Abstract
The demand of enzymes in industrial sectors is increasing rapidly due to their economical and ecological advantages. Micro-organisms produce different types of extracellular enzymes for maintaining their own metabolism, defense, and normal physiological condition. Among several enzymes, proteases have gained special attention in industrial sectors. Several sources of extracellular enzymes are reported by various researchers, but enzymes obtain from microbial sources have high demand in industries due to lower cost, high production rate, availability, stability, and diversity. Among micro-organism, bacteria and fungi are reported to be good sources of different types of proteases such as alkaline protease, cysteine protease, aspartate protease, and metallo protease. In this review, we have summarized the available information about the sources of bacterial and fungal proteases, their purification strategies and their temperature and pH optima. Due to huge competition, companies are trying to reduce their manufacturing cost and that’s why microbial sources of enzymes are important. However, genetically engineered strains or engineered proteases have much more importance over natural isolates/protease in industries due to higher production rate and other advantages. Here we have also summarized the important applications of protease in different industries such as, paper mill, starch degrading sector, food processing factories, and detergent making companies.
Introduction
Enzymes are biological macromolecules that catalyze the chemical reactions. Dr Anselme Payen, the French chemist who first discovered the enzyme diastase in 1833. A few decades later, Louis Pasteur concluded that a vital force converts the sugar into alcohol during fermentation and termed it as ‘ferments’. At last, Dr Wilhelm Kühne first coined the term enzyme in 1877. Nowadays, the demands of enzymes as biocatalysts in various sectors are increasing rapidly, due to several advantages such as (i) efficiency (work at lower concentration), (ii) selectivity (positional, chiral, and functional), (iii) mild reaction condition (lower pH and temperature), (iv) modification (can be modified to enhance activity and stability), and environment friendly nature. The industries that consume the major amount of enzymes include food processing industries, beverage, textile, leather, bio-fuel, detergent, and paper industries. According to Jemli, Ayadi-Zouari, Hlima, and Bejar (Citation2016), the hydrolases are the most used industrial enzymes till date. Among these enzymes (amylase, cellulase, phytase, lipase, chitinase), protease receive special attention due to their potential application in various industries, including biotechnological and medical sectors (Gupta, Beeg, Khan, & Chauhan, Citation2002; van der Maarel, van der Veen, Uitdehaag, Leemhuis, & Dijkhuizen, Citation2002; Rajagopalan & Krishnan, Citation2008; Husain, Citation2010; Padmapriya & Williams, Citation2012; Souza et al., Citation2015). A diverse group of plants, animals, and microbiota are reported to be good source of enzymes. Though, people are interested in enzymes obtain from bacteria and fungi due to lower production cost, extracellular nature, availability, and stability (Gupta, Gigras, Mahapatra, Goswami, & Chauhan, Citation2003; Banerjee, Mukherjee, Bhattacharya, & Ray, Citation2016). Subba Rao, Sathish, Ravichandra, and Prakasham (Citation2009) have stated that the approximate value of industrial enzymes was $220 billion in 2009. Industrial enzymes produced in bulk, generally require little downstream processing, and hence are relatively crude preparations. The commercial use of these enzymes does not require purification but enzymes used in pharmaceutical and clinical sectors require high purity.
Proteolytic enzymes are ubiquitous in occurrence, present in all living organisms and essential for cell growth, metabolism, cell signaling, differentiation and other physiological purpose. Enzymes derived from micro-organisms may be located intracellular, periplasmic, or extracellular (Kohlmann, Nielsen, Steenson, & Ladisch, Citation1991; Ray, Ghosh, & Ringø, Citation2012). Proteases are considered to be the most important classes of industrial enzymes and account for nearly 60% of the total enzyme sale globally (Escobar & Barnett, Citation1993; Paul et al., Citation2016). In this direction, Beg and Gupta (Citation2003) have reported that microbial proteases have highest commercial value over enzymes obtained from animals and plants sources. A wide group of bacteria and fungi produce extracellular proteases (Raut, Sen, Kabir, Satpathy, & Raut, Citation2012; Sharma et al., Citation2015; Souza et al., Citation2015), however most of the protease producing candidates belongs to Bacillus species such as B. subtilis ATCC 14416 (Bhunia, Basak, & Dey, Citation2012), B. sphaericus (Surendran, Vennison, Ravikumar, & Syed Ali, Citation2011), B. licheniformis (Banerjee, Ray, Askarian, & Ringo, Citation2013), B. subtilis RSKK96 (Pant et al., Citation2015), B. cohnii APT5 (Tekin et al., Citation2012), B. stearothermophilus Ap-4 (Qudar, Shireen, Iqbal, & Tuzun, Citation2009), B. firmus (wishard et al., Citation2014), etc. In general, extracellular proteases have advantages over intracellular one in term of cost and availability. The production rate of bacterial enzymes depends on several culture parameters like temperature, pH, vitamins, moisture, inoculums size, carbon, and nitrogen sources (Roy, Banerjee, Dan, & Ray, Citation2013) and might be enhanced through optimization. In this present review, we have summarized the sources of bacterial and fungal proteases, their purification strategies, pH stability, temperature optima, and their application in several industries.
Sources of microbial proteases
Microbes live in such an environment where the nutrient available is in macromolecular form (protein, lipid, and carbohydrate), and thus need to convert it into smaller ones. The breakdown of such macromolecules is completed by the action of different types of enzymes (Conlon & Bird, Citation2015). Proteases are the most important class of enzymes that convert complex protein into smaller peptides or amino acids. Proteases produced by microbiota differ greatly in structure and function, and can be divided into several classes such as, serine protease, cysteine protease, acid protease, and metallo protease (Salevesen & Nagase, Citation1983; Kalisz, Citation2006; Bhunia et al., Citation2012). Serine protease are characterized by the presence of a serine residue at active site and are reported to be most common form of bacterial protease (Salevesen & Nagase, Citation1983; Hershey et al., Citation2016). In a recent investigation, Zhang et al. (Citation2015) have demonstrated the diversity of serine protease producing bacteria in the sediment of the eutrophied Jiaozhou Bay, China. Results of their study reported that the dominant bacterial species belongs to the genus Pseudoalteromonas, Psychrobacter Photobacterium, Vibrio, Halobacillus, Bacillus, Microbulbifer, and Shewanella. Till date, a wide range of bacterial and fungal species have been identified as an active producer of serine proteases (Table ). Generally, serine proteases are alkaline in nature and optimally active at pH 7.0–11.0 (Khan, Ahmad, Zafar, Nasir, & Qadir, Citation2011; Padmapriya, Thamaraichelvan, Nandita, & Raj, Citation2012; Mothe & Sultanpuram, Citation2016). Whereas, cysteine proteases contains cysteine residue at their active site and are mostly produced by fungal species like Aspergillus oryzazae and Sporotrichum pulverulentum (Kalisz, Citation2006; Souza et al., Citation2015). On the other hand, metallo proteases are another class of enzymes that requires a metal ion (usually zinc, cobalt, nickel and manganese) as cofactor. Several types of micro-organisms (B. subtilis, B. cereus, B. megaterium, Streptomyces griseus, Eupenicillium javanicum, Microsporum canis) have been identified as good producer of metallo enzymes (Brouta et al., Citation2001; Saxena & Singh, Citation2011; Neto, Motta, & Cabral, Citation2013). In this present review, we have focused most of our priority to serine proteases, as it has high demand in industries.
Table 1. Serine proteases producing microbial isolates.
Proteases production/secretion by bacterial isolates is a common phenomenon and has been investigated extensively (Table ). There are several sources of bacterial proteases; however, Bacillus strains have commercial recognition in most of the cases (Gupta et al., Citation2002; Pant et al., Citation2015; Tavea, Fossi, Fabrice, Ngoune, & Ndjouenkeu, Citation2016). Due to high demand, the isolation and characterization of protease producing candidates is an important research area and several projects are going on in that particular direction. In a detail investigation, Padmapriya et al. (Citation2012) have characterized an alkaline protease produced by the bacterium Bacillus sp. isolated from Perna viridis. Similarly, Chu, Lee, and Shu-Tsu (Citation1992) and (Boyer & Byng, Citation1996) have also reported the production of alkaline protease from bacteria like Bacillus amyloliquefaciens, Bacillus proreolyticus, Bacillus subtilis. Not only bacteria, a wide range of fungi, such as Aspergillus flavus, Fusarium graminarum, Penicillium griseofulvin, Aspergillus niger, Chrysosporium keratinophilum, Scedosporium apiosermum and Aspergillus melleu were also considered to be good sources of serine proteases (Ellaiah, Srinivasulu, & Adinarayana, Citation2002; Sharma & De, Citation2011; Novelli, Barros, & Fleuri, Citation2016). In a study conducted by Kalpana Devi, Rasheedha Banu, Gnanaprabhal, Pradeep, and Palaniswamy (Citation2008) has characterized the protease producing fungi, A. niger isolated from soil sample. Similarly, Charles et al. (Citation2008) also have reported the proteolytic fungus Aspergillus nidulans HA-10 isolated from poultry farm. On the other hand, Anand (Citation2016) has isolated and characterized several strains of A. flavus from soil and optimized their protease producing capacity through solid state fermentation. Whereas, Penicillium griseofulvum and Scedosporium apiospermum were also recognized to be good producer of proteases (Dixit & Verma, Citation1993; Larcher et al., Citation1996; Jisha et al., Citation2013; Souza et al., Citation2015). We are surrounded by a microbial world and very little information has been documented about their diversity and community, even most of the bacterial isolates (culturable and un-culturable) have not identified till date. The water, soil and gastrointestinal tract (GI) of animal are considered to be the richest sources of several bacterial and fungal candidates (Banerjee et al., Citation2016). Choudhary and Jain (Citation2012) have investigated the diversity of proteolytic fungus at different habitats and reported that Aspergillus sp. and Fusarium sp. were the most predominant genera. Researchers have reported the protease producing candidates in the GI tract of several animals, which play a crucial role in host nutrition and defense (Ray et al., Citation2012; Banerjee et al., Citation2013 Banerjee et al., Citation2016). Fungal sources of proteases also have high industrial demand due to stability, broad diversity, and substrate specificity. Compared to bacterial cells, separation of mycelium is easy and thus it is cost-effective. The available important sources of fungal proteases were given in Table .
