Abstract
Background and aim: It is reported that NF-κB is activated by chemotherapy in some cancer cell lines and NF-κB activation is one of the mechanisms by which tumors are induced to become resistant to chemotherapy. We reported that heat-treatment-induced heat shock protein 70 (Hsp70) could inhibit I-kappa-B kinase, resulting in the inhibition of NF-κB activation. Therefore, we speculated that activated NF-κB in a pancreatic cell line might be inhibited by heat treatment, resulting in the enhancement of gemcitabine-induced cytotoxicity.
Methods: We used the human pancreatic carcinoma cell lines AsPC-1 and MIAPaCa-2. Both cell lines were treated with various concentrations (0, 5, 10, 20, and 30 μM) of gemcitabine for 24 h. Heat treatment (43°C, 1 h) was performed at various times relative to gemcitabine treatment. The effect of gemcitabine and heat treatment on cell survival was determined by WST-8 assay. The status of NF-κB in carcinoma cells exposed to gemcitabine was investigated by electrophoretic mobility shift assay and immunocytochemistry. We analyzed apoptosis and necrosis in AsPC-1 and MIAPaCa-2 cells by flow cytometry. Furthermore, the levels of Hsp70, cyclin D1, caspase-3, and vascular endothelial growth factor in each treatment group were detected by western blotting.
Results: (1) Significant cytotoxicity was observed with gemcitabine. (2) Gemcitabine activated NF-κB binding activity in both cell lines. (3) Heat treatment inhibited the gemcitabine-induced activation of NF-κB. (4) Heat treatment enhanced the cytotoxicity of gemcitabine, especially when heat treatment was performed 24 h before gemcitabine was given. (5) The levels of Hsp70 were increased by heat treatment. Gemcitabine did not affect the protein level of Hsp70. The levels of pro-caspase-3 were decreased by heat treatment combined with gemcitabine.
Conclusions: Heat treatment inhibited gemcitabine-induced activation of NF-κB, resulting in the enhancement of the cytotoxicity of gemcitabine.
Introduction
The transcription factor NF-κB is constitutively activated in many human cancers and has been implicated in cancer cell proliferation, survival, angiogenesis, and chemo-resistance. Activation of NF-κB in cancer cells induces the expression of multiple genes, including those that affect cell proliferation, angiogenesis, and anti-apoptosis. Therefore, the blocking of NF-κB activation may enhance the anticancer effect of chemotherapy Citation1,Citation2. Thus, it is expected that the blocking of NF-κB could attenuate tumor growth and enhance the anti-tumor effect of chemotherapeutic compounds.
Gemcitabine, a chemotherapeutic agent with proven efficacy in the treatment of lung and pancreatic cancers, is a deoxycytidine nucleoside analogue that affects several enzymes involved in DNA synthesis and repair. Once transported into the cell, gemcitabine (dFdCyd) must be phosphorylated to become activated. The triphosphate form, dFdCTP, inhibits DNA synthesis or replication by the addition of dFdCMP into the new DNA strand Citation3,Citation4. Gemcitabine has been shown to cause cell cycle arrest in the S-phase leading to apoptosis Citation5,Citation6. Previous studies also showed that gemcitabine alone could activate NF-κB, resulting in reduced apoptosis, suggesting that NF-κB activation inhibits apoptosis Citation7,Citation8.
In this study, we examined the relevance of NF-κB in the resistance of pancreatic carcinoma cell lines against gemcitabine. We and other groups previously reported that heat-treatment-induced heat shock protein 70 (Hsp70) could inhibit I-kappa-B kinase (IKK), resulting in the inhibition of NF-κB activation Citation9,Citation10. Therefore, we speculated that the activated NF-κB in pancreatic cell lines might be inhibited by heat treatment, resulting in the enhancement of gemcitabine-induced cytotoxicity.
Materials and methods
Chemicals
Gemcitabine (2′-deoxy-2′-difluorodeoxycytidine) is a deoxycytidine analogue with structural and metabolic similarities to cytrabine. Gemcitabine was purchased from Eli Lilly (Tokyo, Japan). Other reagents were from Wako Pure Chemical Industries (Osaka, Japan).
Cell lines and cell culture
Human pancreatic carcinoma cell lines AsPC-1 and MIAPaCa-2 were obtained from the American Type Culture Collection (Rockville, MD). AsPC-1 and MIAPaCa-2 cells were grown in 75-cm2 cell culture flasks and maintained in RPMI 1640 medium supplemented with 10% fetal bovine serum (FBS) (Equitech-Bio, Kerrville, TX), L-glutamine, and penicillin/streptomycin (GIBCO) at 37°C in a humidified atmosphere containing 5% CO2.
