calibration object and the gymnast were digitised using the high-resolution TARGET motion analysis system (Kerwin, Citation1995). Images of the calibration structure were digitised ten times. In each video field the centre of the bar, the centre of the gymnast’s head and the joint centres of the right wrist, elbow, shoulder, hip, knee, ankle, and toe were digitised. Camera calibration was achieved using an 8-parameter direct linear transformation (DLT) algorithm (Kwon, Citation1999), which provided the locations of the joint centres of rotation (Irwin & Kerwin, Citation2005, Citation2005, Citation2007b; Kwon, Citation1999).
Residuals were calculated for the horizontal and vertical locations of the knee, hip, shoulder, and wrist for each performer (Winter, Citation1990). Based on the residual analysis, a digital Gaussian Kernel filter with a bandwidth of 0.15 was implemented for random noise removal. Consistency both of the digitising process (reliability and objectivity) was determined through the repeated digitisation of the longswing during each of the data collections. Reliability was assessed through the cross product of the square root of the average difference squared (RMSD) between 5 repeated sequences during each of the data collections. Objectivity was assessed by comparing these 5 repeated sequences to another experienced digitiser. This same method was employed previously (Irwin & Kerwin, Citation2005, Citation2007a, Citation2007b).
Data analysis
In this study of learning the high bar longswing “circle” we emphasise investigating the acquisition of the qualitative dynamics of the skill as a function of practice and skill level. Thus, we were interested in characterizing the emergence of the pathway of change to the full circle of the CM configuration and a successful trial (complete full circle). The task outcome dependent variable was ΘMaxcircle, defining the radial swing amplitude of the participant’s CM about the bar. The gymnast’s circle angle was defined by the CM to neutral bar location θcircle (°).
The continuous relative phase difference between the CM angular position and velocity and the bar angular position and velocity relative to a shared origin () described the dynamics of the skill. The bar angle was defined by the bar position to a neutral bar projected against a vertical vector (θbar) (). The continuous relative phase of the CM-bar couple where first the phase plane portraits of the CM and bar were normalised to ±1 of the maximum rectified angle and angular velocity range (y axis). The phase angle was given by the arctangent of the angular velocity over the angular displacement for each of the points, within the range 0° ≤ φ ≤ 180° (Irwin et al., Citation2004). A negative out of phase motion was indicative of the bar leading the phase difference. Discrete points from the relative phase profile were focused on CRPθA (initiation of first out of phase sequence); CRPθB (initiation of second out of phase sequence) and CRPθC (end of second out of phase sequence.
Figure 2. Left: Schematic of CM and bar angular motion about the origin, defining the angle and angular velocity of the bar (θb, ωb) and CM (ΘCM, ωCM). Continuous relative phase of the bar – CM interaction (right) within a trial. CRPθA (initiation of first out of phase sequence); CRPθB (initiation of second out of phase sequence) and CRPθC (end of second out of phase sequence)
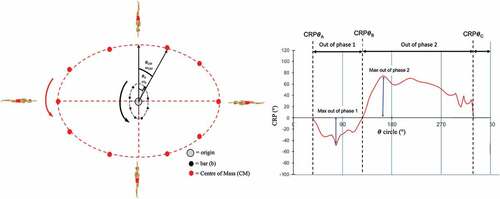
In addition, the radial angular velocity of the CM about the bar at 90° and 180° was analysed. The location of each performer’s CM was determined using a geometric model (Yeadon, Citation1990), with ratio correction to ensure correspondence between calculated and measured body masses. Based on the findings by Burton et al. (Citation2019) and Vicinanza et al. (Citation2018) the assumption was made that the completed repetitive full circle longswing of the novice gymnasts had a relatively low dimensionality approaching 1.0 plus noise, and hence the dynamics of the radial angular velocity of the CM about the bar against circle angle provided a description of this limit cycle attractor. The “functional” phases of the skill were defined as maximum hip extension to flexion and maximum shoulder flexion to extension, and identified when the relevant angular velocity was zero (Irwin & Kerwin, Citation2005).
Statistical analysis
All statistical analyses were performed on SPSSN v2.3 (SPSS Corporation, USA). Following checks for normality (normality was assessed using Kolmogorov-Smirnov, homogeneity of variance and sphericity were also checked) a repeated measures ANOVA (3 groups x 4 testing days) was performed on each of the variables of the continuous relative phase profile shown in . The 3 groups were identified based on their final testing session swing height and the qualitative (nominal) judgment of completing a full circle. The method related to previous work of Williams et al. (Citation2012, p. 2014ab, Citation2015). The 3-group categorization is consistent with: no change, small quantitative change, and qualitative change through completion of full circle. These categories fit current accounts of coordination and control and they are qualitatively different constructs that fit the respective group performance.
A step-wise multiple regression was performed to assess the relationship between the criterion variable ΘMaxcircle, and the predictor variables of continuous relative phase () and angular velocity of the CM at 90° and 180° around the bar. The data were checked to ensure that they satisfied the assumptions of auto correlation and multi collinearity. The step wise multiple regression identified those predictor variables most related to the criterion variable of maximum angular position about the bar (ΘMaxcircle).
Pairwise correlations were performed between all the predictor variables, including; CRPθA = initiation of first out of phase sequence; CRPθB = initiation of second out of phase sequence; CRPθC = end of second out of phase sequence; CRPΘB – CRPΘA = range of the first out of phase sequence; CRPΘB – CRPΘC = range of the second out of phase sequence; angular velocity of the CM about the bar at 90° (ω90°) and 180° (ω180°) around the bar. Alpha levels were set at P < 0.05. A Bonferroni correction was used and statistical significance was set to p = 0.05. Effect sizes were calculated via partial eta squared (ηp2; small >0.01, medium >0.05, large >0.14 (Cohen, Citation1973; Strutzenberger et al., Citation2020).
