Abstract
The proteasome inhibitor bortezomib exhibits antitumor activity in many malignancies including mantle cell lymphoma (MCL). Unfortunately, many patients fail to respond to treatment or become refractory. Hyperthermia is an effective chemosensitizer that in combination with some chemotherapeutic agents has shown clinical activity in phase II and III studies. The aim of this study was to use MCL cell lines to investigate the potential benefit of combining clinically relevant doses of bortezomib with two different thermal doses (41.8°C/120 min and 44°C/30 min) that mimic the heterogeneity of the temperature distributions achieved within tumors during hyperthermia. Treated tumor cells were assessed for proliferation using the WST-1 assay and for apoptosis by annexin V staining, while heat shock protein (HSP) levels were determined following western blot analysis. Our results demonstrated that MCL cell lines that are sensitive to bortezomib are also thermosensitive and have low basal expression of hsp27, whereas the bortezomib-resistant MCL cell line strongly expresses hsp27 and is thermoresistant. Interestingly, pre-treatment of MCL cell lines with heat at the two different thermal doses, and the transient elevation of hsp27 and hsp70, do not impair their primary sensitivity to bortezomib. Finally, we show that the concurrent treatment of heat and bortezomib results in additive killing in MCL cell lines.In conclusion, these results suggest that the application of bortezomib, under thermal conditions, in mantle cell lymphoma cells may be beneficial and warrants further investigation.
Introduction
Bortezomib (Velcade®) is a potent, selective, and reversible inhibitor of the 26S proteasome. The proteasome is composed of a 20S core particle where proteolysis takes place and a 19S thermosensitive regulatory particle. Substrates include various oncogenes and regulatory proteins for cell cycle progression and apoptosis Citation1. The ability of proteasome inhibitors to disrupt cell cycle progression has made this class of small molecule inhibitors attractive chemotherapeutic agents Citation2,Citation3. Bortezomib demonstrated clinical activity in multiple myeloma, for which it gained FDA and EMEA approval in 2004, and in many other malignancies Citation3–5 including mantle cell lymphoma Citation6,Citation7. Mantle cell lymphoma is a unique subtype of B-cell malignant lymphoma that behaves aggressively and remains incurable Citation8,Citation9. Although recently, phase II studies showed that bortezomib as a single agent can achieve durable and complete responses in MCL, many patients fail to respond to treatment or become refractory after an initial response Citation6,Citation10,Citation11. Therefore, efforts have been made to understand the mechanisms responsible for resistance and sensitivity to bortezomib, and to develop strategies that can overcome this resistance.
Hyperthermia is a cancer therapy that has been shown to have clinical activity when combined with radiation or chemotherapy Citation12–14. This improved response to radiation or drugs can result from a simple additive effect due to the heat treatment inducing a direct cytotoxicity effect in the tumor cells, or by sensitization in a temperature-dependent manner Citation15. It is known that heat causes a greater than additive cell killing when combined with alkylating agents, nitrosureas, and platinum drugs. In the case of cisplatinum, heat has been found to reverse cisplatinum resistance Citation16,Citation17, leading to a more general suggestion that hyperthermia could be instrumental in reversing drug-resistance. Thus, heat may be an ideal candidate for combination with bortezomib.
Heat shock proteins are molecular chaperones involved in the regulation of various vital cellular functions, including cell proliferation Citation18. Although HSPs are only induced transiently in cells after periods of stress as can occur with heat, they are often constitutively overexpressed in tumor cells Citation19. Indeed, HSPs are commonly expressed in MCL Citation20,Citation21 and in particular hsp90 overexpression has been found to correlate with the high-grade type of lymphoma Citation21. Recently, overexpression of heat shock proteins such as hsp27, hsp70 and hsp90 has been found to be associated with bortezomib resistance in B-lymphoma cells Citation22. A major concern, therefore, is the possible induction or increase of bortezomib resistance by heat-induced overexpression of HSPs.
In the present study, we aimed to evaluate the time- and temperature-dependent effects of heat exposure in three lymphoma cell lines that displayed differential sensitivity to bortezomib. Based on our kinetic studies, the time of bortezomib exposure for combination experiments was set to 24 h, a window where bortezomib shows an anticancer activity in all mantle cell lymphoma cell lines but does not kill the majority of the cells, allowing study of potential synergistic or additive effect of other anticancer agents or modalities. We investigated the combination of heat and bortezomib comparing two thermal doses, a severe and a mild thermal dose that mimic the heterogeneity of the temperature distribution achieved within tumors during hyperthermia treatment, and two different time points of the heat shock response which correspond to the basal and maximal expression of HSPs, to explore their role in influencing bortezomib sensitivity.