After AsPC-1 and MIAPaCa-2 in 75-cm2 cell culture flasks were trypsinized, 5 × 104 cells/ml of each cell were grown in 10 cm2 cell culture flasks and maintained in RPMI 1640 with 10% FBS and left for 72 h in the incubator at 37°C. These cells were ready for the experiments.
AsPC-1 and MIAPaCa-2 cell cultures were treated with gemcitabine at a concentration of 0–30 μM for 24 h. After the treatment with gemcitabine, the cells were rinsed with phosphate-buffered saline (PBS) and left for 48 h in the incubator at 37°C. In another series of experiments, before or after exposure of cancer cells to gemcitabine, AsPC-1 and MIAAPaCa-2 cells were incubated in a temperature-controlled CO2 incubator at 43°C for 60 min. After the cells were pretreated with heat, they were exposed to passive cooling in a temperature-controlled CO2 incubator (37°C) before the following examinations.
Timing of heat treatment combined with gemcitabine
Heat treatment (43°C, 1 h) was combined with gemcitabine at various times. AsPC-1 and MIA PaCa-2 cells were treated with heat 24 h before the treatment with gemcitabine (30 μM), simultaneously with gemcitabine treatment, 24 h after gemcitabine treatment, and 48 h after gemcitabine treatment ().
Figure 1. Schematic representation of the experimental protocol described in the Materials and methods section. Group 1 was treated with gemcitabine (30 μM, 24 h), group 2 received heat treatment (43°C, 1 h) 24 h before treatment with gemcitabine, group 3 received heat treatment simultaneously with gemcitabine treatment, group 4 received heat treatment 24 h after the treatment with gemcitabine, and group 5 received heat treatment 48 h after the treatment with gemcitabine. After all treatments, cell viability was determined by a WST-8 assay, the numbers of cells were counted, and a qualitative analysis of cell death was performed by flow cytometry.
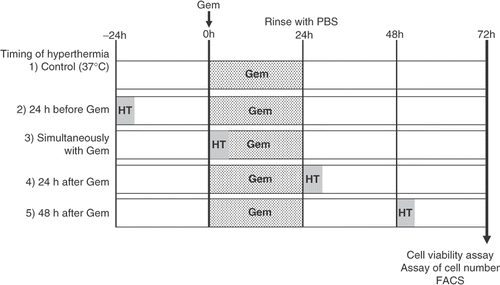
Cell viability assay
The effects of gemcitabine alone and heat treatment combinations with gemcitabine on cell viability were determined by WST-8 assay (Dojin Laboratory, Kumamoto, Japan) according to the manufacturer's instructions. Briefly, WST-8 solution was added to cells in 96-well plates, the cells were incubated at 37°C for 60–90 min, and the optical density of each well was read at 450 nm using a microplate reader (MPR-A4i, Tosoh Corporation, Tokyo, Japan).
Assay of cell number
AsPC-1 cells at 105 cells/ml were placed in 35-mm cell culture dishes and treated with gemcitabine and/or heat treatment. After 24 h of gemcitabine treatment, cells were rinsed once with PBS and removed by trypsin. The number of cells in each group was counted by a cell counter (Z2™ Coulter Counter® Cell and Particle Counter, Beckman Coulter, Fullerton, CA).
Flow cytometric analysis of apoptosis and necrosis
A qualitative analysis of cell death was performed by flow cytometry. AsPC-1 and MIA PaCa-2 cells were cultured in 35-mm cell culture dishes. After heat treatment combined with gemcitabine, medium was removed and each cell culture was washed twice with PBS, collected by centrifugation, and the pellets were resuspended in PBS. Apoptotic or necrotic cells were detected by annexin V-propidium iodide (PI). Analysis was performed using a fluorescence-activated cell sorting (FACS) system (FACS Calibur; Becton Dickinson, Franklin Lakes, NJ).