Results
Maximum angular position of the gymnast about the bar (ΘMaxcircle)
The outcome performance of the longswing skill was determined by the maximum angular position of the gymnast about the bar (ΘMaxcircle). The data produced three clusters of participants based on their performance level during the final testing day; Group 1: 346–360° (n = 7), Group 2: 291–316° (n = 10), Group 3: 263–283° (n = 8).
shows that Group 1 had the highest performance outcome level and improvement in circle performance completion. A two-way ANOVA of testing days (4) by groups (3) confirmed that there was a significant effect of testing days on ΘMaxcircle: F (3, 66) = 93.77, p < 0.05, ηp2 = 0.810) and of Group, F (2,22) = 87.416, p < 0.05, ηp2 = 0.888. The interaction between the Group and testing day was also significant on ΘMaxcircle: F (6, 66) = 11.266, p < 0.05, ηp2 = 0.506. In all cases, the effect sizes were large for this dependent variable.
Figure 3. Peak swing height, θcircle (°), for individual subjects (left) and Groups (right) for each testing day (1–4)
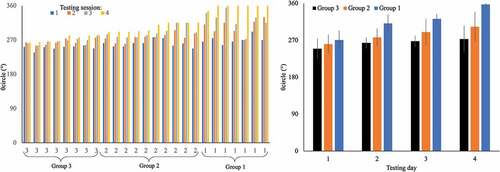
The analysis of simple main effects confirmed that the level of performance improvement over testing days was significantly greater for Group 1 than Group 2 and Group 3 (p < 0.05). At day 1, there was no difference between groups but the group effect became progressively larger over days. At day 2, there was a significant difference between Group 3 compared to Group 2 and Group 1, at day 3 and 4 all groups were different from each other (p < 0.05), with the Group 3 showing no improvement from the initial test 1 angular position prior to practice.
As the participants improved their performance outcome of circle height the angular trajectory of the CM changed across trials (). The progression of the CM rotation about the bar reveals how the longswing developed as a function of skill level. In addition, it shows that during this evolution of the CM angular position there is a continuous interaction with the bar. provides a qualitative illustration of the bar to CM relation as a function of exemplar individual performances over testing conditions.
Figure 4. Vertical (z) and horizontal (y) CM and bar (Bar) position during the testing session 1–4, for two participants from Group 3 (top two rows) and two participants from the more successful Group 1 (bottom two rows). Group average angular position of the participants at the start and end of the hip (red arrow) and shoulder (blue arrow) functional phases for Group 3 (top two rows) and Group 1 (bottom two rows)
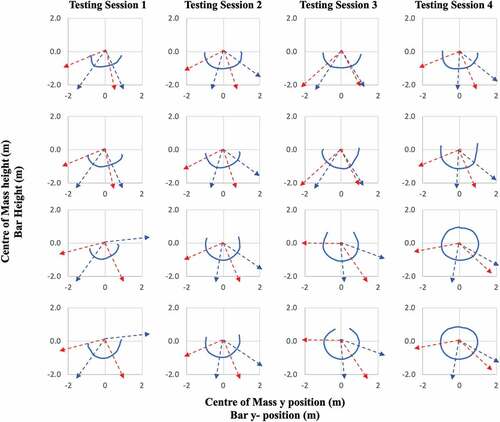
Radial Angular velocity of the CM at Θ90° and Θ180°
There was a significant effect of testing days for radial angular velocity of the CM at ω90° = F (3, 66) = 56.55, p < 0.05, ηp2 = 0.720 and ω180° = F (3, 66) = 31.093, p < 0.05, ηp2 = 0.586. and for Group, ω90° = F (2,22) = 74.05, p < 0.05, ηp2 = 0.871 ω180° = F (2,22) = 26.035, p < 0.05, ηp2 = 0.703. Effect sizes were all large, demonstrating a meaningful difference. Additionally, there was a significant interaction between group and testing day, ω90° = F (6, 66) = 8.079, p < 0.05, ηp2 = 0.423 ω180° = F (6, 66) = 2.616, p < 0.05, ηp2 = 0.192 (). The post hoc analysis showed that during the first testing Day there was no significant difference in ω90° and ω180°. However, at testing days 2, 3 and 4, Group 1 showed a significantly higher ω90° and ω180°, P < 0.05 (). Group 2 also showed a significant increase between testing day 2, 3 and 4.
Figure 5. CM Dynamics during the Testing Session 1–4, for one participant from Group 1 (top row) and one participant from the least successful Group 3 (bottom row)
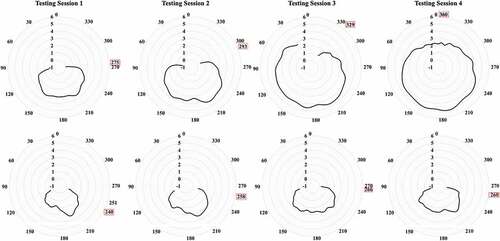
(top row) depicts the progressive increase in radial angular velocity over testing sessions by a subject from Group 1 who also went on to complete the circle. The bottom row in shows a subject from Group 3 that did not improve performance over testing sessions.
Circle motion dynamics
The radial angular velocity of the CM about the bar characterises the attractor dynamics of this skill as a limit cycle when the performer successfully completes a sequence of full longswing circles (Vicinanza et al., Citation2018). Given that only 7 novices completed the longswing through the full but single circle we do not have sufficient data to formally interpret in the dynamical context of a limit cycle. Nevertheless, we note that the circular plot from day 4 of the successful novice (and the other novices in group 3) looks qualitatively and quantitatively similar, including the properties of asymmetry, to those reported in Vicinanza et al. (Citation2018). Example circular plots of angular velocity against angle of CM motion are depicted in for a novice who completed the circle and one that did not over the 4 testing days.
shows that as the group 1 participant improved performance the angular velocity profile increased in area within the circular angular position about the clock frame of reference. Thus, completing the full circle was associated with increased velocity in the initial 180 degree free fall phase and the closing of the phase plane (P < 0.05). In contrast, the group 3 novice showed little change in angular velocity or improvement in circle height over the practice days. These examples reflect the qualitative difference in the angular velocity – position profile as a function of acquiring the full circle of the macroscopic CM variable.
