Abstract
Purpose: Bio-reduction/activation of anti-cancer drug β-lapachone (β-lap) is mediated by NAD(P)H: Quinone oxidoreductase (NQO1). We investigated the feasibility of using mild temperature hyperthermia to increase the anti-cancer effect of β-lap by up-regulating NQO1 expression.
Methods: NQO1 expression in FSaII fibrosarcoma of C3H mice and A549 human lung cancer cells was evaluated with Western blot analysis and immunostaining of cells at different times after water-bath heating. Clonogenic cell survival method was used to determine the sensitivity of cells to heating, β-lap, and in combination. The growth of FSaII tumors in the right hind legs of C3H mice was studied after heating the tumors at 42°C for 1 h with water bath, an i.p. injection of β-lap to host mice or an i.p. injection of β-lap 24 h after heating the tumors.
Results: Heating at 42°C for 1 h significantly increased the expression of NQO1 in the cancer cells with a maximum increase occurring 8–24 h after heating. The sensitivity of cancer cells to β-lap treatment progressively increased until 24 h after heating most likely due to the increase in NQO1 expression. Heating the FSaII tumors at 42°C for 1 h and treating the host mice with an i.p. injection of 50 mg/kg β-lap 24 h after the tumor heating was far more effective than heating alone or β-lap treatment alone to suppress the tumor growth.
Conclusion: Mild temperature heat shock elevates the NQO1 expression in cancer cells, which in turn markedly increases the sensitivity of the cells to the bioreductive drug β-lap in vitro and in vivo.
Introduction
The feasibility of using hyperthermia to activate transfected target genes in gene therapy for cancer has been investigated in recent years. For example, prostate cancer cells were infected with a recombinant adenovirus containing suicide gene under the control of heat-inducible Hsp70 promoter, and heat shock was applied to activate the genes Citation[1], Citation[2]. Likewise, heat shock was used to activate cytotoxic genes such as IL-12 and TNF in tumor cells following infection of the cells with adenoviral vector coding for the genes under the control of Hsp70 promoter Citation[3]. Ku70 is a DNA-targeting component of DNA-PK, and as such, it promotes DNA-PK-mediated repair of radiation-induced DNA double-strand breaks. Infecting cancer cells with adenovirus vectors containing antisense Ku-70 RNA linked to Hsp70 promoter and heating the cells markedly reduced the endogenous Ku70, and thus, reduced the ability of DNA-PK to repair radiation damage in vitro and in vivo Citation[4]. Unfortunately, the effectiveness of these approaches was severely limited due to the difficulty of transfecting cells with sufficient amounts of the target genes. We have reported that ionizing radiation with clinically relevant doses, i.e. 2–4 Gy Citation[5–7] or mild hyperthermia at 42°C for 1 h Citation[8], significantly elevate the expression of endogenous NAD(P)H: Quinone oxidoreductase (NQO1), which mediated the activation of certain quinone-containing drugs, pointing to the possibility that radiation or hyperthermia may be used to sensitize cancer cells to certain drugs by activating endogenous genes.
NQO1 is a cytosolic flavoenzyme which reduces a broad range of quinone compounds to hydroquinones through two-electron reduction using electrons supplied by NADH or NAD(P)H Citation[9–16]. NQO1 has been known to activate mitomycin C (MMC) or its analogue, EO9, to cytotoxic alkylating agents Citation[17]. Other quinone compounds known to be bioactivated to cytotoxic agent by NQO1 are benzoquinone-containing alkylating agents such as carbazilquinone or aziridinylbenzoquinone RHI Citation[18], benzoquinones ansamycins (17-AAG) Citation[19] and other antitumor antibiotics such as streptonigrin and lavendamycin Citation[20]. Importantly, the NQO1 level in malignant tumor is significantly elevated compared to that in adjacent normal tissues Citation[21–25], indicating tumor tissues may be preferentially damaged relative to normal tissues by the NQO1-dependent bioreductive drugs. β-lapachone (β-lap) (3,4-dihydro-2,2-dimethyl-2H-naphthol{1,2-b}pyran-5,6-dione), a-naphthoquinone, is a novel anti-cancer drug, which has recently been demonstrated to be activated by NQO1.
β-lap was originally extracted from the inner bark of the lapacho tree, which is abundant in South America, particularly Brazil. The leaves and bark of lapacho trees have been used as tea or herbal medicine by the local people for centuries. Recently, β-lap has been demonstrated to induce apoptosis and necrotic death in a variety of cancer cells Citation[26–36]. Furthermore, β-lap synergistically kills cancer cells with ionizing radiation Citation[5–7, 37], heat shock Citation[8], and certain chemotherapy drugs Citation[32]. Divergent mechanisms have been proposed to account for the anti-cancer effect of β-lap. We have reported that synergistic cell death caused by β-lap and ionizing radiation was due to following two distinct mechanisms: (a) radiation-induced activation of NQO1 renders cells sensitive to β-lap, and (b) the activated β-lap in turn inhibits the repair of radiation damage Citation[5–7]. We have also reported for the first time that mild temperature hyperthermia can sensitize cancer cells in vitro and in vivo to β-lap by up-regulating the cellular NQO1 Citation[8]. The purpose of the present study was to further reveal factors for the effective use of hyperthermia to sensitize cells to β-lap by elevating the cellular NQO1 level.