Table 2. Important sources of bacterial proteases.
Table 3. Proteases production by fungal isolates.
Proteases engineering
Proteases engineering is an important step that lead to the development of novel tool for therapeutic and biotechnological applications. In general, the activity and stability of any enzymes including proteases depend on the amino acid composition and their respective position. Protein engineering modifies the protease structure in such a way which enhances the activity, specificity and stability of proteases (Pogson et al., Citation2009). Engineering of proteases for diverse industrial purposes has been extensively investigated (Table ). Currently, two different methods are used for making engineered proteases: directed evolution and random design (Böttcher & Bornscheuer, Citation2010). Thermal stability of protease is very important, which can been increased using different engineering strategies such as disulfide bond formation, substrate cite amino acid modification, modification of metal binding site, and comparing sequence homology with other enzymes produced by mesophiles and thermophiles (Li, Yi, Marek, & Iverson, Citation2013). Similarly, pH stability (surface charge engineering) and activity in organic solvent environment (disulfide bond engineering) of proteases have also been reported (Takagi, Hirai, Maeda, Matsuzawa, & Nakamori, Citation2000; Li et al., Citation2013; Vojcic et al., Citation2015). Cummings, Murata, Koepsel, and Russell (Citation2014) have used dual block polymer-based protein engineering to enhance the pH and thermal stability of protease. The need of biocatalyst varies greatly (in term of stability and activity at different temperature and pH) from one industry to another, and protein engineering is the way to make it possible.
Table 4. Engineered proteases for industrial use.
Purification strategies of microbial protease
Biotechnological industries produce different types of microbial enzymes in bulk amount and do not require high purity. However, enzymes used in pharmaceutical, medical and research purpose require high purity. Several types of classical methods have been employed for enzyme purification from microbial sources. However, the general method of enzyme purification was given in Figure . Enzyme purification is not a single step procedure, it require combination of several technologies and methods. Purification details of proteases from several microbial sources are given in Table . Few important techniques that are generally used for microbial enzyme purification are described below.
Figure 1. Diagram demonstrates the general procedure of microbial protease and amylase purification.
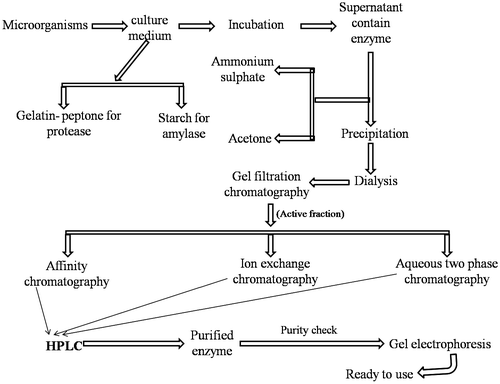
Table 5. Purification strategies of microbial proteases.
Gel filtration chromatography
It is very common method of enzyme purification. Several types of gels or beads have been used for column packing such as Sephadex (G-25, G-50, G-75, G-100, G-200), Sepharose, Sephacryl, etc. The selection of packing material depends on molecular weight of the enzyme. Such as, lower molecular weight enzymes (10–80 kDa) require Sephadex G-25 to G-75. Whereas, enzymes having higher mass require G-100 to G-300. Packing of column is very important for proper purification. Bubble in the column hampers the flow rate and thus before packing, gel should be properly de-gassed. Readymade columns are also available from different company such as, Sigma Pvt. Ltd, Merck Pvt. Ltd, HiMedia Pvt. Ltd, Bio-RAD Pvt. Ltd, etc.
Affinity chromatography
It is a very popular method for enzyme and protein purification. Several types of packing material are used depending on the nature of molecules. The most common matrices for bacterial neutral enzymes are Sephadex-4-phenylbutylamine. The others available matrices are Casein agarose, N-benzoyloxycarbonyl, lectin, etc. The major drawbacks of the affinity chromatography are high purification cost and labile nature of some ligands.
Ion-exchange chromatography
In ion-exchange chromatography, separation or purification of enzymes depends on ionic nature (positive or negative). Generally two types of beads are used, DEAE-sephades/cellulose (anion) and CM-Sephadex/cellulose (Cation). Mostly microbial enzymes are positively charged and require anion exchanger. Elution of bounded molecules is done by increasing salt concentration (usually NaCl and CaCl2) or pH gradient.
Aqueous two-phase system chromatography
Microbial alkaline protease can be purified using a combination of two mixtures such as, polyethylene glycol (PEG) and dextran or PEG and different types of salts. This method has several advantages: provides mild condition for biological macromolecule, higher concentration, less time taking, and cost-effective.
High-pressure and low-pressure liquid chromatography
High-pressure liquid chromatography (HPLC) and low-pressure liquid chromatography (LPLC) are sophisticated technique for protein purification. HPLC differ from LPLC in term of pressure. Both of these techniques are useful for molecular grade purification of protein. Depending on the purpose, HPLC might be divided into three categories: analytical, semi-preparative, and preparative.
Fast protein liquid chromatography
It is one of the most popular chromatography instrument, usually used to purify the proteins and enzymes. Like liquid chromatography, it also contains a liquid mobile phase or buffer (phosphate buffer and citrate buffer) and a stationary phase which contains resin. The flow rate of buffer is controlled by a positive-displacement pump. Selection of column is important in Fast protein liquid chromatography (FPLC) and may be varied (size exclusion, hydrophobicity, ion exchange, reverse phase, etc.) according to the nature of separating molecules.
Temperature and pH optima of microbial protease
The production rate of microbial enzymes are highly dependent on various culture parameters like temperature, pH, moisture content, inoculums size, duration, agitation speed, carbons, and nitrogen sources. The optimization of these factors are important in response to product enhancement, cost-effectiveness and time. Among several parameters, temperature and pH are considered to be most crucial for maximum enzyme activity. Proteases produced by bacteria and fungi vary greatly in structure, substrate specificity, molecular weight, and physical characteristics. The optimum temperature for proteases produced by different Bacillus species has been reported by several researchers such as, 50 °C for Bacillus licheniformis (Sareen & Mishra, Citation2008), 45 °C for Bacillus thermoruber (Manachini, Fortina, & Parini, Citation1998), 70 °C for marine Bacillus sp. (Padmapriya et al., Citation2012) and 70 °C for B. subtilis 50a (Azlina & Norazila, Citation2013). Oseni (Citation2011) has isolated four fungal isolates (A. flavus, A. niger, Aspergillus fumigatus, and Penicillium italicum) and investigated their temperature optima, which lied between 30 and 60 °C.
Like temperature, the activity of microbial proteases also depend on pH of the reaction media such as pH 9.0 for B. subtilis 50a and B. licheniformis 50a (Oseni, Citation2011), pH 12.0 for Bacillus clausii (Joo, Kumar, Park, Paik, & Chang, Citation2003) and pH 7.0 for Pseudomonas fluorescens (Padmapriya et al., Citation2012). Oseni (Citation2011) also has stated that proteases produced by A. niger and P. italicum have acidic pH optima. While, proteases obtained from Aspergillus versicolor have exhibited optimum activity at pH 9.0 (Choudhary & Jain, Citation2012). The sources of protease and their temperature and pH optima were presented in Table .
Table 6. Temperature and pH optima of proteases produced by the selected micro-organisms.
Application of protease in industrial sectors
Microbial enzymes, especially proteases have several important roles in industrial sectors such as paper mill, leather processing, liquid detergent preparation, food processing, and medical sector. Among different classes, alkaline and heat stable serine proteases are considered to be most important. The contribution of proteases in different industrial sectors is highlighted below.
Leather processing industries
Leather industries consume a significant amount of protease for raw leather processing, which consists of several steps like soaking, liming, dehairing, deliming, bating, degreasing, and pickling. Alkaline proteases produced by micro-organism are very useful for removing hair and this is quite safer than the traditional method that used hazardous chemicals like, sodium sulfide, which creates environmental pollution (Ellaiah et al., Citation2002; Sawant & Nagendran, Citation2014; Souza et al., Citation2015). Alkaline proteases hydrolyze non-collagenous constituent of skin and also remove non-fibrillar proteins such as globulin and albumin that are needed for leather processing (Jridi et al., Citation2014). Jisha et al. (Citation2013) have reported the use of some industrially important proteases producing microbes such as Streptomyces sp., A. flavus, Bacillus amyloliquefaciens and Bacillus subtilis. Ellaiah et al. (Citation2002) have stated that tanning of raw leather should be done in high alkaline condition (pH 12.0) and for that purpose alkaline proteases produced by micro-organism is a better choice. However, genetically modified strains have higher priority in industries and are used randomly for getting better results (Khan, Citation2013; Adrioo & Demain, Citation2014). For example, addition of pBX96 plasmid in Bacillus pumilus c172–14 (Ng, Citation2001), site directed mutagenesis in Bacillus amyloliquefaciens (Estell, Graycar, & Wells, Citation1985; Xu, Dai et al. Citation2015) and modification of enzyme producing gene in B. licheniformis (Fleming, Tangney, Jorgensen, Diderichsen, & Priest, Citation1995; Zhang, Duan, & Wu, Citation2016) gives better performance in leather industries.