Functional phases
The angular position of the participants at the start and end of the shoulders (SΘc_start, SΘc_end) and hips (HΘc_start, HΘc_end) functional phases is detailed in . These angular positions of the CM are also shown in .
Table 2. Mean (m) and SD (s) of the angular position of the participant (Θc) at the start and end of the Functional phases of the hips (HΘc_start, HΘc_end) and shoulders (SΘc_start, SΘc_end) and joint angle (Θ) at the start and end of the Functional phases of the hips (HΘstart, HΘend) and shoulders (SΘstart, SΘend) during testing session 1–4
SΘc start showed less than 5° of angular rotation difference between the three Groups at testing day 1, however, from start to end of the functional phase Group 1 moved through a greater range of motion. There was no clear pattern for SΘc_start or SΘc_end. The angular position at the start and end of the hip functional phase (HΘc_start and HΘc_end) shifted earlier in the circle angle as testing day progressed from 1–4.
A key finding was that Group 1 showed a greater range of angular motion about the bar for the hips functional phase as these participants become more successful (, ) (P < 0.05). The joint angular kinematics of the participants at the start and end of the shoulders (SΘstart, SΘend) and hips (HΘstart, HΘend) functional phases are also included in and . The shoulder joints showed a greater range of motion between Group 1 and the other two groups from Day 1 to 3 of testing. Interestingly, on the final testing session there were very similar joint motions between groups at the shoulders.
Continuous relative phase (CRP)
The continuous relative phase (CRP) between the bar and the gymnast’s CM provides a measure of the macroscopic task dynamics, and reflects the candidate collective variable. Key phases of the CRP profile within a circle trial are defined in and show the dependent variables that were derived from this dynamic and also the variables that lead the coupling CRPΘA = start of the bar leading and CRPΘB start of the CM leading.
Continuous relative at CRPΘA. For the initial time point of out of phase coupling, CRPΘA, a two-way ANOVA of testing days (4) by groups (3) showed that there was a significant effect for testing days: (F (3, 66) = 116.425, p < 0.05, ηp2 = 0.841) and for Group, F (2,22) = 206.60, p < 0.05, ηp2 = 0.949. The interaction between the Group and testing day was also significant for Θ CRPΘA: F (6, 66) = 14.209, p < 0.05, ηp2 = 0.841. Across these tests of difference for CRPΘA effect sizes were all large.
The post hoc analysis of the interaction of group and testing day showed that at day 1 the initiation of the out of phase coupling was similar for Groups 2 and 3, however, Group 1 initiated this phase at a significantly earlier angular position about the bar (circle angle) (P < 0.05) on day 1. The differences in the initiation of the out of phase coupling (CRPΘA) were also progressively earlier in the trajectory for Group 1 (P < 0.05) compared to the Groups 2 and 3 as the testing days progressed. By the final testing day, Group 1 had a significantly earlier initiation of the out of phase motion (p < 0.05) ( and ).
Figure 6. Group average for key continuous relative phase variables as a function of practice and skill level group
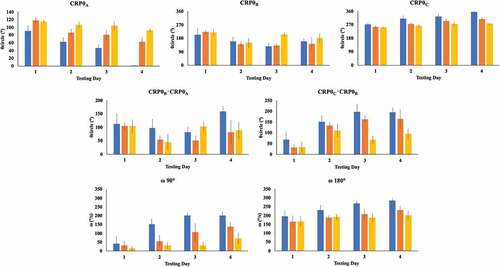
Figure 7. Individual participants continuous relative phase (CRP) of the CM – bar couple on testing day 1 and 4, against angular position of the gymnasts about the bar (θcircle). Highlighted are CRPθA (initiation of first out of phase sequence); CRPθB (initiation of second out of phase sequence) and CRPθC (end of second out of phase sequence). Top row represents group 3, middle row = group 2, bottom row = group 1
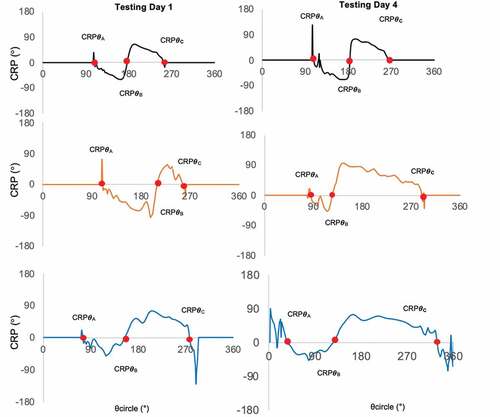
Continuous relative phase CRPΘB. The start of the second segment of out of phase coupling occurs at (CRPΘB) and during this couple the motion of the bar leads that of the CM. There was a significant effect for testing days CRPΘB: F (3, 66) = 23.46, p < 0.05, ηp2 = 0.516 and for Group, F (2,22) = 13.70, p < 0.05, ηp2 = 0.555. Additionally, a significant interaction between group and testing day was found, F (6, 66) = 4.144, p < 0.05, ηp2 = 0.247. Across these tests of difference for CRPΘB effect sizes were all large. The post hoc analysis of the interaction of Group and Day showed that during the first two testing Days there was no significant difference in the angular position of the performer at CRPΘB between any group. However, at testing day 3 and 4 Groups 1 and 2 had a significantly earlier angular position at this phase (CRPΘB) ( and ).
