Abstract
Purpose: The aim of the present study was to investigate whether induction of differentiation by hyperthermia is accompanied by apoptosis and necrosis to further evaluate the benefits of using hyperthermia as a differentiation inducing physical modality.
Materials and method: Differentiation was evaluated in K562 erythroleukaemia cells by measuring haemoglobin synthesis and flow cytometric measurement of glycophorin A expression. Apoptosis was measured by Annexin-V-FITC and Propidium Iodide (PI) double staining assay. Apoptosis and necrosis was also evaluated morphologically using staining with acridine orange/ethidium bromide (AO/EtBr) by fluorescence microscopy. Heat shock protein 70 (HSP70) level was measured by ELISA kit.
Results: Hyperthermia (43°C) induced differentiation as judged by increased haemoglobin synthesis and glycophorin A expression. No sign of apoptosis or necrosis could be detected at this temperature. Cell viability did not change due to heat treatment, and cellular proliferation was reduced in a dose (heating time) dependent manner. At 45°C, hyperthermia induced apoptosis and necrosis with minimal or no sign of differentiation. HSP70 level was significantly increased at 43°C along with differentiation of leukaemic cells, while at 45°C no significant effect on HSP70 production could be observed.
Conclusions: The encouraging results obtained here indicate that by heat treatment at 43°C, hyperthermia can be used alone or in combination with other modalities as a differentiation inducing agent without any detectable apoptotic activity. Positive correlation between HSP70 production and induction of differentiation and lack of apoptosis by hyperthermia confirm the possible role of HSP70 in the heat-induced differentiation and apoptosis in leukaemic cells.
Introduction
Tumour cells, and particularly leukaemic cells, can be considered as maturation-arrested cells which have escaped some normal control and continue to proliferate. Haematopoietic cells undergo three major fates: proliferation, differentiation, and apoptosis. These processes are closely intertwined. Under normal circumstances, haematopoietic cell proliferation and cell death are carefully balanced. Considerable evidence supports the notion that leukaemias are likely to arise from the disruption of the differentiation process of haematopoietic progenitors, which fail to give birth to blood cells with restricted phenotypes, as well as from diminished ability to undergo apoptosis Citation[1]. Recent reports support the key role of apoptotic mechanisms on the outcome of leukaemic patients Citation[2]. Therefore, new therapeutic approaches are being developed based on the induction of apoptosis by various agents Citation[3–6]. Preferential induction of apoptosis for primary human leukaemic stem cells has been achieved by some drugs Citation[7]. Several agents can induce apoptosis as well as differentiation in leukaemic cells Citation[8].
The maturation arrest of leukaemic cells can be reversed by differentiation inducing agents such as antitumour drugs currently used in conventional cytotoxic chemotherapy Citation[9] or immunosuppressive agents Citation[10], alkaloids from marine sponges Citation[11], and physical agents such as hyperthermia Citation[12]. In vitro differentiation of erythroleukaemia cells by chemical agents represents many aspects of the erythroid terminal differentiation programme, including haemoglobin synthesis and proliferation arrest Citation[13], and elevated production of red cell specific determinant Citation[14]. Despite the remarkable progress achieved in the treatment of leukaemias over the last several years, many problems still remain Citation[15].
Hyperthermia is now a well established physical modality which is used alone or in combination with other modalities such as radiation or chemicals in the treatment of malignancies or other pathological disorders Citation[16–20]. In almost all of these applications, hyperthermia is used as a cytotoxic agent that causes cell death or enhances the cytotoxic effects of other modalities. The cytotoxic behaviour of hyperthermia has been described and reviewed in many excellent papers Citation[21]. It has been shown that hyperthermia could induce apoptosis in leukaemic cell lines or enhance the apoptosis induced by other chemicals Citation[22–24].
Recently we showed that hyperthermia induced differentiation in leukaemic cell lines Citation[25]. In that work we did not address the critical question whether apoptosis, in addition to described differentiation, was also responsible for the observed decline in colony forming ability of cancer cells induced by hyperthermia.
In the current report we have carefully analysed the differentiation versus apoptosis induction by hyperthermia in a human erythroleukaemia cell line. We have also investigated the possible role that heat shock proteins may play in the specific effect of hyperthermia on this cell line.
Material and methods
Cell culture
The K562 cells were cultured in RPMI-1640 culture medium (Gibco: UK) supplemented with 10% heat-inactivated fetal bovine serum (FBS), 500 u/ml of penicillin (Sigma: USA) and 200 µg/ml streptomycin (Jaberebn-Hayan: Tehran). Cells were maintained at 37°C in a humidified atmosphere of 7.5% CO2 and subcultured every two to three days in order to maintain the cells in constant exponential growth phase.
Heat treatment
One ml of K562 cells at a density of 1 to 2 × 106 cells/ml in RPMI-1640 were heated by immersing the experimental glass tubes in thermostated water bath (Haake: Germany f3 with ± 0.1°C precision). Heat treatment was applied at 43°C and 45°C for different periods of time, and controls were treated similarly at 37°C. Cell viability was determined by trypan blue dye exclusion test immediately and at various times after heat treatment. Subsequently, for cell differentiation assay and apoptosis detection, heat-treated and control cells (5 × 104 cells/ml) were transferred into 25 or 60 mm culture Petri dishes (Nunc: Denmark) containing RPMI-1640 supplemented with 10% FBS. Cells were incubated for an additional 120 h, collected, and investigated using various assays described below. In order to measure the level of HSP70 protein, the cells were incubated for up to 8 h after heat treatment and then were used for further analysis.