Electrophoretic mobility gel shift assay for nuclear transcription factors
AsPC-1 and MIA PaCa-2 cells were cultured in 35-mm cell culture dishes. After treatment with gemcitabine alone for 3–24 h, medium was removed and cells were washed twice with PBS. Nuclear protein from both cell lines was extracted as follows. The total nuclear protein of 15 μg was incubated with 1.0 pmol of double-stranded [32P] ATP end-labeled oligonucleotides containing consensus binding sequences for NF-κB (sense strand 5-AGGGACTTTCCGCTGGGGACTTTCC-3) in binding buffer (10 mM Hepes, pH 7.9, 80 mM NaCl, 3 mM MgCl2, 0.1 mM EDTA, 1 mM DTT, 1 mM PMSF, and 10% glycerol) for 30 min. After electrophoresis under non-denaturing conditions (0.5× TBE buffer), gels were dried and radioactive bands were visualized on X-ray films. Moreover, AsPC-1 cells were exposed to four kinds of treatment (untreated, gemcitabine alone, heat treatment alone, and heat treatment combined with gemcitabine). In this series of experiments, heat treatment was performed 24 h before the treatment of gemcitabine. The nuclear protein of AsPC-1 cells in each group was extracted after 24 h of gemcitabine treatment and the binding activity of NF-κB was measured by electrophoretic mobility shift assay (EMSA).
Immunocytochemical staining of nuclear transcription factors
AsPC-1 cells were cultured in 8-well chambered glass slides (Lab-Tek, Rochester, NY). After treatment with gemcitabine alone, heat treatment alone, and heat treatment combined with gemcitabine, medium was removed and AsPC-1 cells were washed once with PBS containing 1% bovine serum albumin. AsPC-1 cells were then incubated with rabbit polyclonal antibody directed against the NF-κB subunit RelA (p65) (Santa Cruz Biotechnology, Santa Cruz, CA) for 2 h at 37°C. Subsequently, the cells were incubated with fluorescein isothiocyanate-conjugated goat-anti-rabbit IgG (Biochemical Technologies, Stoughton, MA) for 2 h at room temperature and NF-κB staining was observed using an IX70-23FL/DIC-SP inverted fluorescence microscope (Olympus, Tokyo, Japan). Photographic images were taken from random fields.
Western blotting
AsPC-1 and MIAPaCa-2 cells were treated with heat treatment (43°C, 1 h) 24 h before the treatment with gemcitabine (30 μM) for 24 h. Moreover, cells were further incubated at 37°C for 24 h. At the end of this incubation, cells were washed twice with ice-cold PBS and solubilized. The incubated cells were lysed and equal quantities of protein (25–50 μg) were separated by 12% sodium dodecyl sulphate-polyacrylamide gel electrophoresis (SDS-PAGE) and electroblotted on to nitrocellulose membranes (Bio-Rad Laboratories, Hercules, CA). Membranes were incubated overnight at 4°C with primary antibodies against Hsp70 (Stressgen Bioreagents, Ann Arbor, MI), caspase-3, cyclinD1, vascular endothelial growth factor (VEGF), and Bcl-xL (Santa Cruz Biotechnology), then washed and incubated with the appropriate horseradish peroxidase-conjugated secondary antibody for 1 h at room temperature. Immune complexes were visualized by western blotting analysis using a commercial kit (ECL, Amersham, UK) according to the manufacturer's recommendations.
Statistical analysis
Results are presented as means ± SEM. Data were compared using one-way analysis of variance (ANOVA) and differences were considered significant if the P value was less than 0.05 according to the protected least significant difference test or Scheffe's F multiple comparison test. All analyses were performed using the Stat View 4.11-J software program (Abacus Concepts, Berkeley, CA) on a computer with a Windows operating system.
Results
Cytotoxic effect of gemcitabine alone on human pancreatic carcinoma cell lines
To determine the cytotoxic effects of gemcitabine against human pancreatic cancer cells, AsPC-1 and MIAPaCa-2 cell lines were treated with various concentrations (0, 5, 10, 20, and 30 μM) of gemcitabine for 24 h. After treatment, these cells were rinsed and left for 48 h in the incubator at 37°C. The effects of gemcitabine on cell viability were determined by an MTT-based WST-8 assay kit. Gemcitabine significantly inhibited the growth of AsPC-1 cells at doses of 10–30 μM () and the growth of MIAPaCa-2 cells in a dose-dependent manner (). According to these results, we used gemcitabine at a concentration of 30 μM for the following experiments.
Figure 2. Cytotoxic effect of gemcitabine in pancreatic carcinoma cells. AsPC-1 and MIAPaCa-2 cells were treated with various concentrations (0–30 μM) of gemcitabine for 24 h. After treatment, cells were rinsed with phosphate-buffered saline and left for 48 h in the incubator at 37°C. Cell viability was measured by an MTT-based WST-8 assay. Values represent mean ± SEM of triplicate samples of a representative experiment; similar results were obtained in three independent experiments. *P < 0.05, compared with the control in AsPC-1 cells (a). **P < 0.0001, compared with the control in MIAPaCa-2 cells (b).