Continuous relative phase CRPΘC. The angular position of the performer at the end of the second segment of the out of phase coupling was also analysed (CRPΘC). CRPΘC and showed a significant effect for testing days (F (3, 66) = 89.29, p < 0.05, ηp2 = 0.795), and group (F (2,22) = 146.62, p < 0.05, ηp2 = 0.930). In addition, there was a significant interaction between group and testing day, F (6, 66) = 7.44, p < 0.05, ηp2 = 0.403. The effect sizes for all these tests of difference were large. The post hoc analysis of the interaction showed a later (P < 0.05) end to the second out of phase motion RPΘC for group 1 compared to groups 2 and 3 at the initial and second testing day. On Days 3 and 4 Group 3 had not changed the location of CRPΘC and was significant earlier that groups 3 and 1.
Range CRPΘB – CRPΘA . The range of the angular position the performer realised during the first (CRPΘB – CRPΘA) and second (CRPΘC – CRPΘB) out of phase motion was quantified. During the first phase CRPΘB – CRPΘA) the range of this out of phase coupling showed a significant effect for testing days (F (3, 66) = 12.75, p < 0.05, ηp2 = 0.367), and group (F (2,22) = 16.32 p < 0.05, ηp2 = 0.597). In addition, a significant interaction between group and testing day was also found F (6, 66) = 4.744 p < 0.05, ηp2 = 0.301. The post hoc analysis of the interaction showed that at day 1 all groups were similar in terms of the range of CRPΘB – CRPΘA. In contrast, by the final testing day Group 1 was significantly longer in the range of CRPΘB – CRPΘA than Groups 2 and 3 (P < 0.05). Interestingly, Group 3 did not significantly change the range of this phase across the testing days ().
Range CRPΘB – CRPΘC. The range of the angular position for the performer during the second out of phase motion was described by CRPΘB – CRPΘC. The range of this out of phase coupling showed a significant effect for testing days, (F (3, 66) = 57.56, p < 0.05, ηp2 = 0.724), and group, (F (2,22) = 91.84 p < 0.05, ηp2 = 0.893. In addition, there was a significant interaction between group and testing day, F (6, 66) = 5.22 p < 0.0, ηp2 = 0.322. The post hoc analysis of the interaction revealed that Groups 1 and 2 underwent significantly greater change in angular position in days 3 and 4 compared to group 3 (P < 0.05), ( and ). Across these tests of difference for Range CRPΘB – CRPΘA and Range CRPΘB – CRPΘC CRPΘA effect sizes were all large.
Correlation of key variables using individual trial data
Multiple stepwise regression was used to examine the relationship between the criterion variable swing height (ΘMaxcircle) and ω90°, ω180° around the bar and the key phases of the bar – body CM couple (). The data satisfied the assumptions of auto correlation, normality and multi collinearity () and the correlation analyses were all run with individual trial data.
Table 3. Multiple step wise regression with maximum swing height (ΘMaxcircle) as the dependent variables
Table 4. Multiple step wise regression coefficients, constant and Beta (B). θMaxcircle as the criterion variable and each model relates to a specific testing day
The key finding was that the onset of the first out of phase sequence (CRPΘA) was significantly related to an increased ΘMaxcircle throughout the testing days (1–4), whereby the earlier this occurred in the circle motion the greater the ΘMaxcircle. During testing day 2 ω180° was significantly related to the increase in ΘMaxcircle with a high contribution to this relationship. Additionally, during testing day 2 and 3 ω90° significantly contributed to ΘMaxcircle, during Day 3 ω90° showed a particularly high contribution to this relationship (P < 0.05). On the final testing day two variables were found to significantly corelate Maxcircle namely CRPΘA and CRPΘC with the latter showing a greater contribution to the overall success, highlighting the importance of the later phases (P < 0.05). Thus, early in practice the angular velocity at 90°, and 180°, tended to dominate the variance but by day 4 it was the relative phase measures that dominated in a very high multiple correlation value (r = .93).
Linear pairwise correlations were also examined between all the dependent variables as a function of testing day to observe the strength of these relationships. shows these relations in a correlation matrix based on all the 25 participants and the key variables discussed previously.
Table 5. Linear regression matrix of the key variables associated with swing height
The relation between CRPΘA and both ω90° and ω180° became significant by day 4 (P < 0.05), and the same change in relation was observed for CRPΘC. Additionally, the relation between ΘMaxcircle and CRPΘA and CRPΘC increased between testing day 1 and 4 consistent with the importance of these variables to successful performance. The two relative phase variables CRPΘA and CRPΘC showed an increase in the strength of the relation from −0.661 to −0.921 between testing day 1 and 4, respectively.
Discussion
The experiment examined novice male gymnasts learning through practice to perform for the first time a complete longswing cycle on the high bar. Our focus was on the emergence of the formation of the movement pattern of the longswing and the coupling dynamics of the bar and CM – a candidate collective variable (Kelso, Citation1995; Mitra et al., Citation1998; Newell & Liu, Citation2020) for the task. A previous analysis of the task dynamics had revealed that one task constraint for the longswing is the attainment of a complete limit cycle of the CM angular velocity that needs to be above an implicit minimum level to sustain oscillation of the body around the full circle (Vicinanza et al., Citation2018). Here, our findings showed the presence of individual pathways of change in novices learning to form the qualitative angular dynamics and the associated coupling dynamics of the bar and CM motions.
Bar-body CM relative phase dynamics
The dynamics of the motions of the high bar and CM of the gymnast were determined along with how practice and task performance related to their coupling. The bar has been identified as contributing a relatively small source of system energy but it has not been formally linked to the dynamics of the CM (Fukushima & Russell, Citation1980; Readhead, Citation1997). We examined the hypothesis that with practice the novice gymnast forms the coordination pattern of the longswing as reflected by the coupling of CM and bar motions.