Detection of erythroid differentiation in K562 cells
Benzidine staining assay for haemoglobin synthesis
The percentage of haemoglobin-producing cells was detected by benzidine staining as reported previously Citation[25]. Briefly, heat-treated and control K562 cells at 120 h post-incubation after heat treatment, were collected and resuspended in 20 µl of cell culture medium. Then 15 µl of the cell suspension was transferred into a cytospin tube and centrifuged at 500 rpm for 10 min. The slides were immersed in 30 ml total volume of benzidine stain containing 30% benzidine (1.5% benzidine in methanol) 20% H2O2 and 50% ethanol for 5 min, then rinsed in running water. The percentage of benzidine-positive cells (brown to orange coloured cells) was determined under light microscope.
Measuring of glycophorin A expression
The surface expression of glycophorin A antigen (CD235a) was detected by FITC-conjugated mouse anti-human glycophorin A monoclonal antibody (Clone NAM10-6G4, IQ Products, The Netherlands) using flow cytometry Citation[26]. Heat-treated and control K562 cells at 120 h post-incubation after heat treatment, were washed and resuspended in cold PBS at a density of 107cells/ml. Then 5 µl of the appropriate FITC-conjugated antibody was added to 50 µl of cell suspension. After 15 min incubation on ice (4°C), the cells were washed with PBS and analysed by flow cytometry. The cell population for analysis was gated using forward scatter versus side scatter parameters. At least 1 × 104 cells were collected for each samples and the percentage of glycophorin A-positive cells was determined using LYSIS II software (Becton-Dickenson: USA). As positive and negative control for glycophorin A expression, cells were treated with sodium butyrate (1 mM, Merck: Germany) and hemin (50 µM, Sigma: USA) respectively.
Determination of apoptosis in K562 cells
Analysis of apoptosis by Annexin-V/PI double staining assay
Apoptotic cells were detected by Annexin-V-FITC and Propidium Iodide (PI) double staining assay Citation[27]. In brief, cells (5 × 105) were washed with PBS and labelled by annexin-V-FITC and PI in binding buffer according to the instruction in the Annexin-V-FLUOS Staining Kit provided by the manufacturer (Roche, Germany). Fluorescent signals of FITC and PI were detected, respectively, by FL1 (FITC detector) at 518 nm and FL2 at 620 nm on FACScan (Becton-Dickenson: USA). For each analysis, 1 × 104 events were recorded and data were analysed using the LYSIS II program (Becton-Dickenson: USA).
Morphological study of apoptosis and necrosis
Apoptosis and necrosis was also determined morphologically using staining with acridine orange/ethidium bromide (AO/EtBr) by fluorescence microscopy Citation[28]. Cells were washed with PBS and adjusted at a density of 1 × 106 cells/ml in PBS. Acridine orange/ethidium bromide solution (1:1 v/v) was added to the cell suspension in a final concentration of 100 µg/ml. The cellular morphology was evaluated by Axoscope 2 plus fluorescence Spmicroscopy from ZEISS (Germany). At least 100 cells were counted to calculate the fraction of apoptotic and necrotic cells.
Protein isolation and analysis
Cells were harvested at 0, 4 and 8 h after heat treatment and then washed with PBS. The pellet was lysed in 200 µl of cold lysis buffer at 4°C for 30 min (Hsp70 Extraction Reagent provided in Hsp70 ELISA Kit (Stressgen, Canada) used as the lysis buffer). The lysate was then centrifuged at 4°C, 12000 rpm for 20 min to pellet large cellular debris. The supernatant was then collected and kept frozen (−70°C) for further analysis to determine the protein concentration and measure the level of HSP70. Protein concentration was measured using the Bradford method. The level of HSP70 protein was determined using Hsp70 ELISA Kit (Stressgen, Canada) following the kit instruction.
Statistical analysis
Data in this report are presented as Mean ± SEM and were analysed by Student's t-test. A P-value of ≤0.05 was considered statistically significant.
Results
The effects of hyperthermia on viability and proliferation of K562 cells
K562 cells were heated at 43°C and 45°C for different periods of time, and then were cultured for several hours and studied for viability and capacity of proliferation. Our results showed that heat treatment at 43°C did not have any significant effect on the viability of these cells, as assessed by trypan blue dye exclusion test, since more than 90% of cells were viable after 120 h of incubation at 37°C (). However, heat treatment at 45°C reduced the viability of the cells in a dose- and time-dependent manner to about 60% after 120 h of incubation at 37°C (). As shown in , cell proliferation was affected by hyperthermia. At 43°C, the growth of K562 cells was inhibited in a dose- and time-dependent manner (), and heat treatment at 45°C totally inhibited the growth of these cells ().
Figure 1. The effect of hyperthermia on the viability (A and B) and growth (C and D) of K562 cells at various times after heat treatment. Cells were heat treated at 43°C (A and C) and 45°C (B and D) for different periods of time. The number of cells and their viability was determined 24, 72 and 120 h of incubation at 37°C after heat treatment. Values are Mean ± SEM from three independent experiments.