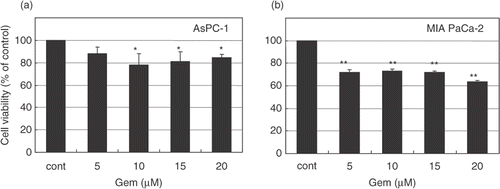
Cytotoxic effect of heat treatment combined with gemcitabine
To determine the optimal timing of heat treatment, we scheduled the four combinations of treatment as follows. (1) The heat treatment was performed 24 h before gemcitabine treatment. (2) The heat treatment was performed simultaneously with gemcitabine treatment. (3) The heat treatment was performed 24 h after gemcitabine treatment. (4) The heat treatment was performed 48 h after gemcitabine treatment. The effects of heat treatment combined with gemcitabine on cell viability were determined by an MTT-based WST-8 assay kit. Heat treatment enhanced the cytotoxicity of gemcitabine, especially when heat treatment was performed 24 h before the treatment with gemcitabine ().
Figure 3. Heat treatment enhanced the cytotoxicity of gemcitabine. AsPC-1 and MIAPaCa-2 cells received heat treatment in combination with gemcitabine treatment (30 μM) at various relative times. Cell viability was measured by an MTT-based WST-8 assay. Values represent mean ± SEM of four samples of a representative experiment; similar results were obtained in three independent experiments. *P < 0.0001, compared with the control in AsPC-1 cells (a). **P < 0.005, compared with the control in MIAPaCa-2 cells (b).
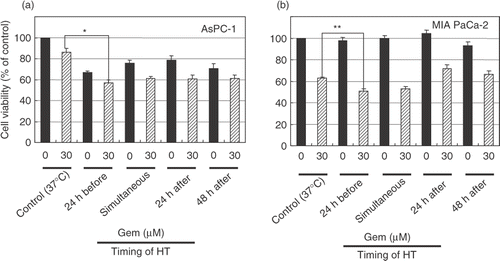
Cytometric analysis of cell death pattern
Cell death was quantified by flow cytometry using annexin-V and propidium iodide. AsPC-1 cells were treated with heat at four different times. Heat treatment without gemcitabine did not induce apoptosis and necrosis in each cell line. On the other hand, 30 µM of gemcitabine increased the ratio of apoptotic cell death from 1.5% to 3.1%. Moreover, the ratio of apoptotic cell death increased to 4.8% when heat treatment was performed before the treatment of gemcitabine. In the case of MIAPaCa-2 cells, heat treatment performed 24 h before the treatment with gemcitabine also enhanced apoptosis ().
Figure 4. Apoptosis and necrosis induced by heat treatment with gemcitabine treatment. Heat treatment enhanced cell death in pancreatic carcinoma cells. The cell death pattern for AsPC-1 and MIAPaCa-2 cell lines was assessed by qualitative flow cytometry using Annexin-V and propidium iodide. The number in the lower left indicates living cells, upper left indicates propidium iodide-positive, lower right indicates Annexin V-positive, and upper right indicates both Annexin V- and propidium iodide-positive. (a) AsPC-1 cells were treated with heat 24 h before the treatment with gemcitabine at various relative times and the resulting apoptotic cell death patterns are shown. This experiment was performed twice with similar results. (b) In the case of MIAPaCa-2 cells, heat treatment performed 24 h before gemcitabine treatment also enhanced apoptosis. This experiment was performed twice with similar results.
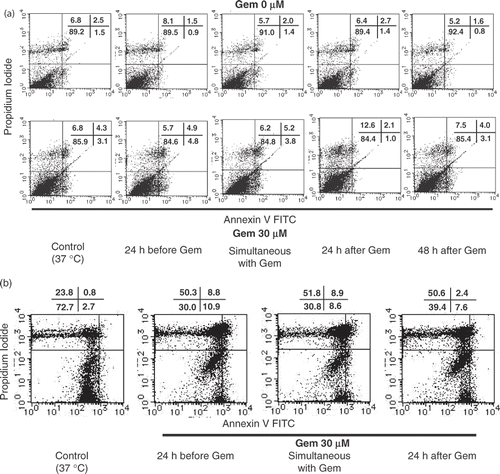
Effects of gemcitabine on NF-B activity
AsPC-1 and MIAPaCa-2 cells were exposed to 10 μM of gemcitabine for 3–24 h. At the indicated time, nuclear extracts were prepared and EMSA for NF-κB binding activity was performed. In AsPC-1 cells, gemcitabine increased NF-κB binding activity between 12 and 24 h (). On the other hand, in MIAPaCa-2 cells, gemcitabine increased NF-κB binding activity as early as 3 h (). Moreover, from the results of cytotoxicity in the WST-8 assay (), we determined the optimal timing of heat treatment combined with gemcitabine treatment. When AsPC-1 cells were exposed to various stimuli (no treatment, gemcitabine alone, heat treatment alone, and heat treatment combined with gemcitabine treatment), gemcitabine alone increased NF-κB binding activity in these cells and this effect was decreased by heat treatment ().