Materials and experimental procedures
Cells
FSaII fibrosarcoma cells of C3H mice and A549 human lung cancer cells were used. FSaII cells were originally obtained from Dr H. Suit's laboratory, Massachusetts General Hospital, Boston. The early generation FSaII cells have been stored in liquid nitrogen and are periodically thawed and used. The A549 cell line was purchased from the American Type Culture Collection (Manassa, VA) and has been stored in liquid nitrogen. FSaII cells were cultured in RPMI 1640 medium (Life Technologies, Grand Island, NY) containing 10% bovine calf serum (Hyclone Laboratoeis, Inc., Logan, UT). The A549 cells were cultured in Dulbecco's Minimum Essential Medium (DMEM) (Gibco-BRL, Grand Island, NY) supplemented with 10% fetal bovine serum (Hyclone Laboratories Inc., Logan, UT). The media also contained penicillin (50 units/mL) and streptomycin (50 µg/mL). The cells were cultured in T-type plastic flasks in a humidified 95% air-5% CO2 atmosphere in a 37°C incubator.
For in vitro studies, exponentially growing cells in culture flasks were treated with 0.25% trypsin for 10 min and the resultant single cells were washed twice with medium containing 10% bovine serum. The number of cells was counted by a hemocytometer, appropriate numbers of the cells were seeded in 25 cm2 plastic tissue culture flasks with 5 mL medium, incubated overnight, and used for experiments.
β-lapachone
β-lap (3,4-dihydro-22,2-dimethyl-2H-naphthol {1,22b}pyran-5,6-dione) (Sigma Co., St. Louis, MO) was dissolved in dimethyl sulfoxide at 5 mM and stored frozen at 20°C. Immediately before use, the stock β-lap was thawed and diluted to desired concentration with either regular medium for in vitro experiments or in β-hydroxypropyl-β-cyclodextrin (Sigma Co, St Louis, MO) for in vivo experiments.
Clonogenic survival assay
The effects of β-lap alone, heating alone, and combination of both on the clonogenicity of cells were studied. For the studies on the effect of β-lap alone on cell survival, the medium in the flask was replaced with β-lap solution in medium and the cells were incubated at 37°C for varying lengths of time. The cells were then gently rinsed twice with medium and cultured with complete medium in a tissue culture incubator at 37°C for 7–10 days. The colonies formed were fixed with a mixture of methanol and acetic acid (10:1 v/v), stained with 1% crystal violet, and the colonies containing more than 50 cells were counted. For heating of the cells, the culture flasks were tightly closed with caps, the caps areas were sealed with wax paper, and then the cells were heated for 1 h by immersing the flasks into a water bath preheated to 40–42°C. After heating, the medium in each flask was replaced with complete medium, the cells were incubated in a tissue culture incubator at 37°C, and the colonies formed were counted 7–10 days later, as described above. For the study on the combined effect of heating and β-lap on cell survival, cells were heated first as described above, treated with β-lap either immediately after heating or after maintaining the cells for varying lengths of time in a tissue culture incubator at 37°C. After β-lap treatment, the cells were washed, incubated in a tissue culture incubator at 37°C for 7–10 days, and then the colonies formed were counted Citation[5–8].
Immunostaining and confocal microscopy for NQO1 in cells
The effect of heating on the expression of NQO1 in cells was examined by immunostaining the cells. Cells were plated on tissue culture chamber slides, maintained under a 95% air-5% CO2 atmosphere overnight, and then heated at 42°C for 1 h by immersing the chamber into a pre-heated water-bath. After replacing the medium with fresh complete medium, the cells were incubated in a tissue culture incubator at 37°C for varying lengths of time, washed with phosphate buffered saline (PBS), and fixed with acetone/methanol (1:1) for 20 min. After blocking with 1% bovine serum albumin, the cells were incubated with anti-NQO1 antibody (Santa Cruz Biotech, Inc, Santa Cruz, Ca) (1:100 dilution with PBS), then incubated with secondary antibody conjugated with FITC (Jackson Immuno Research Laboratory, Inc., West Grove, PA) for 1 h. After washing with PBS four times, cells were examined for NQO1 expression with a confocal laser scanning fluorescence microscope Citation[5],Citation[7],Citation[8].
