Abstract
The creation of a functional blood supply from the normal tissue vasculature via the process of angiogenesis is critical for the continued growth and development of solid tumours. This importance has led to the concept of targeting the tumour vasculature as a therapeutic strategy, and two major types of vascular targeting agents (VTAs) have developed; those that inhibit the angiogenic process–angiogenesis inhibiting agents (AIAs)–and those that specifically damage the already established neovasculature–vascular disrupting agents (VDAs). The tumour vasculature also plays a critical role in influencing the response to hyperthermia. Generally, the poorer the blood supply the better the heat effect, thus combining VTAs with heat would appear to be a logical approach. Numerous pre-clinical studies have now demonstrated the benefits of combining AIAs or VDAs with heat to improve tumour response. VDAs have also been shown to enhance thermoradiosensitisation, especially at mild hyperthermia temperatures. Although some enhancement of normal tissue response has been observed when VDAs are given with heat, the effects are always less than those seen in tumours, and no increased normal tissue reactions are seen when radiation, VDAs and heat are combined, thus a therapeutic benefit exists. These pre-clinical studies clearly encourage clinical trials with these combinations.
Introduction
The growth and development of most solid tumours requires that they develop their own functional blood supply Citation[1], Citation[2] which they do from the already established normal tissue vasculature by the process of angiogenesis Citation[3], Citation[4]. This tumour neovasculature is not only critical for survival of the tumour cells it is also probably one of the most important factors influencing the therapeutic application of hyperthermia. The vasculature is known to be a target for hyperthermia damage Citation[5], Citation[6]. In addition, blood flow is one of the major means by which heat is dissipated from tissues, and thus influences the ability to heat tumours; generally, the lower the rate of blood flow the easier it is to heat Citation[7], Citation[8]. The tumour vasculature also controls the microenvironment within tumours Citation[9], Citation[10] and this plays an important role in determining the response of the tumour cells to heat. Typically, the tumour neovasculature is very different from the normal vessels from which it arises, being both structurally and functionally abnormal Citation[9], Citation[10]. As a result, it is unable to meet all the oxygen and nutrient demands of the growing tumour mass and areas develop within the tumour that are oxygen deprived, nutrient deficient, and highly acidic Citation[9], Citation[10]. Cells existing in such conditions are more sensitive to the cytotoxic action of heat Citation[11], Citation[12].
In recent years, the concept of specifically targeting tumour vasculature has developed as a therapeutic approach. This can be achieved either by inhibiting the angiogenic process or damaging the already established tumour vessels. Both approaches have undergone extensive pre-clinical testing and many of these vascular targeting agents (VTAs) are now in clinical evaluation Citation[13], Citation[14]. Additional studies have demonstrated that physiological modification of tumour blood flow, either by simply clamping Citation[15], Citation[16], or physiological agents Citation[17–19], can significantly increase tumour sensitivity to heat. This suggests that the anti-vascular effects of VTAs may make them promising new candidates for improving the efficacy of hyperthermia.
Angiogenesis and vascular targeting approaches
Angiogenesis is the formation of new blood vessels from pre-existing vessels. In adults, it is normally only associated with certain physiological processes including the female reproductive cycle, pregnancy, muscular hypertrophy and wound healing Citation[20]. However, it is seen with a number of pathological conditions, such as rheumatoid arthritis, diabetes, macular degeneration, psoriasis, cardiovascular disease and cancer Citation[20]. The need for angiogenesis in the successful growth and development of most solid tumours is known Citation[1], Citation[2], but the actual triggers that initiate this process are not fully established. Hypoxia is clearly one factor that plays a significant role Citation[21], but other ‘non-hypoxia factors’ have also been implicated including loss of tumour suppressor gene function and oncogene activation Citation[21–23]. Whatever the trigger, the angiogenic process begins with the release of angiogenic factors, primarily vascular endothelial growth factor (VEGF), by the tumour cells Citation[23]. These factors then set in motion a number of physical steps that include enzymatic destruction of the basal membrane by the endothelial cells, migration of endothelial cells into the extracellular matrix to form sprouts, and endothelial cell division away from the sprout tip Citation[4]. Solid strands of endothelial cells are then formed in the extracellular matrix, lumen develops within those strands, neighbouring sprouts fuse to form loops, and from the primary loops new buds and sprouts emerge Citation[4]. Functional vessels are finally established ().
Figure 1. Schematic illustration of how the growth of most solid tumours is dependent on the development of its own neovasculature from the normal tissue vessels by the process of angiogenesis. Also shown is how vascular targeting agents (VTAs) can be used as a therapeutic approach against the tumour vasculature. They include AIAs (angiogenesis inhibiting agents) and VDAs (vascular disrupting agents), which although similar are distinctly different in their mode of action.