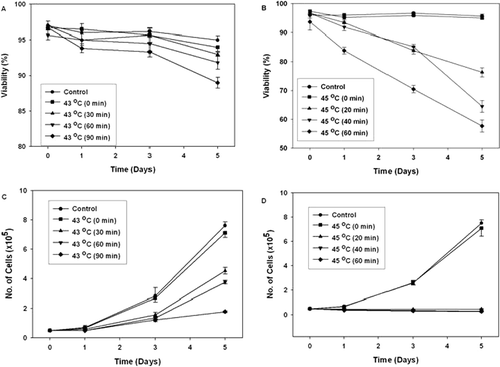
Induction of differentiation by hyperthermia in K562 cells
The effect of hyperthermia on the induction of differentiation on K562 cells was assessed by measuring the haemoglobin synthesis and the glycophorin A expression. Benzidine staining allows easy and rapid distinction between differentiated haemoglobin-containing (benzidine positive) and undifferentiated (benzidine negative) K562 cells. Our results showed that 90 min of heat treatment at 43°C stimulated haemoglobin synthesis by 7.1 fold (). Hyperthermia at 43°C also stimulated the expression of the erythroid-specific membrane antigen glycophorin A. Our results showed that heat treatment at 43°C increased the fraction of glycophorin A positive cells by 2 folds ( and ). These results supported that heat treatment at 43°C induced differentiation of K562 cells into erythroid linage. However, we did not detect any significant induction of differentiation in heat-treated cells at 45°C ().
Figure 2. The effect of hyperthermia on glycophorin A expression in K562 cells. Cells were heat treated for different periods of time at 43°C and incubated for 120 hr after heat treatment at 37°C. Then the cells were stained with FITC-conjugated anti glycophorin A, and the green fluorescence of the stained cells was measured by flow cytometry. (A–D) K562 cells were heat treated at 43°C for 0 min, 30 min, 60 min and 90 min, respectively. (E) As positive control for glycophorin A expression, cells were treated with sodium butyrate (1mM). (Dark Gray line: Control (37°C); Light Gray line: Heat or Sodium butyrate treatement).
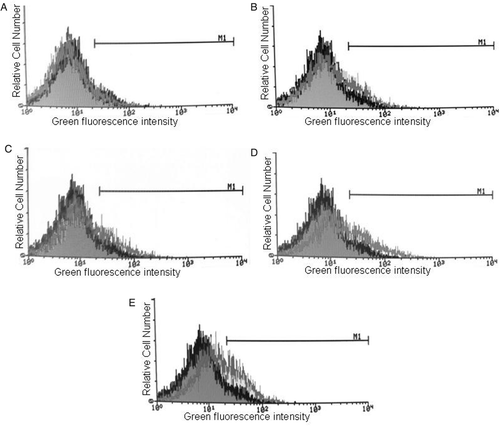
Table I. The effect of hyperthermia (43°C and 45°C) on induction of differentiation (haemoglobin synthesis and glycophorin A expression) in K562 cells. Cells were heated at these temperatures for different periods of time and incubated for 120 h after hyperthermia. As positive controls, cells were treated with sodium butyrate (SB) and hemin. The fraction of benzidine positive cells and glycophorin A-expressing cells are represented as Mean ± SEM from three independent experiments. B+ cells: Benzidine positive cells, Gly A-exp cells: Glycophorin A-expressing cells. Compared with control (37°C); *P < 0.05; ♦P < 0.01.
Induction of apoptosis by hyperthermia in K562 cells
Apoptosis was detected by the surface exposure of phosphatidylserine on the outer leaflet of apoptotic cell-membranes using annexin-V-FITC. Propidium iodide (PI) was used for the simultaneous detection of necrotic or late apoptotic cells. Analysis of the double stained cells by flow cytometry revealed that heat treatment at 43°C did not have any significant effect on the induction of apoptosis or necrosis in K562 cells; the results of the quantitative analysis are summarized in the .
Table II. The effect of hyperthermia (43°C) on induction of apoptosis in K562 cells. Cells were heated at 43°C for different periods of time and studied at 24, 72 and 120 h after heat treatment. Annexin-V and PI staining were measured by flow cytometry. The results of quantitative analysis of Annexin-V/PI staining are represented as mean ± SEM from three independent experiments.
Flow cytometric diagrams of heat-treated cells at 45°C showed that this temperature induced apoptosis and/or necrosis in these cells (). The result of quantitative analysis showed that at 24 h post-incubation after heat treatment, the percentage of early apoptotic cells were 13.93 ± 0.49%, 21.05 ± 2.83%, and 12.53 ± 0.80% in heat-treated cells at 45°C for 20, 40 and 60 min, respectively, as compared to 3.7 ± 0.47% in control (37°C). The percentage of late apoptotic/nectrotic cells increased to more than 20% after 120 h of post-hyperthermia incubation in cells which were heat treated at 45°C for 40 and 60 min, as compared to 2% in the control cells (37°C).
Figure 3. The effect of hyperthermia on Annexin-V binding/PI uptake in K562. Cells were heated at different periods of time at 45°C and incubated for 24 h (A), 72 h (B) and 120 h (C) after heat treatment. Annexin-V and PI staining were measured by flow cytometry. The dual fluorescence dot plots show the normal (viable) cells in the lower left quadrant (Annexin−/PI−), the early apoptotic cells in the lower right quadrant (Annexin+/PI−) and the late apoptotic or necrotic cells in the upper right quadrant (Annexin+/PI+). Cell debris was excluded from the analysis by conventional gating of forward scatter versus side scatter dot plots. X axis: The fluorescence intensity of Annexin-V-FITC and Y axis: The fluorescence intensity of Propidium Iodide (PI).