Figure 5. The effect of gemcitabine on NF-κB activation was studied by electrophoretic mobility shift assay. AsPC-1 and MIAPaCa-2 cells were exposed to 30 μM gemcitabine for various periods. At the indicated time, nuclear extracts were prepared and electrophoretic mobility shift assays for NF-κB binding activity were performed. Gemcitabine increased NF-κB binding activity in both cell lines. In AsPC-1 cells, gemcitabine increased NF-κB binding activity between 12 and 24 h. On the other hand, in MIAPaCa-2 cells, gemcitabine increased NF-κB binding activity as early as 3 h.
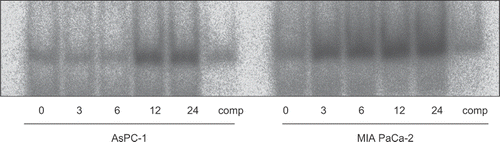
Figure 6. Gemcitabine-induced NF-κB was decreased by the combination therapy with heat treatment. The binding activity of NF-κB was assessed after 12 h of gemcitabine treatment or 24 h of heat treatment. AsPC-1 cells were exposed to four kinds of stimuli. The results for no treatment (control) (lane 1), treatment with gemcitabine alone (30 μM) (lane 2), treatment with heat alone (43°C, 1 h) (lane 3), and heat treatment combined with gemcitabine treatment (lane 4) are shown.
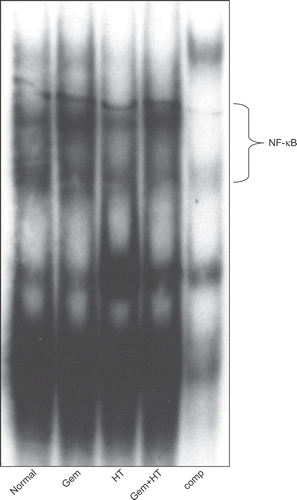
Heat pretreatment inhibited gemcitabine-induced activation of NF-κB
In this series of experiments, AsPC-1 and MIAPaCa-2 cells were treated with heat 24 h before the treatment with gemcitabine. The status of NF-κB activation was determined by immunocytochemical staining using an antibody specific for RelA (p 65). In the untreated cells (control), the p65 subunit was localized exclusively in the cytoplasm. On the other hand, treatment with gemcitabine alone induced translocation of p65 into the nucleus. Heat treatment combined with gemcitabine treatment decreased the translocation of p65 ().
Figure 7. The status of NF-κB activity was determined by immunocytochemical staining using an antibody specific for p65. AsPC-1 cells were grown on gelatin-coated 8-well chambered glass slides and treated with heat 24 h before the treatment with gemcitabine (30 μM). The results for no treatment (control) (a), treatment with gemcitabine (30 μM) (b), treatment with heat (43°C, 1 h) (c), and heat treatment combined with gemcitabine treatment (d) are shown. In the untreated cells (control), the p65 subunit was localized exclusively in the cytoplasm. On the other hand, treatment with gemcitabine induced the translocation of p65 into the nucleus. Heat treatment inhibited the gemcitabine-induced translocation of NF-κB.
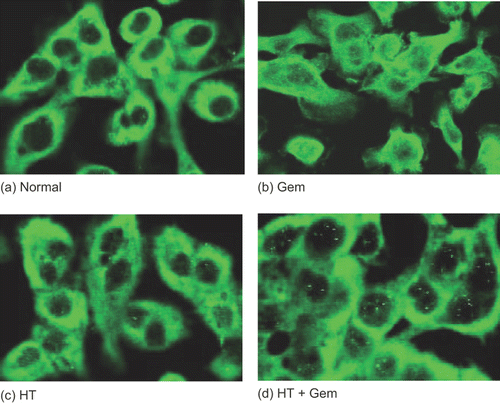
Cell growth after heat treatment combined with gemcitabine treatment
Untreated cells (control) showed normal growth. Gemcitabine alone reduced the total number of cells compared with the untreated control. Heat treatment combined with gemcitabine treatment caused a further decrease in the total number of cells compared with the untreated control ().