Western blot analysis for NQO1 expression
The effect of heating on the expression of NQO1 was studied using the Western blot method. After cells were heated, as described above, the medium in the flasks was replaced with fresh complete medium and the flasks were returned to a tissue culture incubator at 37°C. At various times after heating, cells were harvested using 25% trypsin solution, washed twice with ice-cold PBS and dissolved in solubilizing buffer (pH 7.4, 1% Triton X-100, 0.1% SDS, 20 mM Tris-HCl, 150 mM NaCl, 1 mM EDTA, 1 mM sodium orthovanadate, 1 mM sodium fluoride, 2 mM PMSF, 10 mM iodoacetamide, 10 µG/ml aprotinin and 10 ug/ml leupeptin). The lysed samples containing 10–30 µg protein were placed on 15% sodium dodecyl sulfate polyacrylamide-gel and electrophoresed. The polypeptides were then transblotted from the gel onto Hybond-P (Amersham Life Sciences, Inc., Arlington Heights, IL) in transfer buffer (192 mM glycine, 25 mM sodium dodecyl sulfate, and 10% methanol). Blots were blocked with 3% non-fat dry milk in pH 7.4 TBST, treated with anti-NQO1 antibody (1:100 dilution in PBS) (Santa Cruz Biotech, Santa Cruz, Ca), and the bands were visualized by treatment with chemiluminescence. The density of blots was determined with a Biorad densitometer.
Tumor growth study
The effects of heating alone, β-lap treatment alone, or combination of the two modalities on the growth of FSaII tumors of C3H mice were studied. FSaII cells grown in culture were harvested with trypsin treatment, washed twice with serum-free RPMI medium and re-suspended in the serum-free medium. About 2.0 × 105 cells in 0.05 mL of serum-free medium were injected subcutaneously into the right hind legs of female C3H mice weighing 20–22 g. When the tumors had grown to 180 –200 mm3, mice were lightly anesthetized with an intraperitoneal (i.p.) injection of a mixture of 100 mg/kg ketamine and 10 mg/kg xylazine, and the tumors were heated by immersing the tumor-bearing legs into a 42°C water-bath for 1 h as previously described Citation[8]. At 24 h after tumor heating, host animals were injected i.p. with approximately 0.2 ml of β-lap solution in β-hydroxypropyl-β-cyclodextrin at 50 mg/kg body weight. Other groups of tumors were treated with heating alone or β-lap alone. Tumor diameter was measured with a caliper, and tumor volume (V) was calculated using the V = a2b/2 formula of which a is the shortest tumor diameter and b is the longest tumor diameter Citation[5],Citation[7],Citation[8]. When tumor size reached to approximately 1.8 cm3, the host mice were euthanized by cervical dislocation under anesthesia. All the animal experiments were performed following protocol (Protocol Number 0112A13064) approved by the University of Minnesota Institutional Animal Care Use Committee.
Statistics
Data was analysed using the two-tailed Student's test, and a difference with p value smaller than 0.05 was considered to be statistically significant.
Results
NQO1 expression
Western blot
shows the Western blots for NQO1 expression in FSaII cells and A549 cells before and 0–24 h after heating at 42°C for 1 h. In FSaII cells, the NQO1 protein level slightly increased 4–8 h after heating, and increased further 16–24 h after heating. Before heating, the constitutive NQO1 level in A549 cells was considerably higher than that in FSaII cells. The NQO1 protein level in A549 cells slightly increased 4–8 h after heating, and markedly increased 16–24 h after heating. The densities of NQO1 blots at different times after heating, as determined with a Biorad densitometer, are shown in . The NQO1 level in FSaII cells and A549 cells increased about 2.2 times and 2.5 times, respectively, 16–24 h after heating and then began to recede thereafter in both cell lines.
Figure 1. Changes in expression of NQO1 in tumor cells after heating at 42°C for 1 h. (A) Western blots of NQO1 in FSaII fibrosarcoma cells of C3H mice and A549 human lung cancer cells. (B) Relative changes in NQO1 levels in FSaII cells and A549 cells, as determined from the density of the Western blots. Averages of 4 determinations with 1 SD are shown. (C) Immunofluorescent staining for NQO1 in FSaII cells and A549 cells at different times after heating at 42°C for 1 h.
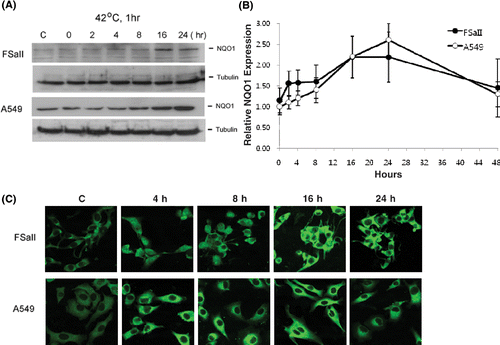
Immunofluorescence microscopy
The confocal microscopy of immunofluorescence staining for NQO1 in FSaII cells and A549 cells after heating at 42°C for 1 h is shown in . Consistent with the Western blot analysis, the immunostaining for NQO1 significantly increased in both FSaII cells and A549 cells 4–24 h after heating at 42°C for 1 h.