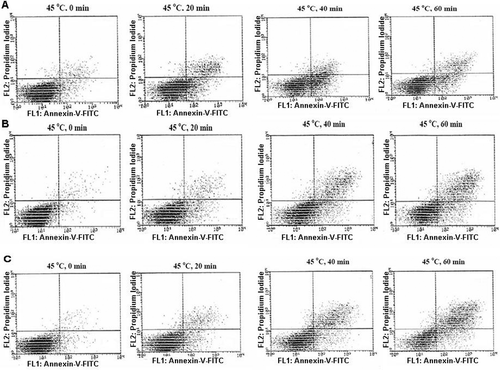
In addition, induction of apoptosis or necrosis in heat-treated cells at 45°C was also determined morphologically after staining with Acridine orange and ethidium bromide by fluorescence microscopy (). In this figure, viable cells are uniformly green, early apoptotic cells are green with bright green dots in their nuclei as a consequence of chromatin condensation and nuclear fragmentation. Ethidium bromide incorporates into the late apoptotic and necrotic cells, therefore these cells are orange, and in contrast to necrotic cells late apoptotic cells show condensed fragmented nuclei. In accordance with the data from flow cytometry, AO/EtBr double staining of K562 cells showed that heat treatment at 45°C induced apoptosis in K562 cells. In addition, these results indicate that in this temperature necrosis is mediated in cell death processes. The results of quantitative analysis are shown in .
Figure 4. Morphological assessment of apoptosis and necrosis in K562 cells. Cells were heat treated at 45°C for different periods of time and studied 72 h after heat treatment. AO/EtBr double staining of K562 cells shows viable cells are uniformly green, early apoptotic cells are green with bright green dots in their nuclei (green arrows) and late apoptotic cells (orange arrows) are orange and in contrast to necrotic cells (orange arrows, N), they show condense fragmented nuclei. (A) Control K562 cells, (B-E) cells were heat treated at 45°C for 0, 20, 40 and 60 min, respectively. Cells were observed under fluorescence microscope at 400×.
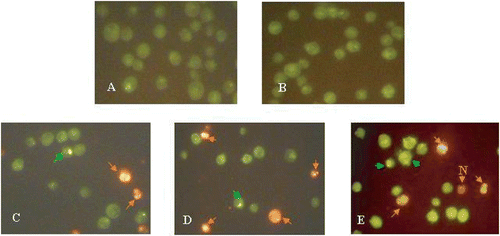
Table III. The effect of hyperthermia (45°C) on induction of apoptosis and necrosis in K562 cells. Cells were heated at 45°C for different periods of time and studied at 72 h after heat treatment. The results of quantitative analysis of AO/EtBr double staining are represented as mean ± SEM. Compared with control (37°C); *P < 0.05, ♦P < 0.01.
The effect of hyperthermia on HSP70 protein level
The relationship between HSP70 protein and differentiation or apoptosis induced by heat shock was studied by applying hyperthermia at 43°C and 45°C for different periods of time. The level of HSP70 was measured at 0, 4 and 8 h after heat treatment using the ELISA HFP70 kit as described in the method section.
As can be seen in , the expression of HSP70 was significantly increased following 30, 60, and 90 min of heat treatment at 43°C as compared to control samples. While, hyperthermia at 45°C did not induce the expression of HSP70 in heated cells and the level of HSP70 did not change significantly after this heat treatment as compared to control ().
Figure 5. The effect of hyperthermia on HSP70 level in K562 cells. Cells were heat treated at 43°C (A) and 45°C (B) for different periods of time and studied 0, 4 and 8 h after heat treatment. The x-axis shows the temperature and the time of heating, the y-axis shows the level of HSP70 as determined by the ELISA HSP70 kit. The results are expressed as the ratio of HSP70 protein/total protein from two independent experiments (each performed in duplicate). P < 0.02 compared to the control samples (37°C).
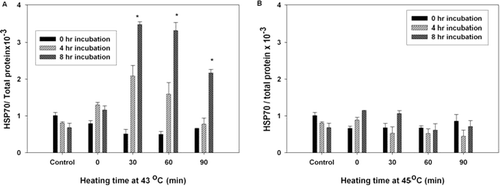
Discussion
Leukaemia is a disease characterized by the failure of cell death, or inability of haematopoietic cells to differentiate into functional mature cells Citation[1]. Therefore, induction of differentiation or cell death in immature haematopoietic cells has been applied for leukaemia prevention or therapy Citation[29], Citation[30]. The effect of hyperthermia on cultured tumour cells has occasionally been reported and it was shown that hyperthermia can induce differentiation Citation[12], Citation[25], Citation[31], Citation[32] or apoptosis Citation[33] in tumour cells depending on the tumour cell type and stress intensity.
The results in this work indicated that heat treatment at 43°C reduced the growth of K562 cells without affecting their viability. Heat treatment at this temperature also induced erythroid differentiation in these cells as judged by two different markers, induction of haemoglobin synthesis and increased glycophorin A expression. These two markers are the most important makers of erythroid differentiation of K562 cell line Citation[34], Citation[35]. Hyperthermia can induce glycophorin A expression as well as haemoglobin synthesis. Therefore, this physical agent can induce terminal differentiation in K562 cells, although a significant but rather low degree of haemoglobin synthesis was induced by hyperthermia. Some differentiation inducing agents, such as hemin, although are more potent in the induction of haemoglobin synthesis Citation[36] as compared to hyperthermia, however they lack the ability to induce glycophorin A. These agents did not induce terminal differentiation in K562 cells. It has been shown that certain reagents which are known to effect the differentiation of K562 cells, possess a different mechanism of action on these cells, at least in respect of haemoglobin synthesis and glycophorin A expression Citation[35]. Our data also demonstrated that hyperthermia at 43°C did not have any significant effect on the induction of apoptosis in K562 cells as evidenced by flow cytometric methods.