Waste management
Microbial sources of keratinases are very useful in waste management due to its broad substrate specificity. In general, keratinases convert the feather or other waste materials (such as scale, hair, wool, etc.) into karatin, which has high demand in poultry and aquaculture industries as feed ingredient (Deivasigamani & Alagappan, Citation2008; Motyan, Toth, & Tozser, Citation2013). In a review, Gopinath et al. (Citation2015) have explored the microbial sources of keratinases and their function in different industrial sectors. Karatin is an insoluble protein; however, the conversion of insoluble karatin to soluble from is possible through submerged fermentation using keratinases produced microbes (Suntornsuk & Suntornsuk, Citation2003). Due to special characteristics, keratinases have been widely used as nematicidal agents, biofuel production, and fertilizer production from poultry waste (Verma et al., Citation2017). Dalev (Citation1994) has reported an alkaline keratinase from B. subtilis which might be useful in processing of feather in poultry industries. In a similar study, Verma, Singh, Anwar, Ansari, and Agrawal (Citation2014) have also reported the feather degrading alkaline keratinase from Thermoactinomyces sp. RS1, which can be used in feed preparation in poultry farm. Other keratin degrading protease were also reported for various function, such for peptide production (Motyan et al., Citation2013), recycling waste (Verma et al., Citation2016), food processing industries (Vanitha, Rajan, & Murugesan, Citation2014) and waste water treatment (Jisha et al., Citation2013).
Food industries
In recent year, microbial proteases have been randomly used in food industries like meat tenderization and cheese preparation (Shi, Coyne, & Weiner, Citation1997; Adrioo & Demain, Citation2014). Milk protein coagulation is the first step in cheese preparation, which is accomplished with the help of protease enzyme. Cheese making industries uses microbial source of proteases; however, it has two drawbacks: low yield and bitterness upon long storage. Among several classes of proteases, aspartic proteases are considered to be best for cheese industries (Claverie-MartÌn & Vega-Hernàndez, Citation2007; Feijoo-Siota et al., Citation2014). Microbes produce several types of proteases which are non-specific and thus cause bitterness in storage food. Nissen (Citation1986) has reported that Alcalase (an alkaline protease) remove bitterness from food stuff and make it sweeter. Whereas, protease produced by B. amyloliquefaciens is useful for making methionine-rich protein hydrolysate from chickpea protein (George, Sivasankar, Jayaraman, & Vijayalakshmi, Citation1997; Wang et al., Citation2016). Proteases obtained from microbial sources are used for the fortification of fruit juices or soft drinks and in preparation of protein rich therapeutic diets also (Adamson & Reynolds, Citation1996; Amore & Faraco, Citation2015). Wheat flour is the major component of baking process which contains an insoluble protein, gluten that determines the bakery dough’s properties. Protease produced by Aspergillus oryzae hydrolyzes the gluten and increases the product quality in terms of color, softness, and texture (Sawant & Nagendran, Citation2014; Souza et al., Citation2015).
Silver recovery
Silver is a valuable metal and major cost component in the film, used in photography, MRI, CT scan, and X ray. X-ray film content approximately 1.5 to 2% silver in its gelatin layer. Recovery of silver from used X-ray film is quite difficult and laborious. There are several chemical methods for silver recovery such as burning of film and oxidation of metallic silver, but these are not environment friendly. Alkaline proteases are used for this particular purpose, which actively degrade the gelatin layer of the film and release the bounded silver (Kumaran, Mahalakshmipriya, & Rajan, Citation2013). Shankar, More, and Seeta Laxman (Citation2010) have isolated and characterized an alkaline protease from Conidiobolus coronatus and reported its silver recovering efficiency. They also have stated that silver hydrolysis by this protease was completed at pH 10.0 within 6 min. While, Singh, Vohra, and Sahoo (Citation1999) have investigated the silver recovering efficiency of protease obtained from Bacillus sphaericus, which was able to hydrolyze the silver at pH 11.0–12.0 within 8 min. Bacillus coagulans and Bacillus sp. 21-2 were also reported to be important bacterial strains in silver recovering industries (Fuziwara & Yamamoto, Citation1987; Gajju, Bhalla, & Agarwal, Citation1996; Jisha et al., Citation2013; Vanitha et al., Citation2014). Similarly, Anju, Kondari, and Sarada (Citation2014) also have reported the efficiency of silver recovery from X-ray and MRI plate by protease producing Bacillus sp. isolated from soil sample. Due to availability, eco-friendly nature, and low cost microbial sources of proteases have great demand in silver recovery industries.
Detergent industries
Different types of enzymes like protease, amylase, lipase, etc. are used in detergent industries. Among these, proteases account for approximately 60.0% of total enzyme sales in detergent industries (Ibrahim et al., Citation2016). In 1913, Otto Ro¨hm discovered that pancreas extract was useful to degrade protein and can be used for washing clothes. Later on, in 1956 bacterial enzyme containing detergent was introduced in market under the trade name Bio-40. The domestic detergent contains different types of toxic chemicals such as polycarboxylates, chlorine, phosphonates, etc. which are not good for environment and can cause skin diseases. The performance of enzyme in detergent depends on various factors such as, washing temperature, type of stain, water hardness, and washing procedure (Hasan, Ali Shah, Javed, & Hameed, Citation2010; Li, Yang, Yang, Zhu, & Wang, Citation2012). The ideal protease that can be used in detergent industries should have two characteristics; stable at alkaline pH and compatible with detergent (Niyonzima & More, Citation2015). Proteases obtained from Bacillus sp. are very useful in manufacturing laundry detergent such as Era-plus (Procter & Gamble), Dynamo and Tide (Colgate Palmotive). In recent year, protease produced from two potential bacterial strains, B. clausii KSM-k16 and Bacillus sp. KSM-KP43 have been incorporated in laundry industries (Hasan et al., Citation2010; Bialkowska et al., Citation2016). Demand of detergent-compatible protease is high and thus, the screening of protease producing bacteria is a continuous process. From environmental perspective, use of microbial proteases in manufacturing detergent is good for health and nature.
Chemical industries
Proteases play a major role in synthetic chemical industry in form of biocatalyst. However, a major drawback of this application is reduction of enzyme activity under anhydrous condition. Several researchers have reported the catalysis of peptide synthesis in organic solvent by alkaline proteases (Motyan et al., Citation2013; Anbu, Citation2016). Patil, Rethwisch, and Dordick (Citation1991) reported a novel alkaline protease (proleather) produced by Bacillus sp. which is very useful in case of sucrose polymer synthesis under anhydrous condition and transesterification of D-glucose into pyridine. On the other hand, alkaline proteases from C. coronatus has potential role in replacing subtilisin from various reaction mixture (Sutar, Srinivasan, & Vartak, Citation1991; Bhunia et al., Citation2012; Bialkowska et al., Citation2016).
Medical purpose
The applications of protease in medical sectors are accepted worldwide. Proteases from microbial sources were detected to be effective in treatment of various diseases like tumor, inflammation, cancer, etc. A. oryzae is an important fungal isolate which produces a novel protease that is useful in treatment of certain enzyme deficiency syndromes (Palanivel, Ashokkumar, & Banagurunathan, Citation2013; Gurung, Ray, Bose, & Rai, Citation2013). In last few years, the concept of protease action has been changed dramatically. Apart from the catalytic activity, proteases are considered to be important agent in several biochemical pathways and physiological functions such as, cell signaling, apoptosis, cell migration, cell adhesion, and metastasis. Researchers have reported that inactivation of such proteases using microbial protease inhibitor might be an alternative way in developing anti cancer drug (Pervaiz, Hirpara, & Clement, Citation1998; Rakashanda & Amin, Citation2013; Petra & Monika, Citation2016). From toxicity point of view, use of enzyme in cancer treatment has advantage over chemotherapeutic agents. Sawant and Nagendran (Citation2014) have reported that Clostridial collagenase or subtilisin has been randomly used in preparation of several antibiotics, whereas, aspargine removing protease, asparginase, produced by E. coli is effective in treatment of lymphocytic leukemia (Kishore, Nishita, & Manonmani, Citation2015). Blood clotting in vascular pipeline block blood supply and it is a major problem that causes cerebral and cardiac attack. Researchers have reported that fibrinolytic activity exhibited by several bacterial and fungal species are important in cardiovascular treatment (Table ). The molecules which dissolve these clots are some kind of proteases called thrombolytic agent. In last few years, several thrombolytic agents from microbial sources like streptokinase (SK) and staphylokinase (SAK) were developed to overcome this critical situation (Srilakshmi, Madhavi, Lavanya, & Ammani, Citation2014; Mane & Tale, Citation2015). Protease also plays a critical role as an anti-inflammatory agent. The bacterium Serratia sp. produced a special protease called serratiopepetidase, which is able to reduce inflammation rapidly. In a review, Patyar et al. (Citation2010) have stated that genetically engineered bacteria are very much effective in different types of cancer therapy, including targeted cell signaling inhibition by bacterial protease such as streptokinase production from Streptococcoi through recombinant DNA technology.
Table 7. Fibrinolytic activity exhibited by micro-organisms.
Other uses
In recent year, proteases from biological source have been used randomly for research purpose also, such as alkaline protease produced by C. coronatus is the substitute of trypsin in animal cell culture (Sawant & Nagendran, Citation2014).