Coordination dynamics holds that an order parameter or collective variable is a low dimensional macroscopic variable that captures the spatial-temporal structure of the action (Kelso, Citation1995; Mitra et al., Citation1998; Newell & Liu, Citation2020). In standing on an oscillating platform, for example, the CM and/or the relative phase of CM-CoP have been postulated as the collective variable (Ko et al., Citation2014). The variable in the longswing that is complementary to this approach is the relative phase of the motions of the CM to those of the bar.
The analysis of the CM and high bar coupling by continuous relative phase revealed a consistent pattern through the longswing circle even when the longswing was not complete (see and ). Furthermore, skill level changed the ratio of the bar leading antiphase coupling segment of the initial 180 degrees to that of the CM leading in the second half of the circle. The more successful novices had close to a 2:1 ratio of CM and the high bar and an earlier onset of the bar leading the CRP phases (CRPΘA,). With practice the in-phase relation emerges which contributes to enhancing the longswing performance and the effectiveness and efficiency of producing a complete full circle on the high bar. In progressions to the most advanced stage of skill learning the hypothesis of Bernstein (Citation1967) is that the gymnast would further exploit in a positive way the reactive forces of the bar.
The relative phase analysis revealed that there is a cyclical structure to the coupling of CM and bar through the execution of a complete longswing cycle. Nevertheless, we do not have the range of control parameter manipulations on the stability/instability of the longswing to formally evaluate the interpretation of CM-bar relative phase as a collective variable (Kelso, Citation1995; Newell & Liu, Citation2020). Nevertheless, the CM-bar relative phase captures the systematic qualitative relation of CM to bar motion as a function of skill level.
The relatively long practice duration required for some learners “to get the idea of the task” can be considerable as shown in studies of learning to: ride a unicycle (Lee et al., Citation2016), 3-ball cascade juggle (Beek & Van Santvoord, Citation1992), and implement the roller ball task (Liu et al., Citation2019, Citation2010; Newell et al., Citation2006). Indeed, in the Lee et al. (Citation2016) unicycle study 2 of the young adults failed to successfully ride the unicycle even after 28 daily sessions of practice. We interpret these general departures from the monotonic continuity of the standard exponential/power law motor learning curves to be due to the differential task constraints and their influence on the change in the nonlinear system dynamics as a function of adaptation and learning of the multiple redundant degrees of freedom (Newell et al., Citation2001; Warren, Citation2006).
Completing the full circle in the longswing reflects the emergence of the macroscopic coordination pattern required in this task. This achievement can be interpreted to reflect the initial stage of “getting the idea” of the task (Fitts, Citation1964; Gentile, Citation1972), but is different to what previously has been taken as the cognitive phase. In the dynamical perspective the initial stage of motor learning is about forming a task relevant coordination pattern (Mitra et al., Citation1998; Newell, Citation1985). Tasks that require the learner to produce a novel coordination mode, never previously produced, tend to show as a function of practice a transition in the dynamics of forming the movement pattern and/or the outcome (Liu et al., Citation2019, Citation2010).
In the longswing task, the transition is from an incomplete longswing circle to a full circle, and eventually through sufficient practice, to generate limit cycle attractor dynamics to performer a sequence of longswings. This is a nominal qualitative change although quantitative assessment of the degree of departure from completing the circle can be derived (Vicinanza et al., Citation2018). Thus, depending on the variable and frame of reference there is both continuous and discontinuous change in coordination and control over practice and learning of the longswing coordination movement pattern.
Our experimental focus has been on the initial early stage of learning a single circle of the high bar over the practice period. We recognise that at the competition level of gymnastics, a sub goal is usually more than one consecutive cycle of the long swing studied here. The influence of previous longswing cycles on subsequent cycles of the same and different performance trials remains to be examined as does the continued progressive change (refining) of the attractor dynamics of elite gymnasts.
Radial angular velocity of CM
Vicinanza et al. (Citation2018) reported a limit cycle dynamical analysis of the CM radial angular velocity of a single longswing circle as a function of skill group – novices through to an international level gymnast. CM was selected for this analysis as it is a macroscopic biomechanical variable of the gymnast in motion. And, in light of other studies of the macroscopic organization of postural control, might be considered a candidate collective variable for the task (Haken et al., Citation1985; Ko et al., Citation2014).
The analysis of Vicinanza et al. (Citation2018) showed that the limit cycle dynamics for CM angular velocity formed a closed trajectory for the complete full longswing cycle with the velocity not symmetrical around the circle. The pronounced asymmetry of the angular velocity through the circle was particularly prevalent due to the impact of the gravity driven fast free fall of the 0–180 degree segment, in addition to segments of the circle that reflected the influence of a transition in control processes that were differentially emphasised in particular aspects of the circle. Here we analysed the angular velocity of CM in all participants because many of the novice trials were not complete circles so that the limit cycle dynamics of many trials was not realised. The data of on the evolving longswing show that the motion of the CM in space appears more symmetrical (circular) than in the limit cycle frame of reference with the inclusion of velocity. also shows a relation between the height of initiating the free fall in the first segment and the height obtained in the final phase. This reflects the important impact of the initial angular momentum in producing a complete circle as supported by the corroborative findings of the multiple regression analysis.
The findings showed that the more accomplished novice gymnasts of Group 1 tended to take less time in the initial fall trajectory to reach both 90° and 180°. This implies that the angular momentum generated from the initial free fall was already higher than that of the less skilled subjects as the CM passed the minimum at 180° and into the upward motion counter gravity segment of the longswing – what has been called the functional phase (Irwin & Kerwin, Citation2005). Enhanced radial angular velocity from the free fall taken into the upward segment would place less demand on additional energy input via musculoskeletal interaction to compensate for loss of momentum and realise completion of the full circle.