β-lap sensitivity of FSaII cells and A549 cells
The effects of a 4 h incubation with different concentrations of β-lap on the clonogenic survival of FSaII cells and A549 cells are shown in . The survival slightly decreased when the cells were treated for 4 h with 2.5 µM β-lap and then exponentially decreased as the dose of β-lap was increased. Slightly more death occurred in A549 cells than in FSaII cells when treated with 10 µM β-lap. However, the difference between the β-lap sensitivity of FSaII cells and A549 cells was not significant.
Changes in β-lap sensitivity after heating
The clonogenic survivals of FSaII cells and A549 cells heated first and then treated with β-lap at different times thereafter are shown in . Heating at 42°C for 1 h or β-lap treatment at 5 µM for 4 h reduced the clonogenic survival of FSaII cells to 92.0 ± 4.1% and 10.3 ± 0.5%, respectively. When FSaII cells were heated at 42°C for 1 h and immediately treated with 5 µM β-lap for 4 h, the cell survival decreased to 2.9 ± 0.7%. The cell death caused by the combined treatment progressively increased as the β-lap treatment was delayed up to 24 h after heating. It is shown that only 0.72 ± 0.08% of FSaII cell could survive when β-lap treatment was applied 24 h after heating. If the cell death caused by the combination of heating (92.0%) and β-lap treatment (10.3%) was additive, the cell survival would be about 9.5%, as indicated by the dotted line. Essentially similar effects were observed with A549 cells. Heating alone and β-lap treatment alone reduced cell survival to 90.5 ± 5.5% and 11.2 ± 0.8%, respectively. The survival of A549 cells decreased to 8.2 ± 0.9% when cells were heated and then immediately treated with β-lap. The combined effect to kill the cells progressively increased as the interval between heating and β-lap treatment was increased. The cell survival decreased to 3.9 ± 0.7% when β-lap treatment was applied 24 h after heating. If the combined effect of heating (90.5% survival) and β-lap treatment (11.2%) was merely additive, about 10% of cells would have survived, as indicated by dotted line. These results clearly indicated that the combination of heating and β-lap treatment killed FSaII cells and A549 cells in a manner more than additive, and that the combined cytotoxicity progressively increased as the interval between heating and β-lap treatment was increased up to 24 h.
Figure 3. Changes in β-lap sensitivity after heating. Clonogenic survivals of FSaII cells (A) and A549 cells (B) were determined after the cells were heated at 42°C for 1 h and then treated with 5 µM β-lap for 4 h at various times after heating. The effects of heating alone or β-lap treatment alone are also shown. The dotted lines indicate the expected percentage cell survival if the combined effect of heating and β-lap treatment was additive. Averages of 6–7 experiments with duplicate cultures and 1 SE are shown.
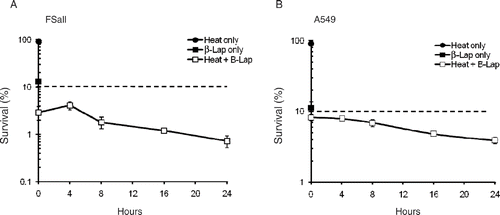
Effect of heating at different temperatures on the response of cells to β-lap
shows that the survival of FSaII cells decreased to 11.9 ± 0.7% when the cells were treated with 5 µM β-lap for 4 h at 37°C. When the cells were heated at 40°C for 1 h and then treated 24 h later with 5 µM β-lap for 4 h, the cell survival decreased to 1.6 ± 0.3%. The cell death caused by the combination of heating and β-lap treatment separated by 24 h increased as the pre-heating temperature was raised. For example, 0.75 ± 0.05% of cells survived when cells were heated at 42°C and treated 24 later with β-lap, which was significantly lower than 2.9 ± 0.7% survival of the cells treated with β-lap immediately after heating () (p < 0.05). As shown in , the survival of A549 cells heated at 40°C for 1 h and then treated 24 h later with 5 µM β-lap for 4 h decreased to 6.5 ± 0.9%, while 9.2 ± 2.3% of cells survived when cells were treated with β-lap without pre-heating. When heated at 42°C and treated 24 h later with 5 µM β-lap for 4 h, the cell survival was 3.4 ± 0.4%, which was significantly lower than 8.2 ± 0.9% survival of A549 ells treated with β-lap immediately after heating () (p < 0.05). These results indicated that the increase in the sensitization of cells to β-lap by pre-heating was dependent on the heating temperature.
Figure 4. Effect of pre-heating at different temperatures on the clonogenic survival of FSaII cells and A549 cells to β-lap treatment applied 24 h after heating. Black columns: cells were treated with 5 µM β-lap for 4 h at 37°C. Gray columns: cells were heated at different temperatures for 1 h. Open columns: cells were heated at different temperatures for 1 h, and then treated 24 h later with 5 µM β-lap for 4 h. Averages of 5–7 experiments with duplicate cultures and 1 SE are shown.