Future scope
Proteases are unique classes of enzymes that perform different role in industries, as well as maintain physiological condition. Till date, researchers have investigated and reported the production of protease by several micro-organisms. In present day, a wide variety of molecular biological and biotechnological methods have been introduced for enhancing the microbial enzyme production through genetic modifications and protein engineering. Cloning, addition of plasmid, site-directed mutagenesis and deletion of repressor gene are modern techniques that are used to manipulate the gene and control their expression. Along with gene editing, protein engineering is also very important and has huge impact in improving proteases properties. The goal of the protein engineering is to change the structure of the enzyme to maximize the industrial production. A huge number of micro-organisms have been reported as protease producer, but few have been accepted as commercial application. The combination of DNA recombinant technology, protein engineering, and gene cloning can overcome this situation. Nowadays more than 50% of industrially important enzymes are produced by genetically modified strains or engineered protein. Thus, targeted modification of such enzyme producing gene and alteration of amino acid position might be effective for better production.
Conclusion
Due to huge industrialization, demand of enzymes increase rapidly. In this present review, we have summarized the importance of protease in different sectors. Production of enzyme from different group such as animal, plant, and microbes were already established, but people are interested in microbial sources of enzymes due to several reasons such as, higher production through fermentation, availability, diversity, stability, and lower cost. Bacteria and fungi were reported to be good sources of different types of enzymes like protease, amylase, lipase, cellulase, pectinase, chitinase, phytase, β-galactosidase, etc. In our review, we have included only protease due to greater importance. Microbes produce different types of proteases but alkaline serine protease has much more importance. Among various bacterial strains, Bacillus species were reported to be most important in enzyme production. Similarly, the fungal isolates belongs to the genus Aspergillus were industrially valuable. Day by day, the use of enzyme in industries will increase, and thus to fulfill the demand, screening of new enzyme producing microbial isolate is important.
Disclosure statement
No potential conflict of interest was reported by the authors.
References
- Adamson, N. J., & Reynolds, E. C. (1996). Characterization of casein phosphopeptides prepared using alcalase: Determination of enzyme specificity. Enzyme and Microbial Technology, 19, 202–207.10.1016/0141-0229(95)00232-4
- Adrioo, J. L., & Demain, A. L. (2014). Microbial enzymes: Tools for biotechnological processes. Biomolecules, 4(1), 117–139.10.3390/biom4010117
- Afifah, D. N., Sulchan, M., Syah, D., Yanti, Suhartono, M. T., & Kim, J. H. (2014). Purification and characterization of a fibrinolytic enzyme from Bacillus pumilus2.g isolated from Gembus, an Indonesian fermented food. Preventive Nutrition and Food Science, 19(3), 213–219.10.3746/pnf.2014.19.3.213
- Ahmad, S., Noor, Z. M., & Ariffin, Z. Z. (2014). Isolation and identification fibrinolytic protease endophytic fungi from Hibiscus leaves in Shah Alam. International Scholarly and Scientific Research & Innovation, 8(10), 1104–1107.
- Al-Askar, A. A., Abdulkhair, W. M., & Rashad, Y. (2014). Production, purification and optimization of protease by Fusarium solani under solid state sermentation and isolation of protease inhibitor protein from Rumex vesicarius L. Journal of Pure and Applied Microbiology, 8(1), 239–250.
- Amore, A., & Faraco, V. (2015). Enzymes for food and beverage industries: Current situation, challenge and perspectives. In V. Ravishankar Rai (Ed.), Advances in food biotechnology (pp. 165–177). Wiley.10.1002/9781118864463
- An, L., Ding, A. Y., Chen, J., Liu, S. A., Zhang, M., & Li, D. C. (2007). Purification and characterization of two thermostable proteases from the thermophilic fungus Chaetomium thermophilum. Journal of Microbiology and Biotechnology, 17(4), 624–631.
- Anand, K. (2016). Fungal protease production by Aspergillus niger and Aspergillus flavus using rice bran as the substrate. Academic Journal of Agricultural Research, 4(6), 333–338.
- Anbu, P. (2016). Enhanced production and organic solvent stability of a protease from Brevibacillus laterosporus strain PAP04. Brazilian Journal of Medical and Biological Research, 49. doi:10.1590/1414-431X20165178
- Anju, S., Kondari, S. R., & Sarada, J. (2014). Bioprocess of silver extraction from used X-ray and MRI. Acta Biologica Indica, 3(1), 561–568.
- Ariole, C. N., & Ilega, E. (2013). Alkaline protease produced by Pseudomonas aeruginosa isolated from the gut of Pila ovate. Journal of Glob Biosciences, 2(5), 126–131.
- Asker, M. M. S., Mahmoud, M. G., El Shebwy, K., & Abd el Aziz, M. S. (2013). Purification and characterization of two thermostable protease fractions from Bacillus megaterium. Journal of Genetic Engineering and Biotechnology, 11(2), 103–109.10.1016/j.jgeb.2013.08.001
- Azlina, I. N., & Norazila, Y. (2013). Thermostable alkaline serine protease from thermophilic Bacillus Species. International Research Journal of Biological Sciences, 2(2), 29–33.
- Banerjee, G., Ray, A. K., Askarian, F., & Ringo, E. (2013). Characterization and identification of enzyme-producing autochthonous bacteria from the gastrointestinal tract of two Indian airbreathing fish. Beneficial Microbes, 4, 277–284.10.3920/BM2012.0051
- Banerjee, G., Mukherjee, S., Bhattacharya, S., & Ray, A. K. (2016). Purification and characterization of extracellular protease and amylase produced by the bacterial strain, Corynebacterium alkanolyticum ATH3 isolated from fish gut. Arabian Journal for Science and Engineering, 41, 9–16.10.1007/s13369-015-1809-4
- Beg, Q. K., & Gupta, R. (2003). Purification and characterization of an oxidation-stable, thiol-dependent serine alkaline protease from Bacillus mojavensis. Enzyme and Microbial Technology, 32(2), 294–304.10.1016/S0141-0229(02)00293-4
- Benito, M. J., Rodríguez, M., Núñez, F., Asensio, M. A., Bermúdez, M. E., & Córdoba, J. J. (2002). Purification and characterization of an extracellular protease from Penicillium chrysogenum Pg222 active against peat Proteins. Applied and Environmental Microbiology, 68(7), 3532–3536.10.1128/AEM.68.7.3532-3536.2002
- Bhunia, B., Basak, B., & Dey, A. (2012). A review on production of serine alkaline protease by Bacillus spp. Journal of Biochemical Technology, 3(4), 448–457.
- Bialkowska, A., Gromek, E., Florczak, T., Krysiak, J., Szulczewska, K., & Turkiewicz, M. (2016). Extremophilic proteases: Developments of their special function, potential resources and biotechnological applications. In P. H. Rampelotto (Ed.), Grand challenges in biology and biotechnology (pp. 399–444). Springer International.
- Böttcher, D., & Bornscheuer, U. T. (2010). Protein engineering of microbial enzymes. Current Opinion in Microbiology, 13, 274–282.10.1016/j.mib.2010.01.010
- Boyer, E. W., & Byng, G. S. (1996). Bacillus proteolyticus species which produce an alkaline protease. U.S. Patent No. 551891.
- Brouta, F., Descamps, F., Fett, T., Losson, B., Gerday, C. H., & Mignon, B. (2001). Purification and characterization of a 43.5 kDa keratinolytic metalloprotease from Microsporum canis. Medical Mycology, 39, 269–275.10.1080/mmy.39.3.269.275
- Chakrabarti, S. K., Matsumura, N., & Ranu, R. S. (2000). Purification and characterization of an extracellular alkaline serine protease from Aspergillus terreus (IJIRA 6.2). Current Microbiology, 40(4), 239–244.10.1007/s002849910048
- Chandrasekaran, M., & Sathiyabama, M. (2014). Production, partial purification and characterization of protease from a phytopathogenic fungi Alternaria solani (Ell. and Mart.) Sorauer. Journal of Basic Microbiology, 54, 763–774.10.1002/jobm.v54.8
- Charles, P., Devanathan, V., Anbu, P., Ponnuswamy, M. N., Kalaichelvan, P. T., & Hur, B. K. (2008). Purification, characterization and crystallization of an extracellular alkaline protease from Aspergillus nidulans HA-10. Journal of Basic Microbiology, 48(5), 347–352.10.1002/jobm.v48:5
- Chen, W. M., Huang, H. W., Chang, J. S., Han, Y. L., Guo, T. R., & Sheu, S. Y. (2013). Tepidimonas fonticaldi sp. nov., a slightly thermophilic betaproteobacterium isolated from a hot spring. International Journal of Systematic and Evolutionary Microbiology, 63, 1810–1816.10.1099/ijs.0.043729-0
- Choudhary, V., & Jain, P. C. (2012). Isolation and identification of alkaline protease producing fungi from soils of different habitats of Sagar and Jabalpur District (M.P). Journal of Academia and Industrial Research, 1(3), 106–113.
- Chu, I. M., Lee, C., & Shu-Tsu, L. (1992). Production and degradation of alkaline protease in batch cultures of Bacillus subtilis ATCC 14416. Enzyme and Microbial Technology, 14(9), 755–761.10.1016/0141-0229(92)90116-6
- Claverie-MartÌn, F., & Vega-Hernàndez, M. C. (2007). Aspartic proteases used in cheese making. In J. Polaina & A. P. MacCabe (Eds.), Industrial Enzymes (pp. 207–219). Netherlands: Springer.