Materials and methods
Tumor cell lines and reagents
Seven cell lines were used. These included five tumor cell lines: Jeko-1, Rec-1, Granta 519, HBL-2 and NCEB-1, and two haematological control cell lines: Jurkat (T-ALL) and Karpas 422 (diffuse large cell lymphoma). All cell lines were either purchased from the German Collection of Microorganisms and Cell Lines (Deutsche Sammlung von Mikroorganismen und Zelllinien, Braunschweig, Germany) or provided by the principle investigators. Jeko-1, Granta 519, and NCEB-1 are human mantle cell lymphoma cell lines established from three different patients, a 78-year-old woman with B-cell non-Hodgkin's lymphoma (NHL), a 58-year-old woman with B-cell NHL, and a 57-year-old male with diffuse centroblastic-centrocytic B-NHL, respectively. Rec-1 and HBL-2 were established from the lymph nodes of two men who had terminal refractory B-cell non-Hodgkin lymphoma and were 61 and 74 years old, respectively. All cells were incubated at 37°C in a humidified atmosphere at 5% CO2/95% air and maintained in RPMI 1640 medium with L-glutamin plus 2 g/L NaHCO3 supplemented with 20% heat-inactivated fetal bovine serum and 1% penicillin/streptomycin. The proteasome inhibitor bortezomib (PS-341) was provided by Millennium Pharmaceuticals (Cambridge, MA) aliquoted and stored at −20°C.
Bortezomib treatment
Fixed cell numbers were incubated in the presence of increasing concentrations of bortezomib and harvested at several time points (12, 24, 48 and 72 h) and assayed for viability, survival, and apoptosis. For the combination experiments, escalating concentrations of bortezomib (ranging from 3.125 nM to 100 nM) were added shortly before or 24 h after heating at 41.8°C for 120 min or 44°C for 30 min in a temperature-controlled water bath Citation23. Control cells were incubated at 37°C with the same drug concentrations for exactly the same time. The inhibitory concentration of bortezomib was expressed as the IC50 dose (the half maximal inhibitory concentration), which represents the drug concentration that is required to inhibit 50% of the target cells.
Heat treatments
Heat exposure as a function of time was performed using two thermal doses: 41.8°C for 120 min and 44°C for 30 min, which mimic the heterogeneity achieved during clinical heat treatment. Heating was performed by directly immersing the cell culture flasks, sealed with parafilm, in a temperature-controlled water bath Citation24. Control cells were sealed and incubated at 37°C for exactly the same time as the heat-exposed samples. After treatment, the cells were returned to 37°C/5% CO2 and allowed to recover for up to 24 or 48 h. At these defined recovery time points, non-viable cells in the supernatant were removed and viable cells were harvested for analysis.
Cell viability, apoptosis, and cell proliferation assays
Cell viability was evaluated at several time points after treatment using the Trypan blue dye-exclusion assay. Only viable cells were harvested and treated with bortezomib. For the apoptosis assay cells were washed and stained with Annexin V-PE/7-ADD in accordance with the manufacturer's protocol (BD Biosciences, Heidelberg, Germany) and subjected to flow cytometry. The degree of apoptosis was calculated from the formula:
For the established MCL cell lines the spontaneous apoptosis rate was 10%. Cell proliferation was measured using the WST-1 Cell Proliferation Assay (Roche Diagnostics, Mannheim, Germany). Briefly, lymphoma cells were exposed to 41.8°C/120 min and 44°C/30 min, and at defined time-points viable cells were harvested, seeded and incubated in 96-well culture plates in the presence of increasing concentrations of bortezomib for 24 h at 37°C. WST was then added to each well for 4 h at 37°C. Absorbance was measured at 450 nm/690 nm using a standard reader (Optimax Pro, Molecular Devices; Sunnyvale, CA). Cell proliferation was estimated as a percentage of the untreated control cells (37°C). Triplicate wells were used for each experimental condition and all experiments were repeated at least three times.
Western blot analysis
Mantle cell lymphoma cells were heated at 41.8°/120 min or 44°C/30 min and allowed to recover at 37°C for different times (0 to 48 h). An equal amount of viable cells were harvested, washed, and lysed in 100 µL buffer (150 mM NaCl; 50 mM TRIS, pH 8; 1% NP40) containing 1 µL/mL leupeptin and 2 µL/mL of protease inhibitors. Cells were incubated for 30 min on ice, centrifuged for 15 min at 4°C and boiled for 10 min at 95°C.
Cell lysates were loaded onto one lane and separated by SDS-PAGE. Cell lysates adjusted for an equal number of cells and an equal quantity of proteins (measured by the Bradford assay) were compared. After electrophoresis, proteins were transferred to nitrocellulose membranes (Sartorius Nitrocellulose Blotting Membranes, Göttingen, Germany) and probed overnight at 4°C with appropriate antibodies. The following monoclonal antibodies were used: the rat antibody IgG1 6B3 Citation25 against hsp70, a mouse antibody against hsp27 (SPA-800, Stressgen Bioreagents), a mouse antibody against hsp90 (SPA-830, Stressgen), and a rat antibody against Hsc70 (SPA-815, Stressgen). A secondary peroxidase-conjugated mouse anti-rat IgG (Jackson ImmunoResearch Laboratories, PA) or goat anti-mouse IgG (Bio-Rad Laboratories, CA) (1:2000) was added for 1 h at room temperature. Detection of the protein/antibody complexes was achieved using the ECL system (Amersham Life Science, Karlsruhe, Germany).
Statistical analysis
The statistical significance of experimental values was assessed by means of an independent Student's t-test on two populations. P-values of p < 0.05 were considered significant.