Figure 8. The effect of heat treatment in gemcitabine-mediated cytotoxicity. When heat treatment was given 24 h before the treatment with gemcitabine, a significant decrease in the total number of cells was seen compared with treatment with gemcitabine alone. Values represent mean ± SEM of three samples of a representative experiment; similar results were obtained in three independent experiments. *P < 0.05, compared with the control. **P < 0.01, compared with the control in AsPC-1 cells.
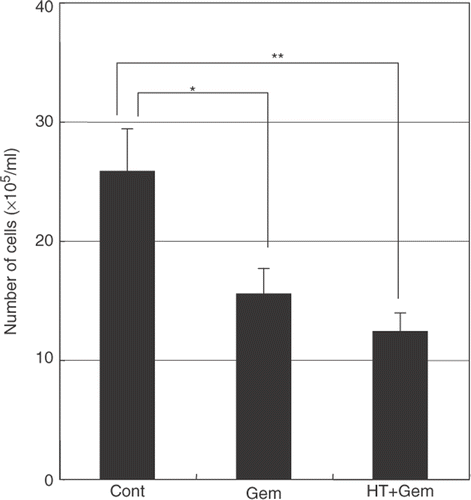
Protein expression levels of Hsp70, pro-caspase3, VEGF, and cyclinD1
AsPC-1 and MIAPaCa-2 cells were evaluated from the levels of proteins that are associated with NF-κB by western blotting.
In this experiment, AsPC-1 and MIAPaCa-2 cells were treated with heat 24 h before treatment with gemcitabine (30 μM) for 24 h. At the end of treatment with gemcitabine, both cell lines were left for 24 h in the incubator at 37°C. Increasing levels of Hsp70 were induced by heat treatment and this effect was further increased by heat treatment combined with gemcitabine treatment in MIAPaCa-2 cells. In contrast, gemcitabine alone did not affect the protein level of Hsp70. In AsPA-1 cells, increased levels of Hsp70 were also induced by heat treatment (data not shown). On the other hand, western blotting showed that heat treatment in combination with gemcitabine treatment reduced the levels of pro-caspase3, VEGF, and cyclinD1 compared with the control ().
Figure 9. The expression levels of Hsp70, pro-caspase-3, VEGF, cyclin D1, and anti-apoptosis proteins. Cells were treated with heat 24 h before the treatment with gemcitabine (30 μM). Whole cell extracts were analyzed by western blotting. The results for no treatment (control) (lane 1), treatment with gemcitabine alone (30 μM) (lane 2), heat treatment alone (43°C, 1 h) (lane 3), and heat treatment combined with gemcitabine treatment (lane 4) are shown. Significant levels of Hsp70 were induced by heat treatment in MIAPaCa-2 cells. In contrast, gemcitabine treatment alone did not affect the protein levels of Hsp70. The levels of VEGF, pro-caspase-3, cyclin D1, and anti-apoptosis protein were decreased by heat treatment combined with gemcitabine treatment.
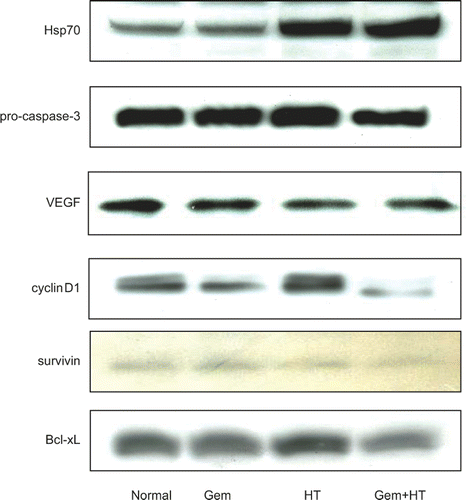
Expression of anti-apoptosis proteins
The levels of anti-apoptosis proteins, such as survivin and Bcl-xL, were decreased by heat treatment combined with gemcitabine treatment in MIAPaCa-2 cells ().
Discussion
This study provided evidence to support the hypothesis that gemcitabine-mediated activation of NF-κB in pancreatic cell lines might be inhibited by heat treatment, resulting in the enhancement of gemcitabine-induced cytotoxicity.