Practice and learning
The findings showed that not all participants were successful in completing a full longswing cycle over the practice time and learning conditions. Only 7 of the 25 novice gymnasts completed one or more full longswing cycles over the practice days. It would be expected that with further deliberate practice (Ericsson, Citation2019; Ericsson et al., Citation1993) more (perhaps all) participants would complete a full cycle on the high bar. Nevertheless, these data are consistent with other reports on adults learning a new coordination pattern (Liu et al., Citation2019) in that they depart from the monotonic exponential and power law accounts that have dominated the analysis of learning curves (cf. Newell et al., Citation2006). Indeed, group 3 with the poorest performers, showed very little change in behaviour over the practice and testing days. This resistance to change reflects, in contrast to movement scaling tasks (Newell, Citation1985), the relative difficulty of inducing and predicting a functional transition requiring the emergence of a stable qualitative solution from an unstable dynamic. The companion paper II shows that the individual differences in attaining a complete limit cycle are strongly linked to delaying the timing of the energy input into the upward trajectory within the 180°-270° circle segment.
Implications for the sport of gymnastics
The macroscopic aspects of the coordination and control of movement have theoretical and practical implications for both the perception and action of gymnastics. For example, studies of the visual perception of biological motion have shown that humans have a strong adeptness to perceive macroscopic properties of movement in identifying action categories and even individual characteristics in motion (Johansson, Citation1973; Scully, Citation1986; Scully & Newell, Citation1985), including those characteristics that support a judge’s perception and scoring of a gymnastic routine. On the action front, gymnastics affords the task structure to examine in a systematic way Bernstein’s (Citation1967) degrees of freedom problem and the related search for the relevant collective and control variables.
The novel feature of this paper is twofold. Firstly, it contributes to knowledge of skill learning and the evolution of a macroscopic variable but reveals the difficulty in identifying the collective variable(s) (Newell & Liu, Citation2020). We suspect that coaches of gymnastics have viable frameworks to formalise the identification and place of collective variables in coaching gymnastics and teaching physical activity more generally. Nevertheless, the standard instructional strategies for motor learning could be tested more directly on the collective variable construct. Secondly, having knowledge of the macroscopic variables provides a platform for coaches to undertake functionally relevant task analysis. Task analysis helps build the conceptual understanding of the target skill (Irwin et al., Citation2005) that is a key part of the coaching process. Based on the training principles the coach uses this strategy to facilitate the development of drills and physical preparation, hence the importance of the task analysis.
Finally, in this paper we have highlighted a candidate collective variable as the phase relation between the motion of the bar and that of the CM. There is a need to return to the longswing and examine further the mechanisms used to maintain the angular motion and explore how the gymnast manages the constraints of the bar and gravity to achieve the overall performance goal. Our companion Paper II examines the evolution of the biomechanical energetic interaction with a focus on the emergence of the task dynamics and the collective variable (Newell & Liu, Citation2020).
Disclosure statement
No potential conflict of interest was reported by the author(s).
References
- Anderson, D. I., & Sidaway, B. (1994). Coordination changes associated with practice of a soccer kick. Research Quarterly for Exercise and Sport, 65(2), 93–99. https://doi.org/10.1080/02701367.1994.10607603
- Arampatzis, A., & Brüggemann, G. P. (1998). A mathematical high bar- human body model for analysing and interpreting mechanical - energetic processes on the high bar. Journal of Biomechanics, 31(12), 1083–1092. https://doi.org/10.1016/S0021-9290(98)00134-1
- Arampatzis, A., & Brüggemann, G. P. (2001). Mechanical energetic processes during the giant swing before the Tkatchev exercise. Journal of Biomechanics, 34(4), 505–512. https://doi.org/10.1016/S0021-9290(00)00212-8
- Bauer, W. L. (1983). Swinging as a way of increasing the mechanical energy in gymnastic manoeuvres. In H. Matsui & K. Kobayashi (Eds.), Biomechanics vol VIII-B (pp. 801–807). Human Kinetics.
- Beek, P. J., & Van Santvoord, A. A. (1992). Learning the cascade juggle: A dynamical systems analysis. Journal of Motor Behavior, 24(1), 85–94. https://doi.org/10.1080/00222895.1992.9941604
- Bernstein, N. (1967). The co-ordination and regulation of movement. Pergamon.
- Burton, S., Vicinanza, D., Exell, T., Williams, G. K. R., Newell, K. M., & Irwin, G. (2019). Limit cycle dynamics across elite male artistic gymnasts. International Society of Biomechanics in Sport, Proceedings Archive, 37(1). Available at https://commons.nmu.edu/isbs/vol37/iss1/35
- Busquets, A., Marina, M., & Angulo-Barroso, R. M. (2013a). Changes in motor strategies across age performing a longswing on the high bar. Research Quarterly for Exercise and Sport, 84(3), 353–362. https://doi.org/10.1080/02701367.2013.810537
- Busquets, A., Marina, M., Irurtia, A., & Angulo-Barroso, R. M. (2013b). Coordination analysis reveals differences in motor strategies for the high bar longswing among novice adults. PLos ONE, 8(6), e67491. https://doi.org/10.1371/journal.pone.0067491
- Busquets, A., Marina, M., Irurtia, A., Ranz, D., & Angulo-Barroso, R. M. (2011). High bar swing performance in novice adults: Effects of practice and talent. Research Quarterly for Exercise and Sport, 82(1), 9–20. https://doi.org/10.1080/02701367.2011.10599717
- Button, C., Seifert, L., Chow, J. Y., Araüjo, D., & Davids, K. (2021). Dynamics of skill acquisition: An ecological dynamics approach (2nd Ed.). Human Kinetics.