While, heat treatment at 45°C inhibited the growth of K562 cells and reduced their viability, but no evidence of differentiation (haemoglobin synthesis or increased glycophorin A expression) could be detected in heat-treated cells. Apoptosis was detected in K562 heat-treated cells (45°C) by the detection of Annexin-V-positive cells and changes in cell morphology (chromatin condensation and nuclear fragmentation). It is possible that K562 cells switch from differentiation to cell death depending on the stress intensity, but little is known about the molecular mechanism underlying these phenomena.
Several studies demonstrated that some chemical agents could induce differentiation in leukaemic cells by blocking cell proliferation Citation[29], Citation[30], Citation[37–39]. Therefore, it is possible that these chemical agents, through inhibition of proliferation, allow leukaemic cells to recover their normal programme of differentiation Citation[1]. It has also been shown that treatment of cells with some chemical agents at subtoxic concentrations may serve to stress the cells and induce some kind of adaptive or stress response such as induction of heat shock protein expression Citation[32]. It is possible that these events may result in changes of specific genes expression leading to cell differentiation, including changes in the expression of certain oncogenes such as myc family Citation[40], Citation[41], that involve in the control of cell growth and differentiation in leukaemic cells Citation[42]. In addition, several studies have suggested a role for heat shock proteins (HSPs) during development and differentiation in different organisms Citation[43], Citation[44]. It has been shown that heat shock proteins (HSP70 and HSP90) can interact with multiple key component of signaling pathway that regulates growth, differentiation and cell death Citation[45], including nuclear hormone receptors Citation[46], Citation[47], tyrosine-and serine/threonine kinases Citation[48], Citation[49] and cell death regulators Citation[50], Citation[51]. Several studies have also shown that HSP70 and HSP90 associate with many components and regulators of the Ras/Raf-1 pathway, which has an important role in proliferation, differentiation, growth arrest and cell death Citation[52], Citation[53]. In addition, the selective expression of HSP70 and HSP28 during differentiation of myeloid leukaemic cells treated with chemical agents suggested a role for these heat shock proteins in differentiation process of these cells Citation[54], Citation[55]. The role of heat shock proteins in differentiation of leukaemic cells has been also reviewed in many excellent papers Citation[56], Citation[57].
Our results also demonstrated that hyperthermia at 43°C increased HSP70 level, while, heating cells at 45°C had no significant effect on the level of HSP70 in heat-treated cells. On the other hand, the differentiation inducing effects of hyperthermia was observed at 43°C, while at 45°C hyperthermia acted as a cytotoxic and apoptosis inducing agent. Therefore, the HSP70 can act as a positive modulator in differentiation process of heat-treated leukaemic cells.
On the other hand, hyperthermia can induce both apoptosis and necrosis in a temperature-dependent manner. It is accepted that hyperthermia (at moderate temperature) triggers apoptosis, while higher temperature or more prolonged heat treatment induce necrosis in many cell types Citation[58], Citation[59]. The fact that hyperthermia (at 43°C) did not induce apoptosis in the K562 cells, while heat treatment at 45°C could induce apoptosis and partially necrosis in these cells could rather be explained with the well known resistance of K562 cells to drug-induced apoptosis Citation[60]. This resistance has been attributed to the activity of P210 (bcr-abl) which transduced anti-apoptotic signals acting on different targets such as Stat1, Stat5, Bcl-xl and Akt Citation[61], Citation[62]. In addition, K562 cells have been shown to express high levels of the anti-apoptosis protein Bcl-xl, a member of the Bcl-2 related family of apoptosis modulators Citation[63].
Strasser and Anderson have shown that both Bcl-2 family and heat shock proteins protected cells from heat-induced apoptosis. They suggested that these Bcl-2 and heat shock proteins act via independent mechanisms to inhibit apoptosis in heat-treated cells Citation[64]. It is possible that heat shock induces two independent pathways leading to apoptosis; Bcl-2 may inhibit one pathway and heat shock proteins the other. However, little is known about the molecular mechanisms underlying these phenomena. Several studies have indicated that heat treatment of cells leads to many alterations, including inactivation of the normal pattern of genetics expression at various levels (transcription and translation), inhibition of cell proliferation (DNA synthesis and cell division) and alterations in cell morphology and cell adhesion Citation[65]. Indeed, many cellular structures are affected by heat treatment Citation[66]. The loss of structural integrity of subcellular components and consequently the loss of their cellular functions may be a consequence of protein denaturation. Therefore, protein denaturation/aggregation may be the main process leading to thermal killing and thermotolerance may be acquired via protection against this protein damage with the aid of HSPs Citation[67].
It has been hypothesized that HSPs are used by stressed cells either to protect against stress-induced alteration in protein structure, or to repair and restore the structure and functional integrity of damaged proteins Citation[68], Citation[69].
On the other hand, several studies indicated a role for HSP70 in protecting the tumour cells against the toxicity effects of different apoptosis-inducing agents. It has been also shown that over-expression of hsp70 gene protected the fibroblast cells against the lethal effect of heat treatment Citation[69].