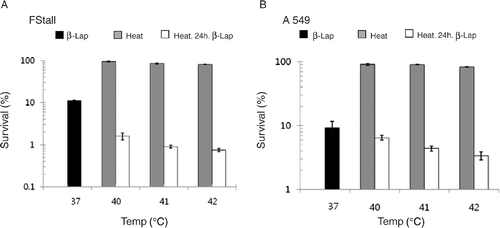
Tumor growth delay
The effects of various treatments on the growth of subcutaneous FSaII tumors established in the right legs of C3H mice are shown in . The average tumor volume at the time of treatment was 190 ± 18 mm3 and the volume of control tumors increased five times (950 mm3) in 9.8 ± 1.1 days. The volume of tumors heated at 42°C for 1 h or treated with an i.p. injection of β-lap at 50 mg/kg increased five times in 10.7 ± 1.3 days and 11.4 ± 1.5 days, respectively, which corresponded to, respectively, 1.09-fold longer and 1.16-fold longer than the control tumor growth time, 9.8 days. When tumors were first heated at 42°C, and the host mice were then treated 24 h later with an i.p. injection of β-lap at 50 mg/kg, the tumor volume increased five times in 15.9 ± 1.5 days, which was 1.6-fold longer than that for the control tumors. If the combined effect of heating and β-lap treatment to suppress the tumor growth was merely additive effect, the tumor growth time may be expected to increase by 1.26-fold (1.09 × 1.16). Since the combined treatment increased the tumor growth time by 1.6-fold, it may be reasonable to conclude that heating and β-lap suppressed tumor growth in a manner more than additive.
Figure 5. Growth of FSaII tumors in the hind legs of C3H mice after receiving various treatments. Heat alone: tumors were heated for 1 h with 42°C water-bath. β-lap alone: host mice were treated with an i.p. injection of 50 mg/kg β-lap. Heat, 24 h, β-lap: tumors were heated, and then the host mice were treated 24 h later with β-lap. Averages of 7–8 tumors and 1 SE are shown.
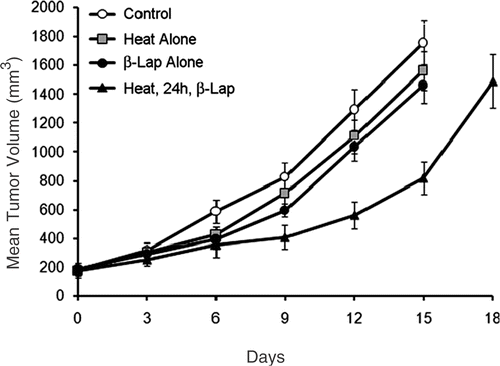
Discussion
We have demonstrated that mild heat shock is able to activate endogenous NQO1 in cancer cells, thereby sensitizing cells to the NQO1-dependent β-lap in vitro as well as in vivo. The β-lap sensitivity of cancer cells progressively increased as the time between heating and β-lap treatment was increased up to 24 h after heating at 40–42°C for 1 h.
The mechanisms underlying the cell death caused by β-lap have been controversial. A school of investigators reported that β-lap induces apoptosis by perturbing cell cycle progression Citation[30],Citation[33],Citation[35]. On the other hand, Boothman and his colleagues Citation[26–29], Citation[35], Citation[36] proposed that obligatory two-electron reductase NQO1 induces two-electron reduction of β-lap to hydroquinone using NADH or NAD(P)H as electron sources. The resultant two-electron reduced form of β-lap (hydroquinone) is unstable and it spontaneously reverts to its formal oxidized form resulting in a futile cycling between oxidized and reduced forms. In the process of oxidation, the two-electron reduced β-lap is first oxidized to one-electron reduced form, semiquinone. The unpaired electron of the semiquinone is then transferred to an oxygen molecule resulting in formation of reactive oxygen species (ROSs), thereby inducing DNA damage. Additionally, PARP-1 is hyperactivated, and NAD+ and ATP are depleted, which then leads to intracellular elevation of Ca2+. The elevation of Ca2+ increases the activity of Ca2+-dependent proteases and induces degradation of vital proteins, resulting in apoptotic or necrotic death.
The expression of NQO1 gene has been reported to be regulated mainly by the antioxidant response element (ARE) identified in the cis-element gene promoter Citation[38]. Various oxidative stresses, such as ionizing radiation, UV irradiation, xenobiotics, hypoxia and heavy metals, have been reported to elevate NQO1 expression by affecting the ARE Citation[39]. For the first time, we have reported that mild heat shock has the ability to significantly elevate the expression of NQO1 in cancer cells Citation[7]. The detailed molecular mechanism underlying the heat-induced up-regulation of NQO1 is being investigated in our laboratory. The results shown in demonstrate that although the NQO1 level in FSaII fibrosarcoma cells of C3H mice before heating was much less than that in A549 human lung cancer cells, FSaII cells were as sensitive as A549 cells to β-lap (). It has been known that subtle structural differences around the active center of NQO1 in different mammalian cell lines greatly influence the ability of NQO1 to cause reduction of chemotherapeutic quinones Citation[40–42]. It would be reasonable to conclude that the NQO1 in FSaII cells before heating was more proficient than that in A549 cells in causing two-electron reduction of β-lap.