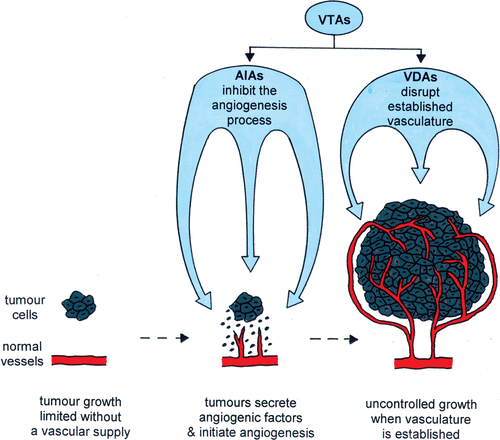
The importance of the tumour neovasculature makes it a potential target for therapy and two major approaches have emerged. One is based on controlling the blood vessel development by inhibiting the angiogenesis process (angiogenesis inhibiting agents; AIAs), and the other involves compromising the function of the already existing blood vessels (vascular disrupting agents; VDAs). Although AIAs and VDAs both target the tumour vascular supply they are two distinct approaches Citation[24], Citation[25]. AIAs are designed to prevent further development of the tumour neovascular network. As such they are likely to be given as a chronic administration and are probably best suited for early stage or metastatic disease. VDAs are agents that cause direct damage to the already established tumour endothelium. Thus, their administration is most likely to be of a more acute type to induce substantial vascular shutdown. There is also good evidence that VDAs have a superior effect on bulky disease. Numerous AIAs capable of inhibiting new blood vessel formation have been identified, and each affects at least one of the several important stages of angiogenesis. The primary targets are the angiogenic factors because they play the most significant role in neo-vascularisation Citation[23]. Of these angiogenic factors, the most potent and specific is VEGF, which is not only crucial for endothelial cell proliferation and blood vessel formation, but also induces significant vascular permeability and plays a key role in endothelial cell survival signalling in newly formed vessels Citation[21], Citation[23]. VEGF has been targeted by a variety of strategies Citation[25–28], including monoclonal antibodies, e.g., bevacizumab/avastin and DC101, inhibitors of endothelial cell receptor-associated tyrosine kinase activity, e.g., SU5474, SU6668, ZD6474 and PTK787/ZK 222584, and antisense. Other approaches, including those targeting basement membrane degradation, endothelial cell migration, endothelial cell proliferation, and tube formation, have also been actively considered Citation[26–28]. Many of these anti-angiogenic therapies are currently under clinical evaluation Citation[13], Citation[26–28].
VDAs include physical treatments like hyperthermia or photodynamic therapy, which have been well documented to induce direct tumour cell killing and an indirect effect through the induction of vascular damage Citation[5], Citation[6]. They also include biological response modifiers or cytokines like tumour necrosis factor (TNF) and interleukins; certain established chemotherapeutic drugs such as vinka alkaloids and arsenic trioxide (ATO); and various ligand-based approaches that use antibodies, peptides or growth factors that can selectively bind to tumour vessels Citation[5], Citation[29–31]. But, more commonly VDAs involve the use of small molecule drugs Citation[31], of which there are two major classes of agents. The first includes flavone acetic acid (FAA) and its derivative 5,6-dimethylxanthenone-4-acetic acid (DMXAA), which have a complex mechanism of action that is poorly understood, but their main effect on vascular endothelial cells is thought to involve a cascade of direct and indirect effects, the latter involving the induction of cytokines, especially TNF-α, leading to the induction of haemorrhagic necrosis Citation[32]. A second group includes the tubulin-binding agents combretastatin A-4 disodium phosphate (CA4P), ZD6126, AVE8062, NPI2358, MN-029, and OXi 4503 Citation[31], Citation[32]. These tubulin depolymerising agents are primarily believed to selectively disrupt the cytoskeleton of proliferating endothelial cells, resulting in endothelial cell shape changes and subsequent thrombus formation and vascular collapse Citation[32]. Since they preferentially target dividing endothelial cells this accounts for their tumour specificity. Both types of small molecular drugs have been shown to have potent anti-vascular and anti-tumour efficacy in a wide variety of preclinical models and the lead agents are undergoing clinical evaluation Citation[14]. Whether AIAs or VDAs are given alone or in combination, the effect on tumours generally does not lead to tumour regression. Thus, their potential benefit is likely to be when combined with other therapies such as radiation, chemotherapy or hyperthermia.
VTAs and hyperthermia
A limited number of studies have investigated the combination of AIAs with hyperthermia (). One of the first agents to be tested was TNP-470, the synthetic analogue of fumagillin first isolated from Aspergillus fumigatus, a potent inhibitor of tumour angiogenesis Citation[33]. Repeated administration of TNP-470 to mice bearing murine or human tumour xenografts significantly inhibited tumour growth Citation[34], Citation[35] and such a schedule given after heating tumours further significantly enhanced the heat response in those same studies. However, this effect was temperature dependent, being observed at 43° and 44°C, but not at 42°C Citation[34], Citation[35]. This enhancement was attributed to TNP-470 inhibiting angiogenesis that occurred following heat-induced vascular damage, the latter effect being temperature dependent. Similar studies in a BT4An rat glioma model using batimastat, a broad spectrum inhibitor of metalloproteinases Citation[36], failed to show any enhancement of heat damage using a temperature of 44°C that produced substantial vascular damage Citation[37]. This lack of effect could be attributed to the fact that batimastat alone at the dose and schedule used in this study, was ineffective at inhibiting growth in this tumour model. Clearly, AIAs can be used to improve the heat response of tumours, but do so via mechanisms that do not involve any improvement in tumour heating, nor tumour sensitisation to heat resulting from any AIA-induced microenvironmental changes. All that is required is that the heat treatment is sufficient to induce vascular damage and that the AIAs are effective as angiogenesis inhibitors. With the large number of AIAs currently in clinical testing as potential anti-cancer agents Citation[13] it is surprising that there are so few studies combining them with hyperthermia.