Results
Time- and dose-dependent differential sensitivity of mantle cell lymphoma cell lines to bortezomib
The kinetics of cytotoxicity, the degree of apoptosis, and antiproliferative activity induced by treatment with bortezomib was evaluated in all cell lines. For assessing viability, cells were incubated with increasing concentrations of bortezomib (0 nmol/L to 50 nmol/L) and harvested at 24, 48 and 72 h. In all cell lines a dose- and time-dependent inhibition of cell growth was observed, with differential sensitivity to the proteasome inhibition among the cell lines. These effects are illustrated in and . (left panel) shows the time dependency using a clinically achievable concentration of bortezomib (25 nM) Citation23 and demonstrates a strong inhibition of cell growth in Jeko-1, HBL-2 and Karpas 422 within 48 h, whereas in NCEB-1 the same exposure time only inhibited growth by 30%. shows the drug concentration dependency after 24 h of treatment in three of the mantle cell lymphoma cell lines. From these results the cell lines can be ranked as having high (Jeko-1), intermediate (Rec-1), or low (NCEB-1) sensitivity to bortezomib.
Figure 1. Cell survival expressed as percentage of proliferating cells of Jeko-1, Rec-1 and NCEB-1 in the presence of increasing concentrations of bortezomib. Cells were incubated with bortezomib (from 0 to 100 nmol/L) for 24 h in a humidified atmosphere at 37°C. Cells were harvested and the relative number of proliferating cells after treatment was assessed by standard WST-1 assay. Error bars represent mean ± standard deviation of triplicates in one representative experiment.
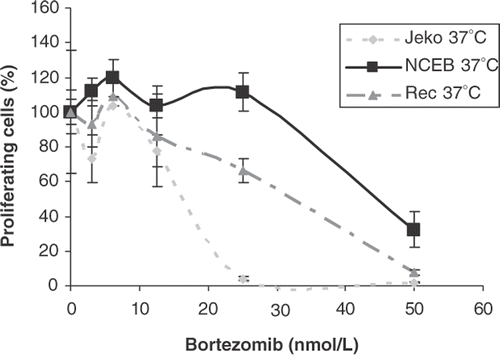
To detect the early and late apoptosis events induced by bortezomib at the clinically relevant concentration (25 nM), viable cells were analysed for apoptosis using flow cytometry after 12 and 24 h of drug exposure. NCEB-1 again arose as the most resistant cell line and Jeko-1 the most sensitive, whereas Rec-1, HBL-2 and Granta 519 showed an intermediate sensitivity (data not shown).
Table I. Kinetics of viability in MCL and haematological control cell lines following bortezomib treatment (left column). Cells were incubated with 25 nM of bortezomib, harvested at 24, 48 and 72 h and assessed for viability. The right column lists the IC50 values derived from the proliferating activity assessed by WST-1 assay in all cell lines treated for 24 h with increasing concentrations of bortezomib.
Finally, the antiproliferative activity of bortezomib at increasing concentrations (3.125 nmol/L to 100 nmol/L) for 24 h in the various cell lines were compared by determining the IC50 doses, and confirmed the previous pattern of sensitivity. The highest IC50 value was again achieved with NCEB-1. This was followed by Rec-1, Granta 519, Jurkat, HBL-2, Karpas 422 and finally Jeko-1 (, right panel).
In conclusion, cytotoxicity, antiproliferative activity and apoptosis assays consistently showed that exposure to bortezomib differentially affects mantle cell lymphoma cells in a time- and dose-dependent manner. To explore the interaction and potential benefit of bortezomib in combination with hyperthermia, we selected three mantle cell lymphoma cell lines with high (Jeko-1), intermediate (Rec-1) and low sensitivity to bortezomib (NCEB-1) ().
Thermal dose-related differential effects of heat on cell viability and survival of mantle cell lymphoma cells
Cells were exposed to a mild heat temperature of 41.8°C for 120 min and a more severe heat treatment of 44°C for 30 min. After heating, the cells were allowed to grow at 37°C/5% CO2 for 24 h before being harvested. Viability and cell survival were assessed using the Trypan-blue exclusion and WST-1 assays, respectively. As expected, exposure of cells to the lower thermal dose (41.8°C/120 min) had no or minimal impact on survival and viability in all three cell lines selected. Survival rates expressed as a percentage relative to control cells treated at 37°C are illustrated in (left panel). Exposure of Jeko-1 and Rec-1 to a more severe thermal dose (44°C/30 min) did reduce tumor cell survival, whereas for NCEB-1 cells no cell killing was observed after exposure to this larger thermal dose (, right panel). These survival patterns show that heat differentially affects mantle cell lymphoma cells in a time- and temperature-dependent manner and suggest that NCEB-1 is more thermoresistant in comparison to Jeko-1 and Rec-1.
Table II. Survival rate in Jeko-1, Rec-1 and NCEB-1 after heat treatment at a mild thermal dose (41.8°C/120 min, left column) and at a severe thermal dose (44°C/30 min, right column). Cells were first heated and allowed to recover at 37°C in a humidified athmosphere for 24 h. Proliferation rate was evaluated by a standard WST assay.