Heated FSaII cells and A549 cells were significantly more sensitive to β-lap treatment than unheated control cells () most likely as a consequence of the increase in NQO1 level after heating (). In support of this conclusion, we previously observed that dicoumarol, a NQO1 inhibitor, significantly prevented the heat-induced increase in β-lap sensitivity in FSaII cells Citation[7]. In this regard, we have also reported that a radiation-induced increase in β-lap sensitivity of cancer cells could be significantly suppressed by dicoumarol or siRNA-NQO1 Citation[5–8]. The results shown in demonstrate that a more than additive cell death occurred when cells were heated and then treated with β-lap. Importantly, the sensitivity of cells to β-lap, demonstrated by the decline in clonogenic cell survival, progressively increased as the interval between heating and β-lap treatment was increased up to 24 h.
In FSaII cells, β-lap treatment 24 h after heating reduced cell survival to 0.72 ± 0.08%, which was approximately 7% of 10.3 ± 0.5% survival observed when cells were treated with β-lap alone. In A549 cells, the β-lap treatment applied 24 h after heating reduced survival to 3.9 ± 0.3% which was approximately 35% of the 11.2% survival observed in the cells treated with β-lap alone. These results imply that the relative increase in sensitivity to β-lap during 24 h after 42°C heating was much greater in FSaII cells than that in A549 cells, although the NQO1 expression significantly increase in the A549 cells. This result may be attributed to the aforementioned possibility that NQO1 in FSaII cells is more effective than NQO1 in A549 cells. Anther possible reason for the relatively small increase in the sensitivity to β-lap in A549 cells despite the significant increase in NQO1 level by heating may be related to the presence of a threshold for the bioreductive activity of NQO1 Citation[43]. It was observed that once the threshold value of NQO1 is achieved, activation of a bioreductive drug is only slightly increased by a further increase in NQO1 activity Citation[43]. It is highly probable that the NQO1 activity in A549 cells was already close to a threshold value before heating and thus a further increase in NQO1 level by heating caused only a modest increase in bioreduction of β-lap and its cytotoxicity.
The results of the tumor growth study () shows that an i.p. injection of 50 mg/kg β-lap to tumor-bearing mice without tumor heating caused only a small tumor growth delay whereas β-lap was cytotoxic in vitro as demonstrated in . It is conceivable that the dose of β-lap reached the target tumor cells after an i.p. injection of 50 mg/kg was insufficient to cause significant cell death. On the other hand, when the same dose of β-lap was administered 24 h after heating the tumors at 42°C for 1 h, significant tumor growth delay occurred probably due to heat-induced elevation of NQO1 level in the tumor cells. It is an established fact that the level of NQO1 in many human tumors is higher than that in normal tissues Citation[21–25]. The results of our studies strongly indicated that localized heating of human tumors at temperatures as low as 40°C may further elevate NQO1 levels in the tumors, and selectively potentiate the response of tumors to β-lap and perhaps other bio-reductive anti-cancer drugs.
Acknowledgements
This work was supported by following grants: the National R&D Program for Cancer Control, Ministry of Health & Welfare, Republic of Korea (0620340-1), National Nuclear Technology Program (2007) from the KOSEF, the Korea Health 21 R&D Project, Ministry of Health & Welfare, Republic of Korea (A062254) and RO1 CA116721 from NIH (USA).