- Conlon, M. A., & Bird, A. R. (2015). The impact of diet and lifestyle on gut microbiota and human health. Nutrients, 7, 17–44.
- Cummings, C., Murata, H., Koepsel, R., & Russell, A. J. (2014). Dramatically Increased pH and temperature stability of chymotrypsin using dual block polymer-based protein engineering. Biomacromolecules, 15(3), 763–771.10.1021/bm401575 k
- Dalev, P. G. (1994). Utilization of waste feathers from poultry slaughter for production of a protein concentrate. Bioresources Technology, 48, 265–267.10.1016/0960-8524(94)90156-2
- De Castro, R. J. S., Ohara, A., Nishide, T. G., Albernaz, J. R. M., Soares, M. H., & Sato, H. H. (2015). A new approach for proteases production by Aspergillus niger based on the kinetic and thermodynamic parameters of the enzymes obtained. Biocatalysis and Agricultural Biotechnology, 4(2), 199–207.10.1016/j.bcab.2014.12.001
- De Castro, R. J. S., & Sato, H. H. (2014). Protease from Aspergillus oryzae: Biochemical characterization and application as a potential biocatalyst for production of protein hydrolysates with antioxidant activities. Journal of Food Processing. ID 372352. doi:10.1155/2014/372352
- Deivasigamani, B., & Alagappan, K. M. (2008). Industrial application of keratinase and soluble proteins from feather keratins. Journal of Environmental Biology, 29(6), 933–936.
- Dian Siti, S., Ihsanawati, & Hertadi, R. (2015). Isolation and characterization of organic-solvent stable protease isolated by Pseudomonas stutzeri BK AB-12. Procedia Chemistry, 16(2015), 341–348.10.1016/j.proche.2015.12.062
- Dixit, G., & Verma, S. C. (1993). Production of alkaline proteases by Penicillium griseofulvin. Indian Journal of Microbiology, 33, 257–260.
- Eijsink, V. G. H., vander Zee, J. K., vanden Burg, B., Vriend, G., & Venema, G. (1991). Improving the thermostability of the neutral protease of Bacillus stearothermophilus by replacing a burried aspargine by leucine. FEBS Letters, 282, 13–16.10.1016/0014-5793(91)80434-5
- Eijsink, V. G. H., Vriend, G., vanden Burg, B., vander Zee, J. R., & Venema, G. (1992). Increasing the thermostability of a neutral protease by replacing positively charged amino acids in the Nterminal turn of α-helices. Protein Engineering, 5, 165–170.10.1093/protein/5.2.165
- Ellaiah, P., Srinivasulu, B., & Adinarayana, K. (2002). A review on microbial alkaline proteases. Journal of Scientific & Industrial Research, 61, 690–704.
- Escobar, J., & Barnett, S. M. (1993). Effect of agitation speed on the synthesis of Mucor miehei acid protease. Enzyme and Microbial Technology, 15(12), 1009–1013.10.1016/0141-0229(93)90047-6
- Estell, D. A., Graycar, T. P., & Wells, J. A. (1985). Engineering an enzyme by site-directed mutagenesis to be resistant to chemical oxidation. Journal of Biological Chemistry, 260(1985), 6518–6521.
- Feijoo-Siota, L., Blasco, L., Rodríguez-Rama, J. L., Barros-Velázquez, J., Miguel, T. d., Sánchez-Pérez, A., & Villa, T. G. (2014). Recent patents on microbial proteases for the dairy industry. Recent Advances in DNA and Gene Sequences, 8(1), 44–55.
- Fleming, A. B., Tangney, M., Jorgensen, P. L., Diderichsen, B., & Priest, F. G. (1995). Extracellular enzyme synthesis in a sporulation-deficient strain of Bacillus licheniformis. Applied and Environmental Microbiology, 61(1995), 3775–3780.
- Fuziwara, N., & Yamamoto, K. (1987). Decomposition of gelatin layers on X-ray films by the alkaline protease from Bacillus sp. Hakkokogaku, 65, 531–534.
- Gajju, H., Bhalla, T. C., & Agarwal, H. O. (1996). Proc 37th Annu Conf Assoc of Microbial India Chennai.
- Gandhi, A., & Shah, N. P. (2014). Cell growth and proteolytic activity of Lactobacillus acidophilus, Lactobacillus helveticus, Lactobacillus delbrueckii ssp. bulgaricus, and Streptococcus thermophilus in milk as affected by supplementation with peptide fractions. International Journal of Food Sciences and Nutrition, 65(8), 937–941.10.3109/09637486.2014.945154
- Gaur, S., Agrahari, S., & Wadhwa, N. (2010). Purification of protease from Pseudomonas thermaerum GW1 isolated from poultry waste site. Open Microbiology, Journal, 4(1), 67–74.10.2174/1874285801004010067
- Geng, C., Nie, X., Tang, Z., Zhang, Y., Lin, J., Sun, M., & Peng, D. (2016). A novel serine protease, Sep1, from Bacillus firmus DS-1 has nematicidal activity and degrades multiple intestinal-associated nematode proteins. Scientific Reports, 6, 25012.10.1038/srep25012
- George, S., Sivasankar, B., Jayaraman, K., & Vijayalakshmi, M. A. (1997). Production and separation of methionine rich fraction from chick pea protein hydrolysate generated by proteases of Bacillus amyloliquefaciens. Process Biochemistry, 32(5), 401–404.10.1016/S0032-9592(96)00082-9
- Giraud, E., Gosselin, L., Marin, B., Parada, J. L., & Raimbault, M. (1993). Purification and characterization of an extracellular amylase from Lactobacillus plantarum strain A6. Journal of Applied Bacteriology, 75(3), 276–282.10.1111/jam.1993.75.issue-3
- Gopinath, S. C. B., Anbu, P., Lakshmipriya, T., Tang, T. H., Chen, Y., Hashim, U., … Md. Arshad, M. K. (2015). Biotechnological aspects and perspective of microbial keratinase production. BioMed Research International, 2015(2015), 140726.
- Graham, L. D., Haggett, K. D., Jennings, P. A., Le Brocque, D. S., Whittaker, R. G., & Schober, P. A. (1993). Random mutagenesis of the substrate binding site of a serine protease can generate enzymes with increased activities and altered primary specificities. Biochemistry, 32, 6250–6258.10.1021/bi00075a019
- Griffiths, M. W., & Tellez, A. M. (2013). Lactobacillus helveticus: The proteolytic system. Frontiers in Microbiology, 4, 30.
- Gupta, R., Beeg, Q. K., Khan, S., & Chauhan, B. (2002). An overview on fermentation, downstream processing and properties of microbial alkaline proteases. Applied Microbiology and Biotechnology, 60(4), 381–395.
- Gupta, R., Gigras, P., Mahapatra, H., Goswami, V. K., & Chauhan, B. (2003). Microbial α-amylases: A biotechnological perspective. Process Biochemistry, 38(11), 1599–1616.10.1016/S0032-9592(03)00053-0
- Gurung, N., Ray, S., Bose, S., & Rai, V. (2013). A broader view: Microbial enzymes and their relevance in industries, medicine, and beyond. BioMed Research International. Article ID 329121. doi:10.1155/2013/329121
- Hasan, F., Ali Shah, A., Javed, S., & Hameed, A. (2010). Enzymes used in detergents: Lipases. African Journal of Biotechnology, 9(31), 4836–4844.
- Hershey, D. M., Ren, X., Melnyk, R. A., Browne, P. J., Ozyamak, E., Jones, S. R., … Komeili, A. (2016). MamO is a repurposed serine protease that promotes magnetite biomineralization through direct transition metal binding in magnetotactic bacteria. PLOS Biology, 14(3), e1002402. doi:10.1371/journal.pbio.1002402
- Husain, Q. (2010). Beta galactosidases and their potential applications: A review. Critical Reviews in Biotechnology, 30(6), 41–62.10.3109/07388550903330497
- Hwang, K. J., Choi, K. H., Kim, M. J., Park, C. S., & Cha, J. (2007). Purification and characterization of a new fibrinolytic enzyme of Bacillus licheniformis KJ-31, isolated from Korean traditional Jeot-gal. Journal of Microbiology and Biotechnology, 17, 1469–1476.
- Ibrahim, A. S. S., Al-Salamah, A. A., El-Toni, A. M., Almaary, K. S., El-Tayeb, M. A., Elbadawi, Y. B., & Antranikian, G. (2016). Enhancement of alkaline protease activity and stability via covalent immobilization onto hollow core-mesoporous shell silica nanospheres. International Journal of Molecular Sciences, 17(2), 184.10.3390/ijms17020184
- Jellouli, K., Bougatef, A., Manni, L., Agrebi, R., Siala, R., Younes, I., & Nasri, M. (2009). Molecular and biochemical characterization of an extracellular serine-protease from Vibrio etschnikovii. Microbial Biotechnology, 36, 939–948.10.1007/s10295-009-0572-5
- Jemli, S., Ayadi-Zouari, D., Hlima, H. B., & Bejar, S. (2016). Biocatalysts: Application and engineering for industrial purposes. Critical Reviews in Biotechnology, 36(2), 246–258.10.3109/07388551.2014.950550
- Jisha, V. N., Smitha, R. B., Pradeep, S., Sreedevi, S., Unni, K. N., Sajith, S., … Benjamin, S. (2013). Versatility of microbial proteases. Advances in Enzyme Research, 1(3), 39–51.10.4236/aer.2013.13005
- Joo, H. S., Kumar, C. G., Park, G. C., Paik, S. R., & Chang, C. S. (2003). Oxidant and SDS-stable alkaline protease from Bacillus clausii I-52: Production and some properties. Journal of Applied Microbiology, 95(2), 267–272.10.1046/j.1365-2672.2003.01982.x
- Jridi, M., Lassoued, I., Nasri, R., Ayadi, M. A., Nasri, M., & NSouissi, N. (2014). Characterization and potential use of cuttlefish skin gelatin hydrolysates prepared by different microbial proteases. BioMed Research International. Article ID 461728. doi:10.1155/2014/461728
- Kalisz, H. M. (2006). Microbial proteinases. Advances in Biochemical Engineering Biotechnology, 36, 1–65.