Differential constitutive expression and heat-induced overexpression of heat shock proteins in mantle cell lymphoma cell lines
To compare the basal expression of HSPs in the three selected MCL cell lines at the physiological growth temperature of 37°C, an equal amount of viable cells were harvested and the expression level of the constitutively expressed Hsc70, hsp70, hsp27, and hsp90 were compared using western blot analysis. At 37°C, hsp70, hsp27, and hsp90 were detectable in all three cell lines, although the basal expression of the inducible hsp70 was low in all lines, and NCEB-1 expressed a much higher basal level of hsp27 than Rec-1 and Jeko-1 (a).
Figure 2. Expression level of hsp27, hsp70, Hsc70, and hsp90 by western blot analysis in an equal number of Jeko-1, Rec-1, and NCEB-1 cells at the physiological growth temperature of 37°C (a). Kinetics of expression of hsp27, hsp70, Hsc70, and hsp90 in Jeko-1, Rec-1 and NCEB-1 after exposure to a mild thermal dose (41.8°C/120 min) (b), and to 44°C/30 min (c). ß-actin was used as a protein loading control. After heat treatment at the selected thermal doses cells were allowed to recover in a humidified atmosphere at 37°C for up to 48 h. At the time points 4, 16, 24 and 48 h an equal numbers of cells were harvested for western blot analysis. Control cells were kept at 37°C (left column of each panel).
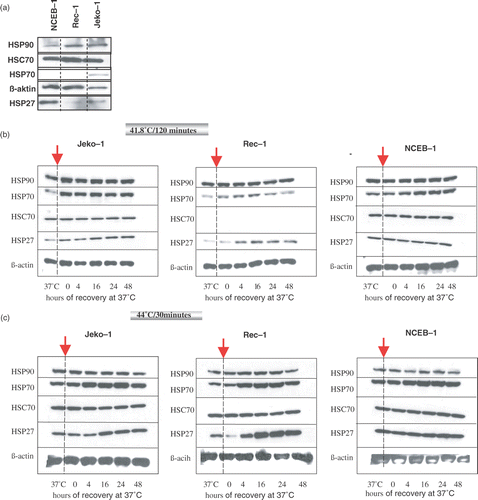
The characteristics of the induction of the heat shock proteins following heating in the selected lymphoma cell lines was assessed by treating cells with the two thermal doses (41.8°C/120 min and 44°C/30 min) and allowing the cells to recover at 37°C for 4, 16, 24, and 48 h. At these time points the expression levels of Hsc70 (constitutive), hsp70 (inducible), hsp27, and hsp90 were investigated using western blot analysis by comparing both an equal number of cells and an equal quantity of proteins among the cell lines (data not shown). c show the kinetics of HSPs expression in Jeko-1, Rec-1 and NCEB-1.
The basal expression of the constitutive form of the hsp70 family (Hsc70) and of hsp90 in all three cell lines, as expected, was not impaired by heat exposure at both thermal doses. Inducible hsp70 was transiently overexpressed with a peak between 16–24 h of recovery after exposure to heat treatment at 41.8°C/120 min (b) and 44°C/30 min (c) in all three cell lines, whereas hsp27 was strongly induced only in Rec-1 and Jeko-1 but not in NCEB-1 (b).
The degree of increase of inducible HSPs is thermal dose-dependent as demonstrated by the stronger increase of hsp70 and hsp27 after exposure to 44°C/30 min compared to 41.8°C/120 min (c).
Pre-treatment with heat and heat-induced overexpression of heat shock proteins does not interfere with the effects of bortezomib in MCL cell lines regardless of the thermal dose
To investigate whether or not the upregulation of HSPs by heat shock treatment correlates with an increase or induction of bortezomib resistance, the three selected MCL cell lines with differential sensitivity to bortezomib and heat were first treated at 41.8°C/120 min or 44°C/30 min and then allowed to grow at 37°C for 24 h. After this time of recovery viable cells were then incubated in the presence of increasing concentrations of bortezomib for an additional 24 h. The effect on cell survival of this combined treatment was assessed by WST assay. shows survival of Jeko-1, Rec-1 and NCEB-1 after treatment with bortezomib vs. the same treatment given 24 h of recovery after heat exposure to 41.8°C/120 min or 44°C/30 min. These survival curves showing the concentration dependency as well as the respective IC50 values clearly show that the pattern of bortezomib sensitivity after heat treatment remained virtually unchanged in all three cell lines in comparison to bortezomib treated cells at 37°C regardless of the concentration of bortezomib or the thermal dose applied ().
Figure 3. Cell survival expressed as percentage of proliferating cells in Jeko-1 (a), NCEB-1 (b), and Rec-1 (c), treated with increasing concentrations of bortezomib after a pre-treatment with heat at 41.8°C/120 min or 44°C/30 min. Briefly, cells were first heated, then allowed to recover for 24 h in a humidified atmosphere at 37°C. At this point cells were treated with bortezomib (from 0 to 100 nmol/L) for 24 h and then harvested for survival assessment by standard WST-1. Survival is expressed as a percentage of control cells (bortezomib-untreated) and compared with non-heated cells (37°C). Error bars represent mean ± standard deviation of three independent experiments. The percentage of proliferating cells at each concentration of bortezomib tested did not significantly differ between heated and non-heated cells and p-value was largely >0.5 (*).