References
- Blackburn RV, Galofore SS, Corry PM, Lee YJ. Adenoviral-mediated transfer of a heat-inducible double suicide gene into prostate carcinoma cells. Cancer Res 1998; 58: 1358–1362
- Borrellli MJ, Schoenherr DM, Wong A, Bernock LJ, Corry PM. Heat-activated transgene expression from adenovirus vectors infected into human prostate cancer cells. Cancer Res 2001; 61: 1113–1121
- Huang Q, Hu JK, Lohr F, Zhang L, Brown R, Lanzen J, Little JB, Dewhirst MW, Li CY. Heat-induced gene expression as a novel targeted cancer gene therapy strategy. Cancer Res 2000; 60: 3435–3439
- Li GC, He F, Shao X, Urano M, Shen L, Kim D, Borrelli M, Leiberl SA, Gutin PH, Ling CC. Adenovirum-mediated heat-activated antisense Ku70 expression radiosensitizes tumor cells in vitro and in vivo. Cancer Res 2003; 63: 3268–3274
- Park HJ, Ahn KJ, Ahn SD, Choi E, Lee SW, Williams B, Kim EJ, Griffin R, Bey EA, Bornmann WG, et al. Susceptibility of cancer cells to β-lapachone is enhanced by ionizing radiation. Int J Radiation Oncol Biol Phys 2005; 61: 212–219
- Suzuki M, Amano M, Choi J, Park HJ, Williams B, Ono K, Song CW. Synergistic effects of radiation and B-lapachone in DU-145 human prostate cancer cells in vitro. Radiation Res 2006; 165: 525–531
- Choi EK, Terai K, Ji IM, Kook YH, Park KH, Oh ET, Griffin RJ, Lim BU, Kim JS, Lee DS, et al. Upregulation of NAD(P)H: quinone oxidoreductase (NQO1) by radiation potentiates the effect of bioreductive β-lapachone against cancer cells. Neoplasia 2007; 9: 634–642
- Park HJ, Choi EK, Choi J, Ahn K, Kim EJ, Ji IM, Kook YH, Ahn S, Williams B, Griffin RJ, et al. Heat-induced up-regulation of NAD(P)H:quinone oxidoreductase potentiates anticancer effects of β-lapachone. Clin Cancer Res 2005; 11: 8866–8871
- Gutierrez PL. The role of NAD(P)H oxidoreductase (DT-diaphorase) in the bioactivation of quinine-containing antitumor agents: A review. Free Radic Biol Med 2000; 29: 263–275
- Pan SS, Forrest GL, Akman SA, Hu LT. NAD(P)H: quinone oxidoreductase expression and mitomycin C resistance developed by human colon cancer HCT116 cells. Cancer Res 1995; 55: 330–335
- Begleiter A, Fourie J. Induction of NQO1 in cancer cells. Methods in Enzymol 2004; 382: 321–351
- Rauth AM, Goldberg Z, Mirsa V. DT-diaphorase: Possible roles in cancer chemotherapy and carcinogenesis. Oncol Res 1997; 9: 339–349
- Ross D, Siegel D. NAD(P)H:quinone oxidoreductase 1 (NQO1, DT-diaphorase), functions and pharmacogenetics. Methods in Enzymol 2004; 382: 115–144
- Siegel D, Beall H, Senekowitach C, Kasai M, Arai H, Gibson NW, Ross D. Bioreductive activation of mitomycin C by DT-diaphorease. Biol Chem 1992; 31: 7879–7885
- Loadman PM, Bibby MC, Phillips RM. Pharmacological approach towards used on the clinically inactive agent EO9. The development of indolequinone bioreductive drugs. Br J Pharmacol 2002; 137: 701–709
- Winski SL, Swann E, Hargreaves RH, Dehn DL, Butler J, Moody CJ, Ross D. Relationship between NAD(P)H:quinone oxidoreductase 1 (NQO1) levels in a series of stably transfected cell lines and susceptibility to antitumor quinones. Biochem Pharmacol 2001; 61: 1509–1516
- Fitzsimmons SA, Workman P, Rever M, Paull K, Camalier R, Lewis AD. Reductase enzyme expression across the National Cancer Institute tumor cell line panel: Correlation with sensitivity to mitomycin C and EO9. J Natl Cancer Inst 1996; 88: 259–269
- Begleiter A. Clinical applications of quinone-containing alkylating agents. Front Bioscience 2000; 5: E153–171
- Guo W, Reigan P, Siegel D, Zirrolli J, Gustafson D, Ross D. Formation of 17-allyaminodemethoxygeldanamycin (17-AAG) hydroquinone by NAD(P)H:quinone oxidoreductase 1: Role of 17-AAG hydroquinone in heat shock protein 90 inhibition. Cancer Res 2005; 65: 10006–10015
- Sharp SY, Kelland LR, Valenti MR, Brunton MA, Hobbs S, Workman P. Establishment of an isogenic human colon tumor model for NQO1 gene expression: Application to investigate the role of DT-diaphorase in bioredcutive drug activation in vitro and in vivo. Mol Pharmacol 2000; 58: 1146–1155
- Marin A, Lopez de Cerain A, Hamilton E, Lewis AD, Martinez-Penuela JM, Idoate MA, Bello J. DT-diaphorase and cytochrome B5 reductase in human lung and breast tumors. Br J Cancer 1997; 76: 923–929