Table I. Preclinical studies combining VTAs and hyperthermia.
This is not the situation with VDAs and many different agents have been combined with hyperthermia, as shown in . The majority of these studies have involved the combination of photodynamic therapy (PDT) and heat. PDT involves the administration of a photosensitising dye with subsequent activation by visible light Citation[38], Citation[39]. Tumour cell killing by PDT in vivo has been suggested to be the result of both a direct effect on tumour cells Citation[40], Citation[41] as well as a result of damage to tumour vasculature Citation[42–44]. When combined with hyperthermia, different schedules have been used, including simultaneous treatments Citation[45–47], the PDT given immediately before or after the heat Citation[48–50], or with a short 30-minute Citation[51] or longer 4-hour Citation[52] time interval. Of the four studies in which different schedules were compared Citation[48–50], Citation[52], only two found the PDT before heat to be superior Citation[49], Citation[52]. Furthermore, several studies have shown that PDT and heat are an effective combination in vitro, especially when the PDT is administered prior to heating Citation[53], Citation[54]. Thus the role of the vascular damaging component of PDT in the sensitisation of tumours to heat remains unclear.
Hyperthermia has also been combined with the cytokine TNF, and various drugs including ATO, vinblastine, FAA, DMXAA and CA4P (). With all these vascular disrupting drugs there was an enhancement of the heat response and this effect was time and schedule dependent, with the maximum response generally observed if the heating was started 1–6 hours after VDA injection Citation[55–63] and this clearly corresponded to the time of maximal reduction in tumour blood flow in those studies. For the TNF studies Citation[64], Citation[65] the heat was only administered 3–4 hours after injecting the VDA, but at this time the decrease in tumour blood flow was substantial. With vinblastine the decrease in tumour blood flow took almost 12 hours to reach a nadir, yet the maximal enhancement of heat response occurred much earlier Citation[62]. Injecting VDAs after heating has been shown to be substantially less effective at enhancing the heat response Citation[55], Citation[56], Citation[60], Citation[61] and in most cases did not appear to be greater than a simple additive effect of the VDA and heat alone.
Several studies have investigated the temperature dependency for the enhancement of hyperthermia by VDAs. Using CA4P in a C3H mammary carcinoma Citation[61], Citation[71] or ATO in the SCK tumour Citation[59], Citation[63] these enhancements of tumour response were generally independent of heating temperatures between 40.5° to 42.5°C. Moreover, the effects obtained with CA4P were very similar to those found in the same tumour model when heated after injecting hydralazine, a transient physiological modifier of blood flow Citation[17]. Interestingly, although measurements of tumour perfusion after treatment with CA4P Citation[72] or ATO Citation[59] were prolonged when compared to hydralazine Citation[17] they were still somewhat transient with substantial recovery within 24 hours. FAA and DMXAA induce a maximum reduction in perfusion in a C3H mammary carcinoma Citation[57], Citation[72] that is actually maintained for 24 hours or more. With these VDAs the enhanced heat response at 42.5°C is similar to CA4P, but at temperatures below 42.5°C the enhancements are greater Citation[57], Citation[60], Citation[71], Citation[73]. Additional studies with hydralazine demonstrated that the reduction in blood flow leads to both a better tumour heating Citation[17], Citation[66], Citation[67] and an increase in tumour damage Citation[17], Citation[67], Citation[68], the latter effect presumably occurring because the reduction in tumour blood flow results in an increase in tumour hypoxia and associated tumour acidosis, and several in vitro studies have shown that prolonged oxygen deprivation of cells will increase their sensitivity to heat, an effect which is due to hypoxia-induced metabolic perturbations (leading to a decrease in pH) rather than hypoxia per se Citation[11], Citation[12]. With the VDAs there is evidence that similar mechanisms are operating, since studies with CA4P, FAA and DMXAA have shown both an improved tumour heating Citation[56], Citation[69] and a decrease in tumour pH Citation[56], Citation[70]. However, the greater enhancements seen with FAA and DMXAA at mild hyperthermia temperatures suggest that factors unrelated to a simple reduction in tumour blood flow are involved. Exactly what these factors are is not clear, although they may simply reflect increased cell killing as a result of the prolonged reduction in tumour blood perfusion.