Considering our results, increasing the level of HSP70 in heat-treated cells (43°C) protected the cells from lethal effects of heat treatment and lead to their differentiation, while in severe treatment condition (45°C) which no increase of HSP70 was observed, the cells triggered to apoptosis. Therefore, the HSP70 may protect the cells against heat-induced apoptosis.
In conclusion, our results first suggested that hyperthermia had powerful growth inhibition, differentiation and apoptosis inducing capacity in human erythroleukaemia cell line. Although many regulating factors are involved in the pathways that regulate differentiation and apoptosis in leukaemic cells, our results shown that HSP70 may play a role in the control of these processes in heat-treated cells.
Acknowledgement
This work was supported by educational grant from University of Tehran.
References
- Czyz M, Szulawska A. [Induced differentiation of the K562 leukaemic cell line.]. Postepy Hig Med Dosw (Online) 2005; 59: 82–97
- Del Principe MI, Del Poeta G, Venditti A, Buccisano F, Maurillo L, Mazzone C, Bruno A, Neri B, Irno CM, Amadori S. Apoptosis and immaturity in acute myeloid leukemia. Hematology 2005; 10: 25–34
- Das Gupta S, Debnath A, Saha A, Giri B, Tripathi G, Vedasiromoni JR, Gomes A. Indian black scorpion (Heterometrus bengalensis Koch) venom induced antiproliferative and apoptogenic activity against human leukemic cell lines U937 and K562. Leuk Res. 2007; 31: 817–825
- Grzanka A, Grzanka D, Orlikowska M. Cytoskeletal reorganization during process of apoptosis induced by cytostatic drugs in K-562 and HL-60 leukemia cell lines. Biochem Pharmacol 2003; 66: 1611–1617
- Kizaki M. New therapeutic approach for myeloid leukemia: Induction of apoptosis via modulation of reactive oxygen species production by natural compounds. Int J Hematol 2006; 83: 283–288
- Fang L, He Q, Hu Y, Yang B. MZ3 induces apoptosis in human leukemia cells. Cancer Chemother Pharmacol 2006; Cancer Chemother Pharmacol. 2007; 59: 397–405
- Guzman ML, Swiderski CF, Howard DS, Grimes BA, Rossi RM, Szilvassy SJ, Jordan CT. Preferential induction of apoptosis for primary human leukemic stem cells. Proc Natl Acad Sci USA 2002; 99: 16220–16225
- Hasinoff BB, Abram ME, Barnabe N, Khelifa T, Allan WP, Yalowich JC. The catalytic DNA topoisomerase II inhibitor dexrazoxane (ICRF-187) induces differentiation and apoptosis in human leukemia K562 cells. Mol Pharmacol 2001; 59: 453–461
- Jeannesson P, Lahlil R, Chenais B, Devy L, Gillet R, Aries A, Morceau F, Trentesaux C. Anthracyclines as tumor cell differentiating agents: Effects on the regulation of erythroid gene expression. Leuk Lymphoma 1997; 26: 575–587
- Mischiati C, Sereni A, Lampronti I, Bianchi N, Borgatti M, Prus E, Fibach E, Gambari R. Rapamycin-mediated induction of gamma-globin mRNA accumulation in human erythroid cells. Br J Haematol 2004; 126: 612–621
- Aoki S, Kong D, Matsui K, Kobayashi M. Erythroid differentiation in K562 chronic myelogenous cells induced by crambescidin 800, a pentacyclic guanidine alkaloid. Anticancer Res 2004; 24: 2325–2330
- Goliaei B, Deizadji A. Effects of hyperthermia and granulocyte-macrophage colony-stimulating factor on the differentiation of human leukemic cell line U937. Leuk Res 1998; 22: 705–710
- Matushansky I, Radparvar F, Rekhtman N, Skoultchi A. Reprogramming erythroleuekmia cells to terminal differentiation and terminal cell division. Front Biosci 2000; 5: D488–492
- Horton MA, Cedar SH, Edwards PA. Expression of red cell specific determinants during differentiation in the K562 erythroleukaemia cell line. Scand J Haematol 1981; 27: 231–240
- Tsiftsoglou AS, Pappas IS, Vizirianakis IS. Mechanisms involved in the induced differentiation of leukemia cells. Pharmacol Ther 2003; 100: 257–290
- Issels RD. High-risk soft tissue sarcoma: Clinical trial and hyperthermia combined chemotherapy. Int J Hyperthermia 2006; 22: 235–239
- Ito A, Honda H, Kobayashi T. Cancer immunotherapy based on intracellular hyperthermia using magnetite nanoparticles: A novel concept of “heat-controlled necrosis” with heat shock protein expression. Cancer Immunol Immunother 2006; 55: 320–328
- Li GC, He F, Ling CC. Hyperthermia and gene therapy: Potential use of microPET imaging. Int J Hyperthermia 2006; 22: 215–221
- Masunaga S, Nagasawa H, Sakurai Y, Uto Y, Hori H, Nagata K, Suzuki M, Maruhashi A, Kinashi Y, Ono K. The usefulness of mild temperature hyperthermia combined with a newly developed hypoxia-oriented 10B conjugate compound, TX-2100, for boron neutron capture therapy. Int J Hyperthermia 2006; 22: 287–299