- Siegel D, Franklin WA, Ross D. Immunohistochemical detection of NAD(P)H:quinone oxidoreductase in human lung and lung tumors. Clin Cancer Res 1998; 4: 2065–2070
- Belinsky M, Jaiswal AK. NAD(P)H:quinone oxidoreductase 1 (DT-diaphorase) expression in normal and tumor tissues. Cancer Metastasis Rev 1993; 12: 103–117
- Cresteil T, Jaiswal AK. High levels of expression of the NAD(P)H:quinone oxidoreductase (NQO1) gene in tumor cells compared to normal cells of the same origin. Biochem Pharmacol 1991; 42: 1021–1027
- Beall HD, Murphy AM, Siegel D, Hargreaves RH, Butler J, Ross D. NAD(P)H:quinone oxidoreductase (DT-diaphorase) as a target for bioreductive antitumor quinones: Quinone cytotoxicity and selectivity in human lung and breast cancer cell lines. Mol Pharmacol 1995; 48: 499–504
- Planchon SM, Pink JJ, Tagliarino C, BornmannWG, Varnes ME, Boothman DA. β-lapachone-induced apoptosis in human prostate cancer cells: Involvement of Nqo/xip3. Exp Cell Res 2001; 267: 95–106
- Pink JJ, Planchon SM, Tagliarino C, Varnes ME, Siegel D, Boothman DA. NAD(P)H: quinone oxidoreductase activity is the principal determinant of beta-lapachone cytotoxicity. J Biol Chem 2000; 275: 5416–5424
- Pink JJ, Wuerzberger-Davis S, Tagliarino C, Planchon SM, Yang X, Froelich CJ, Boothman DA. Activation of cysteine protease in MCF-7 and T47D breast cancer cells during β-lapachone mediated apoptosis. Exp Cell Res 2000; 255: 144–155
- Tagliarino C, Pink JJ, Dubyak GR, Nieminen AL, Boothman DA. Calcium is a key signaling molecule in beta-lapachone mediated cell death. J Biol Chem 2001; 276: 19150–19159
- Li Y, Sun X, LaMont JT, Pardee AB, Li CJ. Selective killing of cancer cells by β-lapachone: Direct checkpoint activation as a strategy against cancer. Proc Natl Acad Sci USA 2003; 100: 2674–2678
- Pardee AB, Li YZ, Li C. Cancer therapy with β-lapachone. Current Cancer Drug Targets 2002; 2: 227–242
- Li CJ, Li YZ, Pinto AV, Pardee AB. Potent inhibition of tumor survival in vivo by beta-lapachone plus taxol: Xombining drugs imposes different artificial checkpoints. Proc Natl Acad Sci USA 1999; 96: 13369–13374
- Li CJ, Wang C, Pardee AB. Induction of apoptosis by beta-lapachon in human prostate cancer cells. Cancer Res 1995; 55: 3712–3715
- Huang L, Pardee AB. β-Lapachone induces cell cycle arrest and apoptosis in human colon cancer. Mol Med 1999; 5: 711–720
- Bentle MS, Reinicke KE, Dong Y, Bey EA, Bootheman DA. Nonhomologous end joining is essential for cellular resistance to the novel antitumor agent, B-lapachone. Cancer Res 2007; 67: 6936–6945
- Bey EA, Bentle MS, Reinicke KE, Dong Y, Yang CR, Girard L, Minna JD, Bornmann WG, Gao J, Boothman DA. An NQO1- and PARP1-1 mediated cell death pathway induced in non-small cell lung cancer cells by beta-lapachone. Proc Natl Acad Sci USA 2007; 1004: 11832–11837
- Boothman DA, Trask DK, Pardee AB. Inhibition of potentially lethal DNA damage repair in human tumor cells by beta-lapachone, an activator of topoisomerase. Cancer Res 1989; 49: 605–612
- Jaiswal AK. Regulation of genes encoding NAD(P)H:quinone oxidoreductases. Free Radic Biol Med 2000; 29: 254–262
- Dhakshinamoorthy S, Jain AK, Bloom DA, Jaiswal AK. Bach1 competes with Nrf2 leading to negative regulation of the antioxidant response element (ARE)-mediated NAD(P)H:quinone oxidoreductase 1 gene expression and induction in response to antioxidants. J Biol Chem 2005; 280: 16891–16900
- Chen S, Knox R, Lewis AD, Friedlos F, Workman P, Deng PS, Fung M, Ebenstein D, We K, Tsai TM. Catalytic properties of NAD(P)H:quinone acceptor oxidoreductase: Study involving mouse, rat, human, and mouse-rat chimeric enzyme. Mol Pharmacol 1995; 47: 934–939
- Chen S, Knox R, Wu K, Deng PS, Zhou D, Bianchet MA, Amzel LM. Molecular basis of the catalytic differences among DT-diaphorase of human, rat, and mouse. J Bio Chem 1997; 272: 1437–1439
- Danson S, Ward TH, Butler J, Ranson M. DT-diaphorase: A target for new anticancer drugs. Cancer Treat Rev 2004; 30: 437–449
- Winski SL, Swann E, Hargreaves RH, Dehn DL, Butler J, Moody CJ, Ross D. Relationship between NAD(P)H:quinone oxidoreductase 1 (NQO1) levels in a series of stably transfected cell lines and susceptibility to antitumor quinones. Biochem Pharmacol 2002; 61: 1509–1516