Enhancing tumour response to heat is of no therapeutic benefit if the same effect occurs in normal tissues. Unfortunately, this important aspect has not been investigated with AIAs and only to a small degree with VDAs. Studies using rats indicated that the uptake of the photosensitiser was greater in a DS-carcinosarcoma than various normal tissues and this would be expected to reduce the effect of PDT in those normal tissues, as well as any enhancement of heat damage Citation[46]. Indeed, in an additional study in which cortical lesions in rat brains were measured following PDT treatment, absolutely no increase was seen with heat, albeit using a mild temperature of 41°C Citation[74]. Eikesdal and colleagues reported on the incidence of lethality and the development of oedema in heat treated feet of rats given CA4P and 44°C heating Citation[58], Citation[62]. Lethality was not influenced by the combination. A moderate increase in oedema was seen within 1–2 days after treatment, but this had fully recovered by day 5. Results from additional experiments into the development of skin damage in the normal feet of mice Citation[57], Citation[60], Citation[61] demonstrated a significant increase in heat damage by VDAs. Neither DMXAA nor CA4P caused any significant effect on blood perfusion of skin Citation[72], so this cannot explain the enhancement seen. Previous studies with FAA suggested that the increase in skin damage was the result of an FAA-induced increase in the concentration of TNF-α in the circulation Citation[57]. However, there was no apparent correlation between TNF-α production after DMXAA treatment and the enhanced skin response to heating Citation[60]. Furthermore, studies with CA4P failed to detect stimulation of TNF-α production Citation[75], yet it was still capable of enhancing heat response of skin Citation[61]. Although the reasons for the enhanced response of heat in normal skin remain to be elucidated, the most important finding is that in those studies in which skin reactions and tumour response were measured with the same drugs in the same mouse strains Citation[57], Citation[60], Citation[61], then the enhancement of the heat response in skin was always less than in tumours, thus combining VDAs with heat does lead to a therapeutic benefit.
Thermoradiotherapy and VTAs
The use of hyperthermia as a curative treatment of cancer in humans is only possible when it is combined with more conventional therapies Citation[76]. This is especially true for radiation, in which preclinical studies have demonstrated a sound rationale for the combination with heat Citation[77] and significant benefit reported in a number of randomised clinical trials Citation[77]. VTAs have also been shown to be effective at enhancing radiation response in animal tumours Citation[25], so the combination of thermoradiotherapy with VTAs seems logical. Additional support for such a triple combination regime comes from the findings that the tumour vasculature plays an important role in the ability of heat to enhance radiation response Citation[77]. Very few studies have actually investigated the combination of radiation, heat and VTAs Citation[25]. None have been done with AIAs, and using VDAs only a limited number of agents have been used.
When combining VDAs with thermoradiotherapy, timing and sequencing of the drug, radiation and heat treatment will be critical to the response obtained. For radiation and heat the greatest anti-tumour effect is obtained when these treatments are given simultaneously, which is primarily because the heat acts as a radiation sensitiser Citation[77]. However, an identical effect is also seen in normal tissues, thus no therapeutic benefit results. When radiation is administered prior to heating in a sequential schedule an enhancement is observed, but since it only results from the heat killing the hypoxic radiation resistant population the overall anti-tumour effect is less than with the simultaneous schedule. The advantage here is that no enhancement of normal tissue damage occurs, thus therapeutically a sequential schedule is superior. A sequential schedule fits very nicely with the application of the VDAs, because one would want to give the VDA prior to heating so that the heat is applied at a time when vascular shut-down is maximal, but with the VDA administered after the radiation treatment to avoid any possible induction of radiation resistant hypoxia. CA4P Citation[61], FAA Citation[73], and DMXAA Citation[78] have all been combined with radiation and heat using such a sequential schedule and the results obtained with DMXAA in a C3H mammary carcinoma using the most clinically relevant endpoint of local tumour control are illustrated in . In these studies, radiation alone resulted in a TCD50 dose (radiation dose necessary to control 50% of tumours) of 53Gy. For hyperthermia alone, one only begins to see any enhancement of radiation response at temperatures above 41.5°C and this effect gets larger as the temperature increases. With the VDA there is an effect of the drug alone on radiation response, but when combined with heat the radiation response is enhanced at temperatures as low as 40.5°C. In fact, at this temperature the enhancement is as good as one finds with 43°C alone. An even greater effect was found with the VDA and heat as the temperature increased to 41.5°C. However, this temperature appears to be optimal for the maximal enhancement, with no further sensitisation at 42.5°C. VDAs have been shown to have absolutely no effect on the thermoradiosensitisation response of normal skin using a temperature of 41.5°C Citation[61], Citation[78], thus the enhancement of tumour response at this temperature is a pure therapeutic benefit.