- Kalpana Devi, M., Rasheedha Banu, A., Gnanaprabhal, G. R., Pradeep, B. V., & Palaniswamy, M. (2008). Purification, characterization of alkaline protease enzyme from native isolates Aspergillus niger and its compatibility with commercial detergents. Indian Journal of Science and Technology, 1(7), 1–6.
- Khalil, M. S. E. H., Allam, A. F. G., & Barakat, A. S. T. (2012). Nematicidal activity of some biopesticide agents and microorganism against root-knot nematode on tomato plant under greenhouse condition. Journal of Plant Protection Research, 52, 47–52.
- Khan, F. (2013). New microbial proteases in leather and detergent industries. Innovative Research in Chemistry, 1, 1–6.
- Khan, M. A., Ahmad, N., Zafar, A. U., Nasir, I. A., & Qadir, M. A. (2011). Isolation and screening of alkaline protease producing bacteria and physio-chemical characterization of the enzyme. African Journal of Biotechnology, 10(33), 6203–6212.
- Kim, T., & Lei, X. G. (2005). Expression and characterization of a thermostable serine protease (TfpA) from Thermomonospora fusca YX in Pichia pastoris. Applied Microbiology and Biotechnology, 68(3), 355–359.10.1007/s00253-005-1911-8
- Kishore, V., Nishita, K. P., & Manonmani, H. K. (2015). Cloning, expression and characterization of l-asparaginase from Pseudomonas fluorescens for large scale production in E. coli BL21. 3 Biotech, 5(6), 975–981.
- Kohlmann, K. L., Nielsen, S. S., Steenson, L. R., & Ladisch, M. R. (1991). Production of proteases by psychrotrophic microorganisms. Journal of Dairy Science, 74(10), 3275–3283.10.3168/jds.S0022-0302(91)78513-5
- Kumaran, E., Mahalakshmipriya, A., & Rajan, S. (2013). Effect of fish waste based protease in silver recovery from X-ray films. International Journal of Current Microbiology and applied Sciences, 2, 49–56.
- Lakshmi, G., & Prasad, N. N. (2015). Purification and characterization of alkaline protease from a Mutant Bacillus licheniformis Bl8. Advances in Biological Research, 9(1), 15–23.
- Larcher, G., Cimon, B., Symoens, F., Tronchin, G., Chabasse, D., & Bouchara, A. (1996). A 33 kDa serine proteinase from Scedosporium apiospermum. Biochemical Journal, 315, 119–126.10.1042/bj3150119
- Leng, Y. W., & Xu, Y. (2013). Improvement of acid protease production by a mixed culture of Aspergillus niger and Aspergillus oryzae using solid-state fermentation technique. African Journal of Biotechnology, 10, 6824–6829.
- Li, X., & Yu, H. Y. (2012). Purification and characterization of novel organic-solvent-tolerant β-amylase and serine protease from a newly isolated Salimicrobium halophilum strain LY20. FEMS Microbiology Letters, 329(2), 204–211.10.1111/fml.2012.329.issue-2
- Li, S., Yang, X., Yang, S., Zhu, M., & Wang, X. (2012). Technology prospecting on enzymes: Application, marketing and engineering. Computational and Structural Biotechnology Journal, 2, e201209017. doi:10.5936/csbj.201209017
- Li, Q., Yi, L., Marek, P., & Iverson, B. L. (2013). Commercial proteases: Present and future. FEBS Letters, 587, 1155–1163.10.1016/j.febslet.2012.12.019
- Lu, J., Wu, X., Jiang, Y., Cai, X., Huang, L., Yang, Y., … Li, A. (2014). An extremophile Microbacterium strain and its protease production under alkaline conditions. Journal of Basic Microbiology, 54, 378–385.10.1002/jobm.v54.5
- Manachini, P. L., Fortina, N. G., & Parini, C. (1998). Thermostable alkaline protease produced by Bacillus thermoruber – a new species of Bacillus. Applied Microbiology and Biotechnology, 28(4–5), 409–413.
- Mane, P., & Tale, V. (2015). Overview of microbial therapeutic enzymes. International Journal of Current Microbiology and applied Sciences, 4, 17–26.
- Martinez-Rosales, C., & Castro-Sowinski, S. (2011). Antarctic bacterial isolates that produce cold-active extracellular proteases at low temperature but are active and stable at high temperature. Polar Research, 30, 7123.10.3402/polar.v30i0.7123
- Marzan, L. W., Manchur, M. A., Hossain, M. T., & Anwar, M. N. (2001). Production of protease and amylase by Fusarium poae. Bangladesh Journal of Microbiology, 18(2), 127–134.
- Mathew, C. D., & Gunathilika, R. M. S. (2015). Production, purification and characterization of a thermostable alkaline serine protease from Bacillus licheniformis NMS-1. International Journal of Biotechnology and Molecular Biology Research, 6(3), 19–27.
- Miyaji, T., Otta, Y., Shibata, T., Mitsui, K., Nakagawa, T., Watanabe, T., … Tomizuka, N. (2005). Purification and characterization of extracellular alkaline serine protease from Stenotrophomonas maltophilia strain S-1. Letters in Applied Microbiology, 41(3), 253–257.10.1111/lam.2005.41.issue-3
- Miyoshi, S. I. (2013). Extracellular proteolytic enzymes produced by human pathogenic vibrio species. Frontiers in Microbiology, 4, 339.
- Mohamed, M. A. (2007). Purification and characterization of an alkaline protease produced by the bacterium Xenorhabdus nematophila BA2, a symbiont of entomopathogenic nematode Steinernema carpocapsae. Research Journal of Agriculture and Biological Science, 3(5), 510–521.
- Mothe, T., & Sultanpuram, V. R. (2016). Production, purification and characterization of a thermotolerant alkaline serine protease from a novel species Bacilluscaseinilyticus. 3. Biotech, 6(1), 53.
- Motyan, J. A., Toth, F., & Tozser, J. (2013). Research applications of proteolytic enzymes in molecular biology. Biomolecules, 3, 923–942.10.3390/biom3040923
- Muthulakshmi, C., Gomathi, D., Kumar, D. G., Ravikumar, G., Kalaiselvi, M., & Uma, C. (2011). Production, purification and characterization of protease by Aspergillus flavus under solid state fermentation. Jordan Journal of Biological Science, 4(3), 137–148.
- Naji, K. M., Abdullah, Q. Y. M., AlZaqri, A. Q. M., & Alghalibi, S. M. (2014). Evaluating the biodeterioration enzymatic activities of fungal contamination isolated from some ancient yemeni mummies preserved in the National Museum. Biochemistry Research International. ID 481508. doi:10.1155/2014/481508
- Nascimento, T. P., Sales, A. E., Porto, C. S., Brandao, R. M. P., Takaki, G. M. C., Teixeira, J. A. C., … Porto, A. L. F. (2015). Production and characterization of new fibrinolytic protease from Mucor subtillissimus UCP 1262 in solid-state fermentation. Advances in Enzyme Research, 3, 81–91.10.4236/aer.2015.33009
- Negi, S., Gupta, S., & Banerjee, R. (2011). Extraction and purification of glucoamylase and protease produced by Aspergillus awamori in a single-stage fermentation. Food Technology and Biotechnology, 49(3), 310–315.
- Neto, Y. A. A. H., Motta, C. M. D. S., & Cabral, H. (2013). Optimization of metalloprotease production by Eupenicillium javanicum in both solid state and submerged bioprocesses. African Journal of Biochemistry Research, 7(8), 146–157.
- Ng, W. J. (2001). Fermentation of starch for enhanced alkaline protease production by constructing an alkalophilic Bacillus pumilus strain. Applied Microbiology and Biotechnology, 57(2001), 153–160.
- Nissen, J. A. (1986). Enzymic hydrolysis of food proteins. New York: Elservier Applied Science.
- Niyonzima, F. N., & More, S. (2015). Detergent-compatible proteases: Microbial production, properties, and stain removal analysis. Preparative Biochemistry and Biotechnology, 45, 233–258.10.1080/10826068.2014.907183
- Novelli, P. K., Barros, M. M., & Fleuri, L. F. (2016). Novel inexpensive fungi proteases: Production by solid state fermentation and characterization. Food Chemistry, 198, 119–124.10.1016/j.foodchem.2015.11.089
- Obeid, A. E. F. E., Alawad, A. M., & Ibrahim, H. M. (2015). Isolation and characterization of Bacillus Subtillus with potential production of nattokinase. International Journal of Advance Research, 3(3), 94–101.
- Ogino, H., Tsuchiyama, S., Yasuda, M., & Doukyu, N. (2010). Enhancement of the aspartame precursor synthetic activity of an organic solvent-stable protease. Protein Engineering Design and Selection, 23(3), 147–152.10.1093/protein/gzp086
- Oseni, O. A. (2011). production of microbial protease from selected soil fungal isolates. Nigerian Journal of Biotechnology, 23, 28–34.