- Schulze T, Wust P, Gellermann J, Hildebrandt B, Riess H, Felix R, Rau B. Influence of neoadjuvant radiochemotherapy combined with hyperthermia on the quality of life in rectum cancer patients. Int J Hyperthermia 2006; 22: 301–318
- Takahashi I, Emi Y, Hasuda S, Kakeji Y, Maehara Y, Sugimachi K. Clinical application of hyperthermia combined with anticancer drugs for the treatment of solid tumors. Surgery 2002; 131: S78–S84
- Mauz-Korholz C, Dietzsch S, Schippel P, Banning U, Korholz D. Molecular mechanisms of hyperthermia- and cisplatin-induced cytotoxicity in T cell leukemia. Anticancer Res 2003; 23: 2643–2647
- Mauz-Korholz C, Dietzsch S, Banning U, Trobs RB, Korholz D. Heat- and 4-hydroperoxy-ifosfamide-induced apoptosis in B cell precursor leukaemias. Int. J. Hyperthermia. 2003; 19: 444–460
- Bartkowiak D, Hogner S, Baust H, Nothdurft W, Rottinger EM. Comparative analysis of apoptosis in HL60 detected by annexin-V and fluorescein-diacetate. Cytometry 1999; 37: 191–196
- Goliaei B, Rafiei M, Soheili Z. Effects of hyperthermia on the differentiation and growth of K562 erythroleukemic cell line. Leuk Res 2004; 28: 1323–1328
- Hatse S, Schols D, De Clercq E, Balzarini J. 9-(2-Phosphonylmethoxyethyl)adenine induces tumor cell differentiation or cell death by blocking cell cycle progression through the S phase. Cell Growth Differ 1999; 10: 435–446
- van Engeland M, Nieland LJ, Ramaekers FC, Schutte B, Reutelingsperger CP. Annexin V-affinity assay: A review on an apoptosis detection system based on phosphatidylserine exposure. Cytometry 1998; 31: 1–9
- Saydam G, Aydin HH, Sahin F, Selvi N, Oktem G, Terzioglu E, Buyukkececi F, Omay SB. Involvement of protein phosphatase 2A in interferon-alpha-2b-induced apoptosis in K562 human chronic myelogenous leukaemia cells. Leuk Res 2003; 27: 709–717
- Huang WQ, Wang BH, Wang QR. Thymosin beta4 and AcSDKP inhibit the proliferation of HL-60 cells and induce their differentiation and apoptosis. Cell Biol Int 2006; 30: 514–520
- Huang WW, Yang JS, Lin CF, Ho WJ, Lee MR. Pycnogenol induces differentiation and apoptosis in human promyeloid leukemia HL-60 cells. Leuk Res 2005; 29: 685–692
- Cellier MF, Taimi M, Chateau MT, Cannat A, Marti J. Thermal stress as an inducer of differentiation of U937 cells. Leuk Res 1993; 17: 649–656
- Richards FM, Watson A, Hickman JA. Investigation of the effects of heat shock and agents which induce a heat shock response on the induction of differentiation of HL-60 cells. Cancer Res 1988; 48: 6715–6720
- Falcieri E, Luchetti F, Burattini S, Canonico B, Santi S, Papa S. Lineage-related sensitivity to apoptosis in human tumor cells undergoing hyperthermia. Histochem Cell Biol 2000; 113: 135–144
- Andersson LC, Jokinen M, Gahmberg CG. Induction of erythroid differentiation in the human leukaemia cell line K562. Nature 1979; 278: 364–365
- Kawasaki N, Morimoto K, Tanimoto T, Hayakawa T. Control of haemoglobin synthesis in erythroid differentiating K562 cells. I. Role of iron in erythroid cell heme synthesis. Arch Biochem Biophys 1996; 328: 289–294
- Rutherford TR, Clegg JB, Weatherall DJ. K562 human leukaemic cells synthesise embryonic haemoglobin in response to haemin. Nature 1979; 280: 164–165
- Chenais B, Molle I, Trentesaux C, Jeannesson P. Time-course of butyric acid-induced differentiation in human K562 leukemic cell line: Rapid increase in gamma-globin, porphobilinogen deaminase and NF-E2 mRNA levels. Leukemia 1997; 11: 1575–1579
- Nyoung MN, Trentesaux C, Aries A, Carpentier Y, Jardillier JC, Gorisse MC, Jeannesson P. Effect of aclacinomycin-doxorubicin association on differentiation and growth of human erythroleukemic K562 cells. Anticancer Res 1994; 14: 1203–1208
- Zhang LP, Jiang JK, Tam JW, Zhang Y, Liu XS, Xu XR, Liu BZ, He YJ. Effects of Matrine on proliferation and differentiation in K-562 cells. Leuk Res 2001; 25: 793–800
- Colotta F, Polentarutti N, Staffico M, Fincato G, Mantovani A. Heat shock induces the transcriptional activation of c-fos protooncogene. Biochem Biophys Res Commun 1990; 168: 1013–1019
- Bukh A, Martinez-Valdez H, Freedman SJ, Freedman MH, Cohen A. The expression of c-fos, c-jun, and c-myc genes is regulated by heat shock in human lymphoid cells. J Immunol 1990; 144: 4835–4840
- Li CY, Zhan YQ, Xu CW, Xu WX, Wang SY, Lv J, Zhou Y, Yue PB, Chen B, Yang XM. EDAG regulates the proliferation and differentiation of hematopoietic cells and resists cell apoptosis through the activation of nuclear factor-kappa B. Cell Death Differ 2004; 11: 1299–1308