Figure 2. Effect of DMXAA and heating on the radiation response of a C3H mammary carcinoma. (Top panel) Tumours were locally irradiated with graded radiation doses and the percentage of mice in each treatment group showing local tumour control 90 days after treatment recorded. Mice were given radiation alone (○); local heating (41.5°C; 60 minutes) starting 4-hours after irradiation (•); DMXAA (20 mg/kg) injected intraperitoneally 1-hour after irradiating (△); or treated with radiation, DMXAA and heat (▴). Points are from an average of 13 mice/group. (Bottom panel) TCD50 values (with 95% confidence intervals) determined from data similar to that shown in the top panel for heat alone (○); or DMXAA with heat (•). Data modified from references 61 and 78.
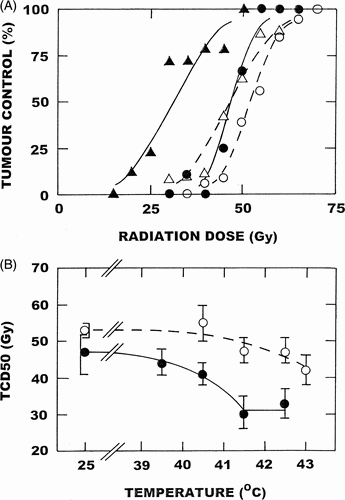
Conclusions and future aspects
The importance of the tumour vasculature makes it an excellent target for therapy. Numerous VTAs have been developed, many of which are in clinical testing, and the list of agents is continually expanding. However, when used as solitary treatments their overall effects on tumour growth are generally considered modest and so for their full potential to be realised they will probably have to be combined with other therapies. In this context, hyperthermia is an excellent choice because of the role that the tumour vasculature also plays in influencing the response to heat treatment.
Numerous preclinical studies have now demonstrated that combining VTAs with heat can be an effective approach for improving tumour response. This effect is clearly dependent on a number of factors including the heat temperature, the VTA dose, and the timing and scheduling between the two therapies. For AIAs the best effect is generally seen if the AIA follows heat, which could be the result of the AIA influencing the repair of cellular damage by heat or preventing angiogenesis occurring after heat-induced vascular damage. Whatever the mechanism the effect is clearly temperature dependent, only being observed at high temperatures, and appears to require that the dose of AIA is sufficient to have anti-tumour activity on its own. With the VDAs, the greatest enhancement is generally seen when the heat is applied at the time of maximal vascular shutdown by the VDA. There is no requirement that the VDA show anti-tumour activity alone and significant enhancements are seen at all hyperthermia temperatures, with the greatest enhancement actually occurring at mild temperatures. Limited normal tissue studies suggest tumour specificity.
Pre-clinical studies have extensively shown that radiation and hyperthermia are an effective combination. Since VTAs work with either heat or radiation, the combination of all three modalities should be beneficial. No such combinations have been investigated with AIAs. A few studies have looked at VDAs with radiation and heat, and substantial improvements in local tumour control reported. This is seen without any influence on normal tissue response, although this is only in early responding skin and clearly needs to be confirmed in more clinically relevant late responding normal tissues. Timing and scheduling are clearly critical issues for maximal benefit and would certainly need to be considered when clinically relevant fractionated schedules are employed. VDA dose and heating temperature do not appear to be so critical.
Clinical testing of a whole range of different VDAs is ongoing Citation[14] and many of these would be suitable candidates for combination with heat and radiation. In fact, several of the VDAs in clinical development are those that have been shown to be the most effective in preclinical studies. Hyperthermia has already undergone extensive clinical evaluation and a number of studies have demonstrated significant improvements in local control and survival when combined with radiation in specific tumour sites Citation[77]. Unfortunately, most studies show little benefit and this is most likely the result of a failure to adequately heat tumours to effective temperatures; while mild hyperthermia temperatures of around 40.5°–41.5°C are easier to obtain clinically, such temperatures are not very efficient at enhancing radiation damage and temperatures of around 42.5°–43°C are required Citation[77]. However, the preclinical data clearly demonstrates that when VDAs are included in the treatment schedule then the tumour response to a temperature of only 40.5°C was as good as that seen with a much higher temperature of 43°C in the absence of any VDA, and a far greater enhancement of radiation damage was seen with the VDAs when the temperature was increased to only 41.5°C. This suggests that if future clinical trials with hyperthermia and radiation were to include a VDA in the treatment regime, we may overcome the problem of ineffective heating of tumours and many more trials should be beneficial.
Acknowledgements
This review was made possible by financial support from the Danish Cancer Society.