- Oyeleke, S. B., Egwim, E. C., & Auta, S. H. (2010). Screening of Aspergillus flavus and Aspergillus fumigatus strains for extracellular protease enzyme production. Journal of Microbiology and Antimicrobials, 2(7), 83–87.
- Padmapriya, M., & Williams, B. C. (2012). Purification and characterization of neutral protease enzyme from Bacillus subtilis. Journal of Microbiology and Biotechnology Research, 2(4), 612–618.
- Padmapriya, B., Thamaraichelvan, R., Nandita, R., & Raj, F. (2012). Production and purification of alkaline serine protease from marine Bacillus species and its application in detergent industry. European Journal of Applied Sciences, 4(1), 21–26.
- Palanivel, P., Ashokkumar, L., & Banagurunathan, R. (2013). Production, purification and fibrinolytic characterization of alkaline protease from extremophilic soil fungi. International Journal of Pharm Bio Sciences, 4(2), 101–110.
- Pant, G., Prakash, A., Pavani, J. V. P., Bera, S., Deviram, G. V. N. S., Kumar, A., … Prasuna, R. G. (2015). Production, optimization and partial purification of protease from Bacillus subtilis. Journal of Taibah University for Science, 9(1), 50–55.10.1016/j.jtusci.2014.04.010
- Patil, D. R., Rethwisch, D. G., & Dordick, J. S. (1991). Enzymatic synthesis of a sucrose-containing linear polyester in nearly anhydrous organic media. Biotechnology and Bioengineering, 37(7), 639–646.10.1002/(ISSN)1097-0290
- Patyar, S., Joshi, R., Prasad Byrav, D. S., Prakash, A., Medhi, B., & Das, B. K. (2010). Bacteria in cancer therapy: A novel experimental strategy. Journal of Biomedical Science, 17, 21.10.1186/1423-0127-17-21
- Paul, S. S., Kamra, D. N., & Sastry, V. R. (2010). Fermentative characteristics and fibrolytic activities of anaerobic gut fungi isolated from wild and domestic ruminants. Archives of Animal Nutrition, 64(4), 279–92.10.1080/17450391003625037
- Paul, T., Jana, A., Mandal, A. K., Mandal, A., Mohpatra, P. K., & Mondal, K. C. (2016). Bacterial keratinolytic protease, imminent starter for NextGen leather and detergent industries. Sustainable Chemistry and Pharmacy, 3, 8–22.10.1016/j.scp.2016.01.001
- Peng, Y., Huang, Q., Zhang, R. H., & Zhang, Y. Z. (2003). Purification and characterization of a fibrinolytic enzyme produced by Bacillus emyloliquefaciens DC-4 screened from Douchi, a traditional Chinese soybean food. Comparative Biochemistry and Physiology Part B: Biochemistry and Molecular Biology, 134, 45–52.10.1016/S1096-4959(02)00183-5
- Pervaiz, S., Hirpara, J. L., & Clement, M. V. (1998). Caspase proteases mediate apoptosis induced by anticancer agent preactivated MC540 in human tumor cell lines. Cancer Letters, 128(1), 11–22.10.1016/S0304-3835(98)00021-4
- Petra, K., & Monika, C. (2016). Spontaneous regression of tumour and the role of microbial infection – possibilities for cancer treatment. Anti Cancer Drugs, 27, 269–277.
- Pogson, M., Georgiou, G., & Iverson, B. L. (2009). Engineering next generation proteases. Current Opinion in Biotechnology, 20, 390–397.
- Qudar, S. A. U., Shireen, E., Iqbal, S., & Tuzun, C. Y. (2009). Optimization of protease production from newly isolated strain of Bacillus sp. PCSIR EA-3. Indian Journal of Biotechnology, 8, 286–290.
- Rai, S. K., Roy, J. K., & Mukherjee, A. K. (2010). Characterisation of a detergent-stable alkaline protease from a novel thermophilic strain Paenibacillus tezpurensis sp. nov. AS-S24-II. Applied Microbiology and Biotechnology, 85(5), 1437–1450.10.1007/s00253-009-2145-y
- Rajagopalan, G., & Krishnan, C. (2008). α-Amylase production from catabolite derepressed Bacillus subtilis KCC103 utilizing sugarcane bagasse hydrolysate. Bioresources Technology, 99(8), 3044–3050.10.1016/j.biortech.2007.06.001
- Rakashanda, S., & Amin, S. (2013). Proteases as targets in anticancer therapy using their inhibitors. Journal of Life Sciences, 5(2), 133–138.10.1080/09751270.2013.11885220
- Rathod, M. G., & Pathak, A. P. (2014). Wealth from waste: Optimized alkaline protease production from agro-industrial residues by Bacillus alcalophilus LW8 and its biotechnological applications. Journal of Taibah University for Science, 8(4), 307–314.10.1016/j.jtusci.2014.04.002
- Raut, S., Sen, S. K., Kabir, N. A., Satpathy, S., & Raut, S. (2012). Isolation and characterization of protease producing bacteria from upper respiratory tract of wild chicken. Bioinformation, 8(7), 326–330.10.6026/bioinformation
- Ray, A. K., Ghosh, K., & Ringø, E. (2012). Enzyme-producing bacteria isolated from fish gut: A review. Aquaculture Nutrition, 18, 465–492.10.1111/anu.2012.18.issue-5
- Rovati, J., Delgado, O. D., De Figueroa, L. I. C., & Fariña, J. I. (2013). Novel source of fibrinolytic activity: Bionectria sp., an unconventional enzyme-producing fungus isolated from Las Yungas rainforest (Tucumán, Argentina). World Journal of Microbiology and Biotechnology, 26, 55–62.
- Roy, T., Banerjee, G., Dan, S. K., & Ray, A. K. (2013). Optimization of fermentation conditions for phytase production by two strains of Bacillus licheniformis (LF1 and LH1) isolated from the intestine of rohu, Labeo rohita (Hamilton). Proceedings of the Zoological Society, 66(1), 27–35.10.1007/s12595-012-0057-9
- Rui, H., Qin, L., Qiyao, W., Ma, Y., Liu, H., Shi, C., & Zhang, Y. (2009). Role of alkaline serine protease, Asp, in Vibrio alginolyticus virulence and regulation of its expression by LuxO-LuxR regulatory system. Journal of Microbiology and Biotechnology, 19(5), 431–438.10.4014/jmb.0807.404
- Salevesen, G., & Nagase, H. (1983). Inhibition of proteolytic enzymens. In J. R. Beynon & J. S. Bond (Eds.), Poteolytic Enzymes; A Practical Approach (p. 83). Oxford: IRL Press.
- Sareen, R., & Mishra, P. (2008). Purification and characterization of organic solvent stable protease from Bacillus licheniformis RSP-09-37. Applied Microbiology and Biotechnology, 79(3), 399–405.10.1007/s00253-008-1429-y
- Sawant, R., & Nagendran, S. (2014). Protease: An enzyme with multiple industrial applications. World Journal of Pharmacy and Pharmaceutical Sciences, 3(6), 568–579.
- Saxena, R., & Singh, R. (2011). Characterization of a metallo-protease produced in solid state fermentation by a newly isolated Bacillus strain. Acta Biologica Szegediensis, 55(1), 13–18.
- Senthilraj, P., & Saravanakumar, K. (2011). Purification and characterization of protease from mangroves derived strain of Bacillus cereus. International Journal of Multidisciplinary Research, Journal, 1(1), 13–18.
- Sethi, S., & Gupta, S. (2015). Optimization of protease production from fungi isolated from soil. International Journal of Applied Biology and Pharmaceutical Technology, 3, 149–154.
- Shabbiri, K., Adnan, A., Jamil, S., Ahmad, W., Noor, B., & Rafique, H. M. (2012). Medium optimization of protease production by Brevibacterium linens DSM 20158, using statistical approach. Brazilian Journal of Microbiology, 43(3), 1051–1061.10.1590/S1517-83822012000300031
- Shankar, S., More, S. V., & Seeta Laxman, R. (2010). Recovery of silver from waste x-ray film by alkaline protease from Conidibolus coronatus. Kathmandu University Journal of Science Engineering and Technology, 6(I), 60–69.
- Sharma, N., & De, K. (2011). Production, purification and crystallization of an alkaline protease from Aspergillus tamari [EF661565.1]. Agriculture and Biology Journal of North America, 2(7), 1135–1142.10.5251/abjna.2011.2.7.1135.1142
- Sharma, A. K., Sharma, V., Saxena, J., Yadav, B., Alam, A., & Prakash, A. (2015). Optimization of protease production from Bacillus isolated from soil. Applied Research Journal, 7, 388–394.
- Shi, J., Coyne, V. E., & Weiner, R. M. (1997). Identification of an alkaline metalloprotease produced by the hydrothermal vent bacterium Hyphomonas jannaschiana VP3. Microbiology, 91, 15–26.
- Singh, J., Vohra, R. M., & Sahoo, D. K. (1999). Alkaline protease from a new obligate alkalophilic isolate of Bacillus sphaericus. Biotechnology Letters, 21(10), 921–924.10.1023/A:1005502824637
- Souza, P. M., Bittencourt, M. L. A., Caprara, C. C., de Freitas, M., de Almeida, R. P. C., Silveira, D., … Magalhães, P. O. (2015). A biotechnology perspective of fungal proteases. Brazilian Journal of Microbiology, 46, 337–346.10.1590/S1517-838246220140359
- Srilakshmi, J., Madhavi, J., Lavanya, S., & Ammani, K. (2014). Commercial potential of fungal protease: Past, present and future prospects. Journal of Pharmaceutical Chemical and Biological Sciences, 2(4), 218–234.