- Zakeri ZF, Wolgemuth DJ. Developmental-stage-specific expression of the hsp70 gene family during differentiation of the mammalian male germ line. Mol Cell Biol 1987; 7: 1791–1796
- Walsh D, Grantham J, Zhu XO, Wei Lin J, van Oosterum M, Taylor R, Edwards M. The role of heat shock proteins in mammalian differentiation and development. Environ Med 1999; 43: 79–87
- Nollen EA, Morimoto RI. Chaperoning signaling pathways: Molecular chaperones as stress-sensing ‘heat shock’ proteins. J Cell Sci 2002; 115: 2809–2816
- Davies TH, Ning YM, Sanchez ER. A new first step in activation of steroid receptors: Hormone-induced switching of FKBP51 and FKBP52 immunophilins. J Biol Chem 2002; 277: 4597–4600
- Zeiner M, Gehring U. A protein that interacts with members of the nuclear hormone receptor family: Identification and cDNA cloning. Proc Natl Acad Sci USA 1995; 92: 11465–11469
- Cutforth T, Rubin GM. Mutations in Hsp83 and cdc37 impair signaling by the sevenless receptor tyrosine kinase in Drosophila. Cell 1994; 77: 1027–1036
- Kimura Y, Rutherford SL, Miyata Y, Yahara I, Freeman BC, Yue L, Morimoto RI, Lindquist S. Cdc37 is a molecular chaperone with specific functions in signal transduction. Genes Dev 1997; 11: 1775–1785
- Beere HM, Wolf BB, Cain K, Mosser DD, Mahboubi A, Kuwana T, Tailor P, Morimoto RI, Cohen GM, Green DR. Heat-shock protein 70 inhibits apoptosis by preventing recruitment of procaspase-9 to the Apaf-1 apoptosome. Nat Cell Biol 2000; 2: 469–475
- Takayama S, Sato T, Krajewski S, Kochel K, Irie S, Millan JA, Reed JC. Cloning and functional analysis of BAG-1: A novel Bcl-2-binding protein with anti-cell death activity. Cell. 1995; 80: 279–284
- Wang HG, Takayama S, Rapp UR, Reed JC. Bcl-2 interacting protein, BAG-1, binds to and activates the kinase Raf-1. Proc Natl Acad Sci USA 1996; 93: 7063–7068
- Kolch W. Meaningful relationships: The regulation of the Ras/Raf/MEK/ERK pathway by protein interactions. Biochem J 2000; 351 Pt 2: 289–305
- Mivechi NF, Park YM, Ouyang H, Shi XY, Hahn GM. Selective expression of heat shock genes during differentiation of human myeloid leukemic cells. Leuk Res 1994; 18: 597–608
- Minowada G, Welch W. Variation in the expression and/or phosphorylation of the human low molecular weight stress protein during in vitro cell differentiation. J Biol Chem 1995; 270: 7047–7054
- Ciocca DR, Calderwood SK. Heat shock proteins in cancer: Diagnostic, prognostic, predictive, and treatment implications. Cell Stress Chaperones 2005; 10: 86–103
- Thomas X, Campos L, Le QH, Guyotat D. Heat shock proteins and acute leukemias. Hematology 2005; 10: 225–235
- Fairbairn DW, Walburger DK, Fairbairn JJ, O’Neill KL. Key morphologic changes and DNA strand breaks in human lymphoid cells: Discriminating apoptosis from necrosis. Scanning 1996; 18: 407–416
- Poe BS, O'Neill KL. Inhibition of protein synthesis sensitizes thermotolerant cells to heat shock induced apoptosis. Apoptosis 1997; 2: 510–517
- McGahon A, Bissonnette R, Schmitt M, Cotter KM, Green DR, Cotter TG. BCR-ABL maintains resistance of chronic myelogenous leukemia cells to apoptotic cell death. Blood 1994; 83: 1179–1187
- Henderson YC, Guo XY, Greenberger J, Deisseroth AB. Potential role of bcr-abl in the activation of JAK1 kinase. Clin Cancer Res 1997; 3: 145–149
- Wang W, Yao LB, Liu XP, Feng Q, Shang ZC, Cao YX, Sun BZ. Effects of STI571 and p27 gene clone on proliferation and apoptosis of K562 cells. World J Gastroenterol 2005; 11: 2130–2135
- Ray S, Bullock G, Nunez G, Tang C, Ibrado AM, Huang Y, Bhalla K. Enforced expression of Bcl-XS induces differentiation and sensitizes chronic myelogenous leukemia-blast crisis K562 cells to 1-beta-D-arabinofuranosylcytosine-mediated differentiation and apoptosis. Cell Growth Differ 1996; 7: 1617–1623
- Strasser A, Anderson RL. Bcl-2 and thermotolerance cooperate in cell survival. Cell Growth Differ 1995; 6: 799–805
- Hildebrandt B, Wust P, Ahlers O, Dieing A, Sreenivasa G, Kerner T, Felix R, Riess H. The cellular and molecular basis of hyperthermia. Crit Rev Oncol Hematol 2002; 43: 33–56
- Laszlo A. The effects of hyperthermia on mammalian cell structure and function. Cell Prolif 1992; 25: 59–87
- Kampinga HH. Thermotolerance in mammalian cells. Protein denaturation and aggregation, and stress proteins. J Cell Sci 1993; 104: 11–17
- Jaattela M. Heat shock proteins as cellular lifeguards. Ann Med 1999; 31: 261–271
- Li WX, Chen CH, Ling CC, Li GC. Apoptosis in heat-induced cell killing: The protective role of hsp-70 and the sensitization effect of the c-myc gene. Radiat Res 1996; 145: 324–330