References
- Brem S, Brem H, Folkman J, Finkelstein D, Patz A. Prolonged tumor dormancy by prevention of neovascularization in the vitreous. Cancer Res 1976; 36: 2807–2812
- Folkman J. How is blood vessel growth regulated in normal and neoplastic tissue?. Cancer Res 1986; 46: 467–473
- Hahnfeldt P, Panigrahy D, Folkman J, Hlatky L. Tumor development under angiogenic signaling: A dynamic theory of tumor growth, treatment response, and postvascular dormancy. Cancer Res 1999; 59: 4770–4775
- Bergers G, Benjamin LE. Tumorigenesis and the angiogenic switch. Nat Revs Cancer 2003; 3: 401–410
- Denekamp J, Hill S. Angiogenic attack as a therapeutic strategy for cancer. Radiother Oncol 1991; Suppl.20: 103–112
- Horsman MR. Tissue physiology and the response to heat. Int J Hyperthermia 2006; 22: 197–203
- Jain RK, Grantham FH, Gullino PM. Blood flow and heat transfer in Walker 256 mammary carcinoma. J Natl Cancer Inst 1979; 62: 927–933
- Patterson J, Strang R. The role of blood flow in hyperthermia. Int J Radiat Oncol Biol Phys 1979; 5: 235–241
- Vaupel P, Kallinowski F, Okunieff P. Blood flow, oxygen and nutrient supply, and metabolic micro-environment of human tumors: A review. Cancer Res 1989; 49: 6449–6465
- Vaupel P. Tumor microenvironmental physiology and its implications for radiation oncology. Semin Radiat Oncol 2004; 14: 198–206
- Overgaard J, Bichel P. The influence of hypoxia and acidity on the hyperthermic response of malignant cells in vitro. Radiol 1977; 123: 511–514
- Gerweck LE, Nygaard TG, Burlett M. Response of cells to hyperthermia under acute and chronic hypoxic conditions. Cancer Res 1979; 39: 966–972
- National Cancer Institute, Website: www.cancer.gov/clinicaltrials
- Patterson DM, Rustin GJS. Vascular damaging agents. Clin Oncol 2007; 19: 443–456
- Thrall DE, Gillette EL, Dewey WC. Effect of heat and ionising radiation on normal and neoplastic tissue of the C3H mouse. Radiat Res 1975; 63: 363–377
- Hill SA, Denekamp J. The effect of vascular occlusion on the thermal sensitization of a mouse tumor. Br J Radiol 1978; 51: 997–1002
- Horsman MR, Christensen KL, Overgaard J. Hydralazine-induced enhancement of hyperthermic damage in a C3H mammary carcinoma in vivo. Int J Hyperthermia 1989; 5: 123–136
- Prescott DM, Samulski TV, Dewhirst MW, Page RL, Thrall DE, Dodge RK, Oleson JR, et al. Use of nitroprusside to increase tissue temperature during local hyperthermia in normal and tumor-bearing dogs. Int J Radiat Oncol Biol Phys 1992; 23: 377–385
- Urano M, Montoya V, Booth A. Effect of hyperglycemia on the thermal response of murine normal and tumor tissue. Cancer Res 1983; 43: 453–455
- Carmeliet P. Angiogenesis in health and disease. Nature Med 2003; 9: 653–660
- Pugh CW, Ratcliffe PJ. Regulation of angiogenesis by hypoxia: Role of the HIF system. Nat Med 2003; 9: 677–684
- Moeller BJ, Cao Y, Vujaskovic Z, Li CY, Haroon ZA, Dewhirst MW. The relationship between hypoxia and angiogenesis. Sem Radiat Oncol 2004; 14: 215–221
- Ferrara N, Gerber HP, LeCouter J. The biology of VEGF and its receptors. Nat Med 2003; 9: 669–676
- Siemann DW, Bibby MC, Dark GG, Dicker AP, Eskens FA, Horsman MR, Marmé D, Lo Russo PM, et al. Differentiation and definition of vascular-targeted therapies. Clin Cancer Res 2005; 11: 416–420
- Horsman MR, Siemann DW. Pathophysiological effects of vascular-targeting agents and the implications for combination with conventional therapies. Cancer Res 2006; 66: 11520–11539
- Kerbel R, Folkman J. Clinical translation of angiogenesis inhibitors. Nat Rev Cancer 2002; 2: 727–739
- Marmé D. The impact of anti-angiogenic agents on cancer therapy. J Cancer Res Clin Oncol 2003; 129: 607–620
- Eskens FALM. Angiogenesis inhibitors in clinical development: Where are we now and where are we going?. Br J Cancer 2004; 90: 1–7
- Chaplin DJ, Dougherty GJ. Tumour vasculature as a target for cancer therapy. Br J Cancer 1999; 80(Suppl.1)57–64
- Thorpe PE. Vascular targeting agents as cancer therapeutics. Clin Cancer Res 2004; 10: 415–427
- Siemann DW, Chaplin DJ, Horsman MR. Vascular targeting therapies for treatment of malignant disease. Cancer 2004; 100: 2491–2499
- Tozer GM, Kanthou C, Baguley BC. Disrupting tumour blood vessels. Nat Rev Cancer 2005; 5: 423–435