The present results showing that gemcitabine treatment decreased the cell viability in both AsPC-1 and MIAPaCa-2 pancreatic carcinoma cell lines suggest that gemcitabine activates the apoptosis pathway in two different pancreatic carcinoma cell lines. In addition, the present FACS analysis revealed that more gemcitabine-treated carcinoma cells entered apoptosis than untreated carcinoma cells.
In general, many kinds of anti-cancer agents, including gemcitabine, attack the DNA and activate the apoptotic pathway. However, NF-κB is often activated by anti-cancer agents, such as 5-FU and CPT-11, and NF-κB activation is one of the mechanisms by which tumors are induced to become resistant to chemotherapy Citation1,Citation2. Therefore, the blocking of NF-κB activation may enhance the anti-tumor effects of chemotherapy and may prevent chemo-resistance.
In this study, gemcitabine activated NF-κB binding activity in both AsPC-1 and MIAPaCa-2 pancreatic carcinoma cell lines and treatment with heat significantly attenuated gemcitabine-mediated NF-κB activation. Taken together with the previous reports Citation10,Citation11, this study strongly suggests that the combination therapies of hyperthermia with gemcitabine might show enhanced cell cytotoxicity on carcinoma cells by blocking the activation of NF-κB.
Although inhibition of the activation of NF-κB by hyperthermia is relatively well known Citation12,Citation13, an understanding of the detailed mechanisms by which heat treatment blocks the activation of NF-κB remains incomplete. Previous studies from our laboratory have demonstrated that Hsp70 and Hsp32 induced by hyperthermia play important roles in the inhibition of NF-κB activation. If heat-sensitive proteins such as Hsp70 and Hsp32 induced by heat treatment inhibit NF-κB activation, resulting in the enhancement of gemcitabine-mediated cytotoxicity in AsPC-1 and MIAPaCa-2 cell lines, the timing of heat treatment relative to gemcitabine treatment must be important. Thus, we developed a protocol in which the two pancreatic cell lines were treated with gemcitabine and heat at four relative times. AsPC-1 and MIAPaCa-2 cells were treated with heat 24 h before the treatment with gemcitabine (30 μM), simultaneously with gemcitabine treatment, 24 h after gemcitabine treatment, and 48 h after gemcitabine treatment. The treatment with gemcitabine alone showed significant cytotoxicity. Heat treatment combined with gemcitabine treatment enhanced cytotoxicity, especially when heat treatment was performed 24 h before the treatment with gemcitabine. Taken together with previous reports Citation12,Citation13, this study suggests that heat treatment enhances the cytotoxicity of gemcitabine through the inhibition of NF-κB. We had already examined the time course of the expression of Hsp70 after heat treatment in AsPC-1 and MIAPaCa-2 cells. Heat induced Hsp70 expression 3 h later and this induction was continued at least for 72 h. shows the Hsp70 expression 72 h after heat treatment. On the other hand, the activation of NF-κB was initiated between 3 h and 24 h after cancer cells were exposed to gemcitabine. Therefore, the inhibitory effect of Hsp70 on NF-κB activation by gemcitabine might be exercised during this period.
The present western blotting analysis showing that the expression of NF-κB-regulated proteins were inhibited by heat treatment combined with gemcitabine suggests that heat-treatment-mediated inhibition of NF-κB may be effective not only in the inhibition of increasing numbers of cancer cells in vitro but also in the inhibition of tumor growth in vivo. Survivin and Bcl-xL were decreased by treatment with heat plus gemcitabine, which might enhance the induction of apoptosis of AsPC-1 and MIAPaCa-2 cells. The decrease in cyclin D1 might affect the proliferation of these cells. The decrease in cell counts from the treatment of gemcitabine alone or the treatment of heat plus gemcitabine is due not only to apoptosis in the carcinoma cell line but also to cell cycle delay. According to our data shown in , the expression of cyclin D1 was down-regulated by the treatment of gemcitabine alone and further down-regulated by treatment of heat plus gemcitabine. This present study suggests that heat-treatment-mediated inhibition of NF-κB caused the decrease in cell numbers by both the induction of apoptosis and the attenuation of cell proliferation.
Furthermore, the decrease in VEGF by heat plus gemcitabine may inhibit tumor growth in vivo. This scenario is supported by the previous report from Solorzano et al. Citation14, which shows that VEGF plays a key role in regulating tumor vascularization, and is further supported by other studies that indicate that an over-expression of NF-κB is the key component of the angiogenic cascade, which contributes to VEGF-induced angiogenesis through the up-regulation of VEGF mRNA expression in many tumors Citation15.