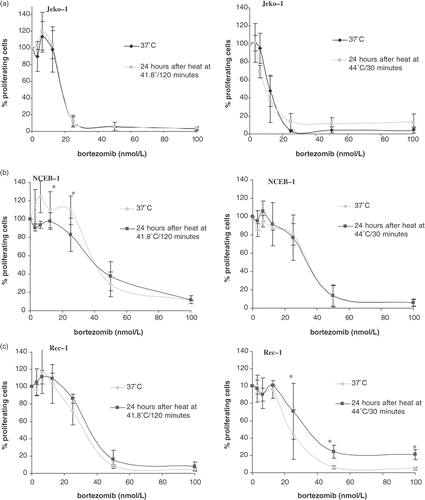
Concurrent application of heat and bortezomib is additive in MCL cell lines
To investigate the biological effect of the combination of bortezomib and heat, mantle cell lymphoma cells were concurrently exposed to heat at the two selected thermal doses and increasing concentrations of bortezomib for 24 h. The effect on cell survival was assessed with the WST assay and compared using the IC50 values, as summarized in . In all three cell lines the IC50 values, as well as the survival curves after bortezomib alone versus concurrent bortezomib and heat at 41.8°C/120 min or at 44°C/30 min (figure not shown) clearly demonstrate that there is no synergism regardless of the cell line, the thermal dose applied, or the bortezomib concentration used. In particular, in the most heat- and bortezomib-resistant NCEB-1 cell line, the combination treatment did not reverse resistance even at sub-therapeutic concentrations. However, when the survival rates of heated cells treated with bortezomib were compared to untreated control cells at 37°C, an additive effect was found in all three cell lines in particular at concentrations <25 nM, considered to be the clinically achieved concentration. shows the survival of cells expressed as percentage of proliferating cells treated at 44°C/30 min alone or with the combination of heat and bortezomib. At the IC50 concentration, survival of heated cells in Jeko-1 and Rec-1 was about 20% compared to 50% in control cells (, dotted lines), showing a clear additive effect of heat to the bortezomib treatment, whereas for NCEB-1 this additive effect was not evident at the IC50 (c).
Figure 4. Cell survival expressed as a percentage of proliferating cells in Jeko-1 (a), Rec-1 (b), and NCEB-1 (c), treated with increasing concentrations of bortezomib given at 37°C (black line) or given concurrently to heat at 44°C/30 min (grey line). Survival of heated cells is expressed as a percentage of untreated cells at 37°C. Briefly, cells were first treated with bortezomib (from 0 to 100 nmol/L) and immediately heated. After the concomitant treatment, cells were incubated for 24 h in a humidified atmosphere at 37°C. At this point cells were harvested for survival assessment by standard WST-1. Error bars represent mean ± standard deviation of four independent experiments. Dotted lines indicated the IC50.
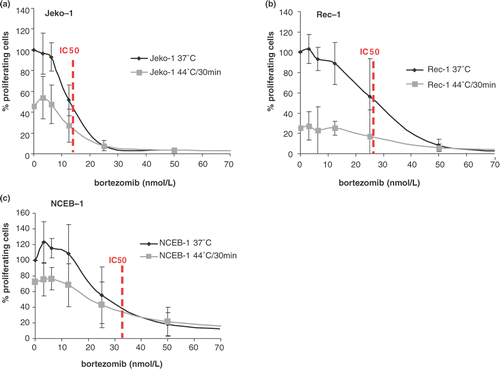
Overall, the combination of bortezomib and heat at a lethal temperature has an additive but not synergistic effect in thermosensitive MCL cell lines.
Table III. IC50 values obtained in Jeko-1, Rec-1, and NCEB-1 cells in the presence of increasing concentrations of bortezomib (from 0 to 100 nmol/L) for 24 h at: 37°C (control cells) listed in the left column; concomitant to heat treatment (middle column); or 24 h after pre-treatment with heat at the indicated thermal doses (right column).
Discussion
Exposure to bortezomib affects mantle cell lymphoma (MCL) cells in a time- and dose-dependent manner, although variations occur among various cell lines ( and ). In the present study we evaluated the time- and temperature-dependent effects of heat exposure in combination with bortezomib in three mantle cell lymphoma cell lines (Jeko-1, Rec-1, and NCEB-1) that displayed high, intermediate and low sensitivity to bortezomib (). We observed that cell lines sensitive to heat were also sensitive to bortezomib, whereas the most bortezomib-resistant cell line, NCEB-1, was also the most thermoresistant. All three MCL cell lines expressed basal levels of the heat shock proteins 70, 27, and 90 and in particular the most thermo- and bortezomib-resistant cell line NCEB-1 expressed a much higher basal level of hsp27 than the more sensitive cell lines (a). It has been suggested that high basal expression of HSPs can protect against proteasome inhibitor-induced apoptosis; in particular Shringapure et al. showed a correlation between expression of hsp27, hsp70, and hsp90 and the bortezomib-resistant phenotype in a gene expression analysis of B-cell lymphoma Citation22. In our study in particular, we did not find a stronger basal expression of hsp70 or hsp90 but only of hsp27 in the more resistant cell line, supporting a previous observation that only hsp27 confers resistance to proteasome inhibition Citation22,Citation26. Indeed, hsp27 blockade overcomes bortezomib resistance in lymphoma cells, whereas ectopic expression of wild-type hsp27 renders bortezomib-sensitive cells resistant Citation26.