- Ingber D, Fujita T, Kishimoto S, Sudo K, Kanamaru T, Brem H, Folkman J, et al. Synthetic analogues of fumagillin that inhibit angiogenesis and suppress tumour growth. Nature 1990; 348: 555–557
- Yano T, Tanase M, Watanabe A, Sawada H, Yamada Y, Shino Y, Nakano H, Ohnishi T, et al. Enhancement effect of an anti-angiogenic agent, TNP-470, on hyperthermia-induced growth suppression of human esophageal and gastric cancers transplantable to nude mice. Anticancer Res 1995; 15: 1355–1358
- Nishimura Y, Murata R, Hiraoka M. Combined effects of an angiogenesis inhibitor (TNP-470) and hyperthermia. Br J Cancer 1996; 73: 270–274
- Brown PD. Matrix metalloproteinase inhibitors: A new class of anticancer agents. Curr Opin Invest Drug 1993; 2: 617–626
- Eikesdal HP, Bjorkhaug ST, Dahl O. Hyperthermia exhibits anti-vascular activity in the BT4An rat glioma: Lack of interaction with the angiogenesis inhibitor batimastat. Int J Hyperthermia 2002; 18: 141–152
- Kessel D. Hematoporphyrin and HPD. Photophysics, photochemistry and phototherapy. Photochem Photobiol 1984; 39: 851–859
- Dougherty TJ. Photosensitizers: Therapy and detection of malignant tumors. Photochem Photobiol 1987; 45: 879–889
- Nelson JS, Wright WH, Berns MW. Histopathological comparison of the effects of hematoporphyrin derivative on two different murine tumors using computer-enhanced digital video fluorescence microscopy. Cancer Res 1985; 45: 5781–5786
- Winter J. Photodynamic therapy effect in an intraocular retinoblastoma-like tumour assessed by an in vitro colony forming assay. Br J Cancer 1989; 59: 869–872
- Selman SH, Kreimer-Birnbaum M, Klaunig JE, Goldblatt PJ, Keck RW, Britton SL. Blood flow in transplantable bladder tumors treated with hematoporphyrin derivative and light. Cancer Res 1984; 44: 1924–1927
- Star WM, Marijnissen HPA, van den Berg-Blok AE, Versteeg JAC, Franken KAP, Reinhold HS. Destruction of rat mammary tumor and normal tissue microcirculation by hematoporphyrin derivative photoradiation observed in vivo in sandwich observation chambers. Cancer Res 1986; 46: 2532–2540
- Horsman MR, Winther J. Vascular effects of photodynamic therapy in an intraocular retinoblastoma-like tumour. Acta Oncol 1989; 28: 693–697
- Orenstein A, Kostenich G, Kopolovic Y, Babushkina T, Malik Z. Enhancement of ALA-PDT damage by IR-induced hyperthermia on a colon carcinoma model. Photochem Photobiol 1999; 69: 703–707
- Kelleher DK, Bastian J, Thews O, Vaupel P. Enhanced effects of aminolaevulinic acid-based photodynamic therapy through local hyperthermia in rat tumours. Br J Cancer 2003; 89: 405–411
- Kelleher DK, Thews O, Scherz A, Salomon Y, Vaupel P. Combined hyperthermia and chlorophyll-based photodynamic therapy: Tumour growth and metabolic microenvironment. Br J Cancer 2003; 89: 2333–2339
- Waldow SM, Dougherty TJ. Interaction of hyperthermia and photoradiation therapy. Radiat Res 1984; 97: 380–385
- Henderson BW, Waldow SM, Potter WR, Dougherty TJ. Interaction of photodynamic therapy and hyperthermia: Tumor response and cell survival studies after treatment of mice in vivo. Cancer Res 1985; 45: 6071–6077
- Matsumoto N, Miyoshi N, Saito H, Fukuda M. Combination therapy of microwave hyperthermia and photodynamic therapy on squamous cell carcinoma in vivo. Hyperthermic Oncology, T Sugahara, M Saito. Taylor and Francis, London 1989; 1: 363–364
- Levendag PC, Marijnissen HPA, de Ru VJ, Versteeg JAC, van Rhoon GC, Star WM. Interaction of interstitial photodynamic therapy and interstitial hyperthermia in a rat rhabdomyosarcoma–A pilot study. Int J Radiat Oncol Biol Phys 1988; 14: 139–145
- Chen Q, Chen H, Shapiro H, Hetzel F. Sequencing of combined hyperthermia and photodynamic therapy. Radiat Res 1996; 146: 293–297
- Christensen T, Wahl A, Smedshammer L. Effects of haematoporphyrin derivative and light in combination with hyperthermia on cells in culture. Br J Cancer 1984; 50: 85–89
- Mang TS, Dougherty TJ. Time and sequence dependent influence of in vitro photodynamic therapy (PDT) survival by hyperthermia. Photochem Photobiol 1985; 42: 533–540
- Horsman MR, Chaplin DJ, Overgaard J. The effect of combining flavone acetic acid and hyperthermia on the growth of a C3H mammary carcinoma. Int J Radiat Biol 1991; 60: 385–388