- Cohen, J. (1973). Eta-squared and partial eta-squared in fixed factor anova designs. Educational and Psychological Measurement, 33, 107–112. doi:10.1177/001316447303300111
- Ericsson, K. A. (2019). Towards a science of the acquisition of expert performance in sports: Clarifying the differences between deliberate practice and other types of practice. Journal of Sports Sciences, 38(159–176). https://doi.org/10.1080/02640414.2019.1688618
- Ericsson, K. A., Krampe, R. T., & Tesch-Romer, C. (1993). The role of deliberate practice in the acquisition of expert performance. Psychological Review, 100(3), 363–406. https://doi.org/10.1037/0033-295X.100.3.363
- Fitts, P. M. (1964). Perceptual-motor skill learning. In A. W. Melton (Ed.), Categories of human learning (pp. 243–285). Academic Press.
- Fukushima, S., & Russell, W. (1980). Men’s gymnastics. Faber & Faber.
- Gentile, A. M. (1972). A working model of skill acquisition with application to teaching. Quest, 17, 3–23.
- Haken, H., Kelso, J. A. S., & Bunz, H. A. (1985). A theoretical model of phase transitions in human hand movements. Biological Cybernetics, 51(5), 347–356. https://doi.org/10.1007/BF00336922
- Hiley, M. J., & Yeadon, M. R. (2003). Optimum technique for generating angular momentum in accelerated backward giant circles prior to a dismount. Journal of Applied Biomechanics, 19(2), 119–130. https://doi.org/10.1123/jab.19.2.119
- Irwin, G., Hanton, S., & Kerwin, D. G. (2004). Elite coaching I: The origins of elite coaching knowledge. Reflective Practice, 5(3), 419–436. https://doi.org/10.1080/1462394042000270718
- Irwin, G., Hanton, S., & Kerwin, D. G. (2005). The conceptual process of skill progression development in artistic gymnastics. Journal of Sports Sciences, 23(10), 1089–1099. https://doi.org/10.1080/02640410500130763
- Irwin, G., & Kerwin, D. G. (2005). Gymnastics. Sports Biomechanics, 4(2), 164–178. https://doi.org/10.1080/14763140508522861
- Irwin, G., & Kerwin, D. G. (2007a). Musculoskeletal demands of progressions for the longswing on high bar. Sports Biomechanics, 6(3), 361–374. https://doi.org/10.1080/14763140701491336
- Irwin, G., & Kerwin, D. G. (2007b). Inter-segmental co-ordination of high bar progressions. Sports Biomechanics, 6 (2) 131–144.DOI:https://doi.org/10.1080/14763140701323117
- Irwin, G., Williams, G., & Newell, K. (2019). The dynamics of coordination, control and skill in sport. In: Proceedings of the 37th International Conference on Biomechanics in Sports, Miami, USA, International Society of Biomechanics in Sport, Proceedings Archive, 37(1). Available at https://commons.nmu.edu/isbs/
- Johansson, G. (1973). Visual perception of biological motion and a model for its analysis. Perception and Psychophysics, 14(2), 201–211. https://doi.org/10.3758/BF03212378
- Kelso, J. A. S. (1995). Dynamic patterns: The self-organization of brain and behavior. MIT Press.
- Kelso, J. A. S. (2021). Unifying large- and small-scale theories of coordination. Entropy, 23(5), 537. https://doi.org/10.3390/e23050537
- Kerwin, D. G. (1995). Apex/Target high-resolution video digitizing system. In J. Watkins (ed.), Proceedings of the Sports Biomechanics Section of the British Association of Sports and Exercise Sciences (pp. 1–4). Leeds: British Association of Sports and Exercise Sciences.
- Kerwin, D. G., & Hiley, M. J. (2003). Estimation of reaction forces in high bar swinging. Sports Engineering, 6(1), 2–30. https://doi.org/10.1007/BF02844157
- Ko, J.-H., Challis, J. H., & Newell, K. M. (2014). Transition of COM–COP relative phase in a dynamic balance task. Human Movement Science, 38, 1–14. https://doi.org/10.1016/j.humov.2014.08.005
- Kugler, P. N., Kelso, J. A. S., & Turvey, M. T. (1980). On the concept of coordinative structures as dissipative structures: I. Theoretical lines of convergence. In G. E. Stelmach & J. Requin (Eds.), Tutorials in motor behavior (1-49). North–Holland.
- Kwon, Y. H. (1999). 2D Object plane deformation due to refraction in two dimensional underwater motion analysis. Journal of Applied Biomechanics, 15(4), 396–403. https://doi.org/10.1123/jab.15.4.396
- Lee, I.-C., Liu, Y.-T., & Newell, K. M. (2016). Learning to ride a unicycle: Coordinating balance and propulsion. Journal of Motor Learning and Development, 4(2), 287–306. https://doi.org/10.1123/jmld.2015-0030
- Liu, Y.-T., Chuang, K.-L., Newell, K. M., & Haddad, J. M. (2019). Mapping collective variable and synergy dynamics to task outcome in a perceptual-motor skill. PloS One, 14(4), e0215460. https://doi.org/10.1371/journal.pone.0215460
- Liu, Y.-T., Mayer-Kress, G., & Newell, K. M. (2010). Bi-stability of movement coordination as a function of skill level and task difficulty. Journal of Experimental Psychology: Human Perception and Performance, 36, :1515–1524. https://doi.org/10.1037/a0018734
- Liu, Y.-T., Mayer-Kress, G., & Newell, K. M. (2006) Qualitative and quantitative change in the dynamics of motor learning. Journal of Experimental Psychology: Human Perception and Performance, 32, 380–393. https://doi.org/10.1037/0096-1523.32.2.380
- Mitra, S., Amazeen, P. G., & Turvey, M. T. (1998). Intermediate motor learning as decreasing active (dynamical) degrees of freedom. Human Movement Science, 17(1), 17–65. https://doi.org/10.1016/S0167-9457(97)00023-7
- Newell, K. M. (1985). Coordination, control and skill. In D. Goodman, I. Franks, & R. Wilberg (Eds.), Differing perspectives in motor learning, memory and control (pp. 295-317). North-Holland.