It is generally assumed that HSP expression becomes deregulated in cancer, leading to elevated expression. Elevated HSP expression promotes cancer by inhibiting programmed cell death (hsp27, hsp70) and by promoting autonomous growth (hsp90), and that this leads to resistance to chemotherapy and hyperthermia Citation19. Many compounds have been recently developed that directly target the heat shock response by blocking HSF1 or HSP mRNA translation that dramatically sensitizes cancer cells to proteasome inhibitors Citation27. Inhibition of hsp90 by geldanamycin, moreover, has been found to exert an antilymphoma activity by increasing the proteasomal degradation of hsp90 client proteins, and to sensitize cancer cells to bortezomib Citation28,Citation29. Consequently, a major concern in cancer therapy is the potential increase in drug resistance by the induction of HSPs.
In the present study we observed that tumor cells with much higher levels of hsp27 and hsp70 induced upon heat are no more resistant to bortezomib than tumor cells with low basal levels of these HSPs. Indeed, the maximal expression of hsp27 and hsp70 achieved between 16 and 24 h after heat exposure did not correlate with an increased resistance to bortezomib in Jeko-1, Rec-1, or NCEB-1 (, ). This effect was found regardless of the thermal dose applied, indicating that the degree of inducible HSPs, which are higher after a more severe thermal stress, is not critical in conferring or impacting resistance. This observation is consistent with previous evidence that tumor cells with more HSPs are not more resistant to chemotherapy than those with low basal levels Citation30. Most importantly, it disproves the hypothesis that pre-treatment of cells with heat shock results in an increased resistance to proteasome inhibitors Citation27,Citation31. A possible explanation for this is that, unlike thermotolerant cells in which transiently elevated levels of HSPs are freely available to interact with new substrates, the pool of HSPs in tumor cells could be engaged in chaperone complexes and unavailable for use in cytoprotection Citation19. In summary, we observed in our in vitro experiments that a pre-treatment with heat and the heat-induced overexpression of heat shock proteins 27 and 70 does not interfere with the sensitivity to proteasome inhibitors in MCL cell lines.
The response of cells to stress depends upon its severity, which is a product of intensity and duration. Also, the proteasome is a target of heat and impact of its activity is likely to correlate with the severity of heat. In agreement with previous findings Citation32,Citation33 Pajonk et al. reported that severe heat shock at 44°C for 60 minutes rapidly inhibited the proteasome activity resulting in NF-κB inhibition, by the downregulation of the regulatory, thermosensitive subunit 19S in human prostate cancer cells Citation34. Conversely, it has been found that proteasome levels may even increase in response to long-term mild stress Citation1.
Hyperthermia modifies the cytotoxicity of many chemotherapeutic agents Citation15. The extent of thermal chemosensitization can be quantified by comparing clonogenic cell growth and proliferation activity in vitro or tumor growth inhibition in vivo when treated with the drug alone and with the same drug at elevated temperature. It is generally accepted that most alkylating agents (e.g., cyclophosphamide and ifosfamide), platinum compounds, and nitrosoureas (e.g., BCNU and CCNU) are linearly enhanced in their cytotoxic effect if temperatures are raised from 37°C to over 40°C, and exert more than an additive effect. In contrast, most antimetabolites (e.g., 5-fluorodeoxyuridin and methotrexate), as well as vinca-alkaloids or taxanes, show an independent action (additive effect). Studies on drug-heat sequence show, in general, that drugs administered immediately before or concomitantly to hyperthermia are most effective. The interaction between proteasome inhibitors and hyperthermia has been explored recently in a human colon cancer cell system Citation35. Chen et al. found a significant synergistic effect on cell viability with proteasome inhibition under hyperthermic conditions of 43°C for 60 minutes and showed that the concomitant combination of heat and proteasome inhibition decreased hsp27 levels, leading to thermal sensitization and increased apoptosis Citation35. The proposed mechanism of sensitization to both heat and to the proteasome inhibition through a decrease in hsp27 is plausible, as it is known that hsp27 blockade sensitizes both thermoresistant cells and cells resistant to chemotherapy in general and in particular to bortezomib. However, both heat shock as well as proteasome inhibition per se induce the heat shock response and a transient overexpression of inducible HSPs Citation2,Citation36, so that this mechanism must be strongly time-dependent. In our experimental setting, the concurrent application of heat at two thermal doses and bortezomib in increasing concentration neither reversed bortezomib resistance in NCEB-1, that highly expressed high basal level of hsp27, nor produced more than additive killing (synergism) as a result of sensitization in Jeko-1 and Rec-1, regardless of the thermal dose applied. In our in vitro study the concomitant application of heat and bortezomib led merely to additive killing (independent action) at concentrations of bortezomib <20 nM and at thermal doses above the temperatures that induce exponential cell killing (), and heat-induced overexpression of hsp27 did not interfere with bortezomib sensitivity. There are several possible explanations for the discrepancy in these results. Firstly, it has to be mentioned that NCEB-1 has five to eight stable murine chromosomes and expresses human and murine bcl-2 protein Citation37, so that possibly antiapoptotic genes may be differently regulated. Secondly, the MCL cell lines and colon cancer cells may indeed display different patterns of HSPs basal expression and behave differently following heat shock. Finally, the proteasome inhibitors were applied and their concentrations were different in the two systems; we chose increasing concentrations of bortezomib in a range (0–100 nM) that reproduces the clinically achievable concentrations Citation23, whereas Chen et al., used MG132 at much higher concentrations (10 µM), which are likely to result in different biological and pharmacological responses Citation35.