In clinical practice, we perform hyperthermia (heat treatment) while patients receive an intravenous infusion of anti-cancer agents or immediately after intra-arterial infusion of anti-cancer agents. However, in the case of anti-cancer agents such as gemcitabine that induce the activation of NF-κB, we conclude that it is better to perform hyperthermia before chemotherapy.
References
- Wang CY, Mayo MW, Baldwin AS, Jr. TNF- and cancer therapy-induced apoptosis: Potentiation by inhibition of NF-kappaB. Science 1996; 274: 784–787
- Wang CY, Cusack JC, Jr, Liu R, Baldwin AS, Jr. Control of inducible chemoresistance: Enhanced anti-tumor therapy through increased apoptosis by inhibition of NF-kappaB. Nat Med 1999; 5: 412–417
- Vertrees RA, Das GC, Popov VL, Coscio AM, Goodwin TJ, Logrono R, Zwischenberger JB, Boor PJ. Synergistic interaction of hyperthermia and gemcitabine in lung cancer. Cancer Biol Ther 2005; 4: 1144–1153
- Pereira S, Fernandes PA, Ramos MJ. Mechanism for ribonucleotide reductase inactivation by the anticancer drug gemcitabine. J Comput Chem 2004; 25: 1286–1294
- Hertel LW, Boder GB, Kroin JS, Rinzel SM, Poore GA, Todd GC, Grindey GB. Evaluation of the antitumor activity of gemcitabine (2′,2′-difluoro-2′-deoxycytidine). Cancer Res 1990; 50: 4417–4422
- Bold RJ, Chandra J, McConkey DJ. Gemcitabine-induced programmed cell death (apoptosis) of human pancreatic carcinoma is determined by BCL-2 content. Ann Surg Oncol 1999; 6: 279–285
- Banerjee S, Zhang Y, Ali S, Bhuiyan M, Wang Z, Chiao PJ, Philip PA, Abbruzzese J, Sarkar FH. Molecular evidence for increased antitumor activity of gemcitabine by genistein in vitro and in vivo using an orthotopic model of pancreatic cancer. Cancer Res 2005; 65: 9064–9072
- Arlt A, Gehrz A, Muerkoster S, Vorndamm J, Kruse ML, Folsch UR, Schafer H. Role of NF-kappaB and AKT/PI3K in the resistance of pancreatic carcinoma cell lines against gemcitabine-induced cell death. Oncogene 2003; 22: 3243–3251
- Guzhova IV, Darieva ZA, Melo AR, Margulis BA. Major stress protein hsp70 interacts with NF-κB regulatory complex in human t-lymphoma cells. Cell Stress Chaperones 1997; 2: 132–139
- Ran R, Lu A, Zhang L, Tang Y, Zhu H, Xu H, Feng Y, Han C, Zhou G, Rigby AC, Sharp FR. Hsp70 promotes TNF-mediated apoptosis by binding ikk gamma and impairing NF-kappaB survival signaling. Genes Dev 2004; 18: 1466–1481
- Frossard JL, Pastor CM, Hadengue A. Effect of hyperthermia on NF-kappaB binding activity in cerulein-induced acute pancreatitis. Am J Physiol Gastrointest Liver Physiol 2001; 280: G1157–1162
- Nakabe N, Kokura S, Shimozawa M, Katada K, Sakamoto N, Ishikawa T, Handa O, Takagi T, Naito Y, Yoshida N, et al. Hyperthermia attenuates TNF-alpha-induced up regulation of endothelial cell adhesion molecules in human arterial endothelial cells. Int J Hyperthermia 2007; 23: 217–224
- Sakamoto N, Kokura S, Okuda T, Hattori T, Katada K, Isozaki Y, Nakabe N, Handa O, Takagi T, Ishikawa T, et al. Heme oxygenase-1 (hsp32) is involved in the protection of small intestine by whole body mild hyperthermia from ischemia/reperfusion injury in rat. Int J Hyperthermia 2005; 21: 603–614
- Solorzano CC, Jung YD, Bucana CD, McConkey DJ, Gallick GE, McMahon G, Ellis LM. In vivo intracellular signaling as a marker of antiangiogenic activity. Cancer Res 2001; 61: 7048–7051
- Fujioka S, Sclabas GM, Schmidt C, Frederick WA, Dong QG, Abbruzzese JL, Evans DB, Baker C, Chiao PJ. Function of nuclear factor kappaB in pancreatic cancer metastasis. Clin Cancer Res 2003; 9: 346–354