- Strausberg, S., Ruan, B., Fisher, K., Alexander, P., & Bryan, P. (2005). Directed coevolution of stability and catalytic activity in calcium-free subtilisin. Biochemistry, 44, 3272–3279.10.1021/bi047806 m
- Subba Rao, C., Sathish, T., Ravichandra, P., & Prakasham, R. S. (2009). Characterization of thermo- and detergent stable serine protease from isolated Bacillus circulans and evaluation of eco-friendly applications. Process Biochemistty, 44, 262–268.10.1016/j.procbio.2008.10.022
- Suntornsuk, W., & Suntornsuk, L. (2003). Feather degradation by Bacillus sp FK 46 in submerged cultivation. Bioresource Technology, 86, 239–243.10.1016/S0960-8524(02)00177-3
- Surendran, A., Vennison, S. J., Ravikumar, S., & Syed Ali, M. (2011). Optimization of alkaline protease production Bacillus sphaericus SBS4 by soil bacterium. Journal of Pharmacy Research, 4(5), 1517–1519.
- Sutar, I. I., Srinivasan, M. C., & Vartak, H. G. (1991). A low molecular weight alkaline proteinase from Conidiobolus coronatus. Biotechnology Letters, 13(2), 119–124.10.1007/BF01030462
- Takagi, H., Hirai, K., Maeda, Y., Matsuzawa, H., & Nakamori, S. (2000). Engineering subtilisin E for enhanced stability and activity in polar organic solvents. Journal of Biochemistry, 127, 617–625.10.1093/oxfordjournals.jbchem.a022649
- Taprig, T., Akaracharanya, A., Sitdhipol, J., Visessanguan, W., & Tanasupawat, S. (2013). Screening and characterization of protease-producing Virgibacillus, Halobacillus and Oceanobacillus strains from Thai fermented fish. Journal of Applied Pharmaceutical Science, 3(02), 025–030.
- Tavea, F., Fossi, B. T., Fabrice, N. T., Ngoune, L. T., & Ndjouenkeu, R. (2016). Production and partial characterization of an extracellular thermophile alkaline protease from a selected strain of Bacillus sp. isolated from abattoir soil in the North Region of Cameroon. Journal of Bioprocessimg & Biotechniques, 6, 279.
- Tekin, N., Cihan, A. C., Takac, Z. S., Tuzun, C. Y., Tunc, K., & Cokmus, C. (2012). Alkaline protease production of Bacillus cohnii APT5. Turkish Journal of Biology, 36(2012), 430–440.
- Thomas, P. G., Russell, A. J., & Fersht, A. R. (1985). Tailoring the pH dependence of enzyme catalysis using protein engineering. Nature, 28, 375–376.10.1038/318375a0
- Tripathi, P., Kukreja, N., Singh, B. P., & Arora, N. (2009). Serine protease activity of Cur l 1 from Curvularia lunata augments Th2 response in mice. Journal of Clinical Immunology, 29(3), 292–302.10.1007/s10875-008-9261-9
- Tsujibo, H., Miyamoto, K., Okamoto, T., Orikoshi, H., & Inamori, Y. (2000). A serine protease-encoding gene (aprII) of Alteromonas sp. strain O-7 Is regulated by the iron uptake regulator (Fur) protein. Applied and Environmental Microbiology, 66(9), 3778–3783.10.1128/AEM.66.9.3778-3783.2000
- Umar Dahot, M. (1994). Purification and some properties of alkaline protease from Penicillium expansum. Journal of Islamic Academy of Science, 7(2), 100–105.
- van der Maarel, M. J., van der Veen, B., Uitdehaag, J. C., Leemhuis, H., & Dijkhuizen, L. (2002). Properties and applications of starch-converting enzymes of the α-amylase family. Journal of Biotechnology, 94(2), 137–155.10.1016/S0168-1656(01)00407-2
- Vanitha, N., Rajan, S., & Murugesan, A. G. (2014). Optimization and production of alkaline protease enzyme from Bacillus subtilis 168 isolated from food industry waste. International Journal of Current Microbiology and Applied Sciences, 3(6), 36–44.
- Verma, A., Singh, H., Anwar, M. S., Ansari, M. W., & Agrawal, S. (2014). Production of alkaline protease from a haloalkaliphilic soil thermoactinomycete and its application in feather fibril disintegration. African Journal of Microbiology Research, 8(27), 2565–2573.
- Verma, A., Singh, H., Anwar, S., Chattopadhyay, A., Tiwari, K. K., Kaur, S., & Dhilon, G. S. (2016). Microbial keratinases: Industrial enzymes with waste management potential. Critical Review in Biotechnology, 13, 1–16.
- Verma, A., Singh, H., Anwar, S., Chattopadhyay, A., Tiwari, K. K., Kaur, S., & Dhilon, G. S. (2017). Microbial keratinases: Industrial enzymes with waste management potential. Critical Reviews in Biotechnology, 37(4), 476–491.10.1080/07388551.2016.1185388
- Vidyasagar, M., Prakash, S., Jayalakshmi, S. K., & Sreeramulu, K. (2007). Optimization of culture conditions for the production of halothermophilic protease from halophilic bacterium Chromohalobacter sp. TVSP101. World Journal of Microbiology and Biotechnology, 23(5), 655–662.10.1007/s11274-006-9279-1
- Vidyasagar, M., Prakash, S., Mahajan, V., Shouche, Y. S., & Sreeramulu, K. (2009). Purification and characterization of an extreme halothermophilic protease from a halophilic bacterium Chromohalobacter sp. TVSP101. Brazilian Journal of Microbiology, 40(1), 12–19.10.1590/S1517-83822009000100002
- Vojcic, L., Pitzler, C., Körfer, G., Jakob, F., Martinez, R., Maurer, K. H., & Schwaneberg, U. (2015). Advances in protease engineering for laundry detergents. New Biotechnology, 32(6), 629–634.10.1016/j.nbt.2014.12.010
- Wang, C., Du, M., Zheng, D., Kong, F., Zu, G., & Feng, Y. (2009). Purification and characterization of nattokinase fromBacillus subtilis Natto B-12. Journal of Agricultural and Food Chemistry, 57, 9722–9729.10.1021/jf901861v
- Wang, H., Yang, L., Ping, Y., Bai, Y., Luo, H., Huang, H., & Yao, B. (2016). Engineering of a Bacillus amyloliquefaciens strain with high neutral protease producing capacity and optimization of its fermentation conditions. PLoS ONE, 11(1), e0146373.10.1371/journal.pone.0146373
- wishard, R., Jaiswal, M., Parveda, M., Amareshwari, P., Bhadoriya, S. S., Rathore, P., … Nair, A. S. (2014). Identification and characterization of alkaline protease producing Bacillus firmus species EMBS023 by 16S rRNA gene sequencing. Interdisciplinary Science, 6(4), 271–278.10.1007/s12539-014-0187-z
- Xu, B. L., Dai, M., Chen, Y., Meng, D., Wang, Y., Fang, N., … Tang, B. (2015). Improving the thermostability and activity of a thermophilic subtilase by incorporating structural elements of its psychrophilic counterpart. Applied and Environmental Microbiology, 81, 6302–6313.10.1128/AEM.01478-15
- Xu, W., Shao, R., Wang, Z., & Yan, X. (2015). Improving the neutral phytase activity from Bacillus amyloliquefaciens DSM 1061 by site-directed mutagenesis. Applied Biochemistry and Biotechnology, 175(6), 3184–94.10.1007/s12010-015-1495-4
- Yadav, S., & Siddalingeshwara, K. G. (2015). Screening and biosynthesis of fibrinolytic enzyme from Aspergillus japonicum. Journal of Drug Delivery and Therapeutics, 5(6), 60–62.
- Yang, J. K., Shih, I. L., Tzeng, Y. M., & Wang, S. L. (2000). Production and purification of protease from a Bacillus subtilisthat can deproteinize crustacean wastes. Enzyme and Microbial Technology, 26(5–6), 406–413.10.1016/S0141-0229(99)00164-7
- Yin, L. J., Chou, Y. H., & Jiang, S. T. (2013). Purification and characterization of acidic protease from Aspergillus Oryzae BRRC 30118. Journal of Martine Science and Technology, 21(1), 105–110.
- Yoo, A. Y., & Park, J. K. (2016). Isolation and characterization of a serine protease-producing marine bacterium Marinomonas arctica PT-1. Bioprocess and Biosystems Engineering, 39(2), 307–314.10.1007/s00449-015-1514-4
- Zanphorlin, L. M., Cabral, H., Arantes, E., Assis, D., Juliano, M. A., Da-Silva, R., … Binilla-Rodriguez, G. O. (2011). Purification and characterization of a new alkaline serine protease from the thermophilic fungus Myceliophthora sp. Process Biochemistry, 46(11), 2137–2143.10.1016/j.procbio.2011.08.014
- Zhang, X. Y., Han, X. U., Chen, X. L., Dang, H. Y., Xie, B. B., Qin, Q. L., … Zhang, Y. Z. (2015). Diversity of cultivable protease-producing bacteria in sediments of Jiaozhou Bay, China. Frontiers in Microbiology. doi:10.3389/fmicb.2015.01021
- Zhang, K., Duan, X., & Wu, J. (2016). Multigene disruption in undomesticated Bacillus subtilis ATCC 6051a using the CRISPR/Cas9 system. Scientific Reports, 6. doi:10.1038/srep27943