Overall, despite the intrinsic limitation of a merely in vitro study, our results suggest that the application of bortezomib under thermal conditions may be beneficial and deserves further clinical investigation. Moreover, our results suggest that MCL may be an appropriate tumor entity for this combinational treatment since MCL cell lines are sensitive to bortezomib at clinically relevant concentrations, can be killed by heat in a temperature-dependent manner, and the combined treatment results in at least additive killing.
Declaration of interest: The authors report no conflicts of interest. The authors alone are responsible for the content and writing of the paper.
References
- Glickman MH, Raveh D. Proteasome plasticity. FEBS Lett 2005; 579(15)3214–3223
- Mitsiades N, Mitsiades CS, Poulaki V, Chauhan D, Fanourakis G, Gu X, Bailey C, Joseph M, Libermann TA, Treon SP, et al. Molecular sequelae of proteasome inhibition in human multiple myeloma cells. Proc Natl Acad Sci USA 2002; 99(22)14374–14379
- Boccadoro M, Morgan G, Cavenagh J. Preclinical evaluation of the proteasome inhibitor bortezomib in cancer therapy. Cancer Cell Int 2005; 5: 18
- Richardson PG, Barlogie B, Berenson J, Singhal S, Jagannath S, Irwin D, Rajkumar SV, Srkalovic G, Alsina M, Alexanian R, et al. A phase 2 study of bortezomib in relapsed, refractory myeloma. N Engl J Med 2003; 348(26)2609–2617
- Mitsiades N, Mitsiades CS, Richardson PG, Poulaki V, Tai YT, Chauhan D, Fanourakis G, Gu X, Bailey C, Joseph M, et al. The proteasome inhibitor PS-341 potentiates sensitivity of multiple myeloma cells to conventional chemotherapeutic agents: Therapeutic applications. Blood 2003; 101: 2377–2380
- O'Connor OA, Wright J, Moskowitz C, Muzzy J, MacGregor-Cortelli B, Stubblefield M, Straus D, Portlock C, Hamlin P, Choi E, et al. Phase II clinical experience with the novel proteasome inhibitor bortezomib in patients with indolent non-Hodgkin's lymphoma and mantle cell lymphoma. J Clin Oncol 2005; 23: 676–684
- Goy A, Younes A, McLaughlin P, Pro B, Romaguera JE, Hagemeister F, Fayad L, Dang NH, Samaniego F, Wang M, et al. Phase II study of proteasome inhibitor bortezomib in relapsed or refractory B-cell non-Hodgkin's lymphoma. J Clin Oncol 2005; 23: 667–675
- Dreyling M, Bergsagel PL, Gordon LI, Cotter FE. Mantle cell lymphoma and other t(11;14) disorders: How biology can drive therapy. Annual Meeting Educational Book, Chicago: ASCO , 2006, 476–484
- Weigert O, Unterhalt M, Hiddemann W, Dreyling M. Current management of mantle cell lymphoma. Drugs 2007; 67: 1689–1702
- Fisher RI, Bernstein SH, Kahl BS, Djulbegovic B, Robertson MJ, de Vos S, Epner E, Krishnan A, Leonard JP, Lonial S, et al. Multicenter phase II study of bortezomib in patients with relapsed or refractory mantle cell lymphoma. J Clin Oncol 2006; 24: 4867–4874
- Belch A, Kouroukis CT, Crump M, Sehn L, Gascoyne RD, Klasa R, Powers J, Wright J, Eisenhauer EA. A phase II study of bortezomib in mantle cell lymphoma: The National Cancer Institute of Canada Clinical Trials Group trial IND.150. Ann Oncol 2007; 18: 116–121
- Falk MH, Issels RD. Hyperthermia in oncology. Int J Hyperthermia 2001; 17: 1–18
- van der Zee J, Gonzalez Gonzalez D, van Rhoon GC, van Dijk JD, van Putten WL, Hart AA. Comparison of radiotherapy alone with radiotherapy plus hyperthermia in locally advanced pelvic tumours: A prospective, randomised, multicentre trial. Dutch Deep Hyperthermia Group. Lancet 2000; 355: 1119–1125
- Jones EL, Oleson JR, Prosnitz LR, Samulski TV, Vujaskovic Z, Yu D, Sanders LL, Dewhirst MW. Randomized trial of hyperthermia and radiation for superficial tumors. J Clin Oncol 2005; 23: 3079–3085
- Kampinga HH. Cell biological effects of hyperthermia alone or combined with radiation or drugs: A short introduction to newcomers in the field. Int J Hyperthermia 2006; 22: 191–196
- Hettinga JV, Konings AW, Kampinga HH. Reduction of cellular cisplatin resistance by hyperthermia–A review. Int J Hyperthermia 1997; 13: 439–457