- Sakaguchi Y, Maehara Y, Baba H, Kusumoto T, Sugimachi K, Newman RA. Flavone acetic acid increases the antitumor effect of hyperthermia in mice. Cancer Res 1992; 52: 3306–3309
- Horsman MR, Sampson LE, Chaplin DJ, Overgaard J. The in vivo interaction between flavone acetic acid and hyperthermia. Int J Hyperthermia 1996; 12: 779–789
- Eikesdal HP, Schem BC, Mella O, Dahl O. The new tubulin inhibitor combretastatin A-4 enhances thermal damage in the BT4An rat glioma. Int J Radiat Oncol Biol Phys 2000; 46: 645–652
- Griffin RJ, Lee SH, Rood KL, Stewart MJ, Lyons JC, Lew YS, Park H, Song CW, et al. Use of arsenic trioxide as an antivascular and thermosensitising agent in solid tumors. Neoplasia 2000; 2: 555–560
- Murata R, Overgaard J, Horsman MR. Potentiation of the anti-tumor effect of hyperthermia by combining with the vascular targeting agent 5,6-dimethylxanthenone-4-acetic acid. Int J Hyperthermia 2001; 17: 508–519
- Murata R, Overgaard J, Horsman MR. Combretastatin A-4 disodium phosphate: a vascular targeting agent that improves the anti-tumor effects of hyperthermia, radiation and mild thermoradiotherapy. Int J Radiat Oncol Biol Phys 2001; 51: 1018–1024
- Eikesdal HP, Bjerkvig R, Mella O, Dahl O. Combretastatin A-4 and hyperthermia: A patent combination for the treatment of solid tumors. Radiother Oncol 2001; 60: 147–154
- Griffin RJ, Monzen H, Williams BW, Park H, Lee SH, Song CW. Arsenic trioxide induces selective tumour vascular damage via oxidative stress and increases thermosensitivity of tumours. Int J Hyperthermia 2003; 19: 575–589
- Kallinowski F, Moehle R, Vaupel P. Substantial enhancement of tumor hyperthermic response by tumor necrosis factor. Hyperthermic Oncology, T Sugahara, M Saito. Taylor and Francis, London 1989; 1: 258–259
- Lin JC, Park HJ, Song CW. Combined treatment of IL-α and TNF-α potentiates the antitumour effect of hyperthermia. Int J Hyperthermia 1996; 12: 335–344
- Dewhirst MW, Prescott DM, Clegg S, Samulski TV, Page RL, Thrall DE, Leopold K, Rosner G, Acker JC, Oleson JR, et al. The use of hydralazine to manipulate tumour temperatures during hyperthermia. Int J Hyperthermia 1990; 6: 971–983
- Honess DJ, Hu DE, Bleehen NM. A study of the mechanism of hydralazine enhancement of thermal damage in the KHT tumour. Int J Hyperthermia 1991; 7: 667–679
- Kalmus J, Okunieff P, Vaupel P. Dose-dependent effects of hydralazine on microcirculatory function and hyperthermic response of murine FSaII tumors. Cancer Res 1990; 50: 15–19
- Eikesdal HP, Bjerkvig R, Dahl O. Vnblastine and hyperthermia target the neovasculature in BT4AN rat gliomas: Therapeutic implications of the vascular phenotype. Int J Radiat Oncol Biol Phys 2001; 51: 535–544
- Breidahl T, Nielsen FU, Stødkilde-Jørgensen H, Maxwell RJ, Horsman MR. The effects of the vascular disrupting agents combretastatin A-4 disodium phosphate, 5,6-dimethylxanthenone-4-acetic acid and ZD6126 in a murine tumour: A comparative assessment using MRI and MRS. Acta Oncol 2006; 1998; 45: 306–316
- Horsman MR, Murata R. Vascular targeting therapies and hyperthermia. Vascular-targeted therapies in oncology, DW Siemann. John Wiley and Sons, London 2006; 137–157
- Murata R, Overgaard J, Horsman MR. Comparative effects of combretastatin A-4 disodium phosphate and 5,6-dimethylxanthenone-4-acetic acid on blood perfusion in a murine tumour and normal tissues. Int J Radiat Biol 2001; 77: 195–204
- Horsman MR, Murata R, Overgaard J. Improving local tumor control by combining vascular targeting drugs, mild hyperthermia and radiation. Acta Oncol 2001; 40: 497–503
- Dereski M, Madigan L, Chopp M. The effect of hypothermia and hyperthermia on photodynamic therapy of normal brain: Experimental study. Neurosurgery 1995; 36: 141–146
- Chaplin DJ, Pettit GR, Parkins CS, Hill SA. Antivascular approaches to solid tumour therapy: Evaluation of tubulin binding agents. Br J Cancer 1996; 74(Suppl.XXVII)S86–S88
- Overgaard J. Rationale and problems in the design of clinical trials. Hyperthermic oncology, J Overgaard. Taylor and Francis, London 1985; 2: 325–338
- Horsman MR, Overgaard J. Hyperthermia: A potent enhancer of radiotherapy. Clin Oncol 2007; 19: 418–426
- Murata R, Horsman MR. Tumour-specific enhancement of thermoradiotherapy at mild temperatures by the vascular targeting agent 5,6-dimethylxanthenone-4-acetic acid. Int J Hyperthermia 2004; 20: 393–404