- Newell, K. M., & McDonald, P. V. (1994). Learning to coordinate redundant biomechanical degrees of freedom. In S. Swinnen, H. Heuer, J. Massion, & P. Casaer (Eds.), The control and modulation of patterns of inter-limb coordination: A multidisciplinary perspective (pp. 515-536). Academic Press.
- Newell, K. M., & Liu, Y.-T. (2020). On collective variables and task constraints in movement coordination, control and skill. Journal of Motor Behavior, Oct 26, 1–27. https://doi.org/10.1080/00222895.2020.1835799
- Newell, K. M., Liu, Y.-T., & Mayer-Kress, G. (2001). Time scales in motor learning and development. Psychological Review, 108(1), 57–82. https://doi.org/10.1037/0033-295X.108.1.57
- Newell, K. M., Mayer-Kress, G., & Liu, Y.-T. (2006). Human learning: Power laws or multiple characteristic time scales? Tutorials in Quantitative Methods for Psychology, 2(2), 66–76. https://doi.org/10.20982/tqmp.02.2.p066
- Nourrit, D., Delignières, D., Caillou, N., Deschamps, T., & Lauriot, B. (2003). On discontinuities in motor learning: A longitudinal study of complex skill acquisition on a ski-simulator. Journal of Motor Behavior, 35(2), 151–170. https://doi.org/10.1080/00222890309602130
- Okamoto, A., Sakurai, S., Ikegami, Y., & Yabe, K. (1987). Mechanical work in the giant swing on the horizontal bar. In G. de Groot, A. P. Hollander, P. A. Huijing, & G. J. van Ingen Schenau (Eds.), Biomechanics XI-B (pp. 758–762). Free University Press.
- Readhead, L. (1997). Men’s gymnastics coaching manual. Crowood Press.
- Scully, D. M. (1986). Visual perception of technical execution and aesthetic quality in biological motion. Human Movement Science, 5(2), 185–206. https://doi.org/10.1016/0167-9457(86)90024-2
- Scully, D. M., & Newell, K. M. (1985). Observational learning and the acquisition of motor skills. Journal of Human Movement Studies, 11, 169–186.
- Sheets, A. L., . &., & Hubbard, M. (2009). Influence of optimization constraints in uneven parallel bar dismount swing simulations. Journal of Biomechanics, 42(11), 1685–1691. https://doi.org/10.1016/j.jbiomech.2009.04.014
- Sparrow, W. A., & Irizarry-Lopez, V. M. (1987). Mechanical efficiency and metabolic cost as measures of learning a novel gross motor task. Journal of Motor Behavior, 19(2), 240–264. https://doi.org/10.1080/00222895.1987.10735410
- Strutzenberger, G., Edmunds, R., Nokes, L. D., Mitchell, I. D., Mellalieu, S. D., & Irwin, G. (2020). Player–surface interactions: Perception in elite soccer and rugby players on artificial and natural turf. Sports Biomechanics, 4, 1–11. https://doi.org/10.1080/14763141.2020.1720279
- Turvey, M. T. (1990). Coordination. American Psychologist, 45(8), 938–953. https://doi.org/10.1037/0003-066X.45.8.938
- Vicinanza, D., Newell, K. M., Irwin, G., Smith, L., & Williams, G. K. R. (2018). Limit cycle dynamics of the gymnastics longswing. Human Movement Science, 57, 217–226. https://doi.org/10.1016/j.humov.2017.12.014
- Warren, W. H. (2006). The dynamics of perception and action. In Psychological Review 113(2), 358–389.
- Williams, G., Irwin, G., Kerwin, D. G., & Newell, K. M. (2012). Kinematic changes during learning the longswing on high bar. Sports Biomechanics, 11(1), 20–33. https://doi.org/10.1080/14763141.2011.637120
- Williams, G., Irwin, G., Kerwin, D. G., & Newell, K. M. (2015a). Changes in joint kinetics during learning the longswing on high bar. Journal of Sports Sciences, 33(1), 29–38. https://doi.org/10.1080/02640414.2014.921831
- Williams, G., Irwin, G., Kerwin, D. G., & Newell, K. M. (2015b). Biomechanical energetic analysis of technique during learning the longswing on the high bar. Journal of Sports Sciences, 33(13), 1376–1387. https://doi.org/10.1080/02640414.2014.990484
- Winter, D. A. (1990). Biomechanics of human movement. Wiley.
- Wu, Y.-H., & Latash, M. L. (2014). The effects of practice on coordination. Exercise and Sport Sciences Reviews, 42(1), 37–42. https://doi.org/10.1249/JES.0000000000000002
- Yeadon, M. R. (1990). The simulation of aerial movement—II. A mathematical inertia model of the human body. Journal of Biomechanics, 23(1), 67–74. https://doi.org/10.1016/0021-9290(90)90370-I
- Yeadon, M. R., & Hiley, M. J. (2000). The mechanics of the backward giant circle on the high bar. Human Movement Science, 19(2), 153–173. https://doi.org/10.1016/S0167-9457(00)00008-7
- Zanone, P. G., & Kelso, J. A. S. (1992). Evolution of behavioral attractors with learning: Nonequilibrium phase transitions. Journal of Experimental Psychology: Human Perception and Performance, 18(2), 403–421. https://doi.org/10.1037/0096-1523.18.2.4