- Hettinga JV, Lemstra W, Meijer C, Dam WA, Uges DR, Konings AW, De Vries EG, Kampinga HH. Mechanism of hyperthermic potentiation of cisplatin action in cisplatin-sensitive and -resistant tumour cells. Br J Cancer 1997; 75: 1735–1743
- Bukau B, Weissman J, Horwich A. Molecular chaperones and protein quality control. Cell 2006; 125: 443–451
- Mosser DD, Morimoto RI. Molecular chaperones and the stress of oncogenesis. Oncogene 2004; 23: 2907–2918
- Ghobrial IM, McCormick DJ, Kaufmann SH, Leontovich AA, Loegering DA, Dai NT, Krajnik KL, Stenson MJ, Melhem MF, Novak AJ, et al. Proteomic analysis of mantle-cell lymphoma by protein microarray. Blood 2005; 105: 3722–3730
- Valbuena JR, Rassidakis GZ, Lin P, Atwell C, Georgakis GV, Younes A, Jones D, Medeiros LJ. Expression of heat-shock protein-90 in non-Hodgkin's lymphomas. Mod Pathol 2005; 18: 1343–1349
- Shringarpure R, Catley L, Bhole D, Burger R, Podar K, Tai YT, Kessler B, Galardy P, Ploegh H, Tassone P, et al. Gene expression analysis of B-lymphoma cells resistant and sensitive to bortezomib. Br J Haematol 2006; 134: 145–156
- Weigert O, Pastore A, Rieken M, Lang N, Hiddemann W, Dreyling M. Sequence-dependent synergy of the proteasome inhibitor bortezomib and cytarabine in mantle cell lymphoma. Leukemia 2007; 21: 524–528
- Milani V, Frankenberger B, Heinz O, Brandl A, Ruhland S, Issels RD, Noessner E. Melanoma-associated antigen tyrosinase but not Melan-A/MART-1 expression and presentation dissociate during the heat shock response. Int Immunol 2005; 17: 257–268
- Noessner E, Gastpar R, Milani V, Brandl A, Hutzler PJ, Kuppner MC, Roos M, Kremmer E, Asea A, Calderwood SK, et al. Tumor-derived heat shock protein 70 peptide complexes are cross-presented by human dendritic cells. J Immunol 2002; 169: 5424–5432
- Chauhan D, Li G, Shringarpure R, Podar K, Ohtake Y, Hideshima T, Anderson KC. Blockade of Hsp27 overcomes bortezomib/proteasome inhibitor PS-341 resistance in lymphoma cells. Cancer Res 2003; 63: 6174–6177
- Zaarur N, Gabai VL, Porco JA, Jr, Calderwood S, Sherman MY. Targeting heat shock response to sensitize cancer cells to proteasome and Hsp90 inhibitors. Cancer Res 2006; 66: 1783–1791
- Neckers L, Neckers K. Heat-shock protein 90 inhibitors as novel cancer chemotherapeutic agents. Expert Opin Emerg Drugs 2002; 7: 277–288
- Isaacs JS, Xu W, Neckers L. Heat shock protein 90 as a molecular target for cancer therapeutics. Cancer Cell 2003; 3: 213–217
- Hettinga JV, Lemstra W, Meijer C, Los G, de Vries EG, Konings AW, Kampinga HH. Heat-shock protein expression in cisplatin-sensitive and -resistant human tumor cells. Int J Cancer 1996; 67: 800–807
- Meriin AB, Yaglom JA, Gabai VL, Zon L, Ganiatsas S, Mosser DD, Zon L, Sherman MY. Protein-damaging stresses activate c-Jun N-terminal kinase via inhibition of its dephosphorylation: A novel pathway controlled by hsp72. Mol Cell Biol 1999; 19: 2547–2555
- Kuckelkorn U, Knuehl C, Boes-Fabian B, Drung I, Kloetzel PM. The effect of heat shock on 20S/26S proteasomes. Biol Chem 2000; 381: 1017–1023
- Mattson D, Bradbury CM, Bisht KS, Curry HA, Spitz DR, Gius D. Heat shock and the activation of AP-1 and inhibition of NF-kappa B DNA-binding activity: Possible role of intracellular redox status. Int J Hyperthermia 2004; 20: 224–233
- Pajonk F, van Ophoven A, McBride WH. Hyperthermia-induced proteasome inhibition and loss of androgen receptor expression in human prostate cancer cells. Cancer Res 2005; 65: 4836–4843
- Chen F, Rezavi R, Wang CC, Harrison LE. Proteasome inhibition potentiates the cytotoxic effects of hyperthermia in HT-29 colon cancer cells through inhibition of heat shock protein 27. Oncology 2007; 73: 98–103
- Welch WJ, Suhan JP. Cellular and biochemical events in mammalian cells during and after recovery from physiological stress. J Cell Biol 1986; 103: 2035–2052
- Camps J, Salaverria I, Garcia MJ, Prat E, Beà S, Pole JC, Hernández L, Del Rey J, Cigudosa JC, Bernués M, et al. Genomic imbalances and patterns of karyotypic variability in mantle-cell lymphoma cell lines. Leuk Res 2006; 30: 923–934