Abstract
Purpose: Thermal ablation has been used to manage liver malignancy. This study aimed to assess histological changes in rat liver after microwave ablation (MWA) and to investigate whether thermal damage caused by MWA on surrounding liver tissue enhances the efficiency of liver gene transfer.
Methods: MWA was applied to rat liver, and the pathological tissue and ultrastructural changes were evaluated. Green fluorescent protein (GFP) and Renilla luciferase-expressing plasmids were administered to liver tissues by direct injection. GFP expression in liver tissue was analysed in frozen sections using an inverted fluorescence microscope, and Renilla luciferase expression in target tissue was determined using a luminometer.
Results: Tissue demarcations were observed in liver tissue after ablation, and a transition zone with morphological changes was present between necrotic and normal tissue. Hepatocytes in the transition zone showed decreased numbers of microvilli on cell surfaces and increased extracellular space. GFP expression was observed in the transition zone after MWA and plasmid injection and lasted up to 7 days post-ablation. Both the fluorescence and luminescence levels in the transition zone of the liver tissue were significantly higher than those in the untreated tissue (P < 0.001).
Conclusions: Direct plasmid injection to the liver tissue of the transition zone after MWA can achieve effective gene transfection. These findings provide an experimental basis for exploring MWA-assisted target gene transfer for cancer gene therapy.
Introduction
Liver cancer is one of the most common cancers worldwide. China alone accounts for approximately 50% of total new liver cancer cases and deaths due to the high prevalence of chronic hepatitis B virus infection [Citation1]. Most primary liver cancers that occur worldwide (70–90%) are hepatocellular carcinoma (HCC). To date, surgical resection remains the most effective treatment option for HCC, but <30% of patients qualify for resection, and the recurrence rate is as high as 70% within five years [Citation2]. To maximally preserve liver function, treatments that are both effective and minimally invasive are required, especially for unresectable or recurrent cases.
In recent years, thermal ablation techniques have become promising therapeutic choices for cancer treatment. Various energy sources are used for heating biological tissues such as radiofrequency ablation (RFA) and microwave ablation (MWA), laser-induced thermotherapy (LITT), and ultrasonic (US) ablation. The aim of any ablation technique is to raise the temperature of the lesions to over 50 °C in order to achieve cell death within minutes [Citation3]. Evidence regarding the use of thermal ablation for liver cancer has steadily accumulated [Citation4–6]. MWA, which has been used as a classical thermal ablation method, induces an alternating electromagnetic field in the frequency range of 900–2450 MHz, causing water molecule rotation. Unlike RFA, MWA induces microscopically well-demarcated lesions with no intra-lesional hepatocyte survival [Citation7]. The temperature in the surrounding liver tissue is usually kept lower than 50 °C. The temperature increase affects the fluidity of the phospholipid bilayer, which directly results in changes in membrane permeability. Furthermore, the changes in the cell membrane permeability and fluidity due to the thermal damage could allow macromolecules, such as plasmids, to be transferred into the cytoplasm [Citation8]. The aim of this study was to assess the histological changes in rat liver after MWA and to explore whether the thermal damage to the surrounding liver tissue could enhance the efficacy of gene transfer.
Materials and methods
Animals
Adult male Sprague Dawley (SD) rats, 6 to 8 weeks of age, were obtained from the Animal Institute of the Chinese Academy of Medical Sciences. All animals received appropriate humane care from properly trained professional staff in compliance with both the Principals of Laboratory Animal Care and the Guide for the Care and Use of Laboratory Animals. These studies were approved by the Animal Experimental Ethics Committee of Tianjin Medical University.
Microwave ablation procedures
A KY-2000 microwave apparatus (Kangyou Microwave Energy Application Institute, Nanjing, China) with a frequency of 2450 MHz and a power output of 0–100 W was used for MWA. A coaxial cable was used for transmitting energy from the generator to the applicator. The system was equipped with a MW antenna (14 gauge, 15 mm), which was connected to a water-circulating cooling circuit. For in vivo MWA, rats were anaesthetised (ketamine 100 mg/kg, xylazine 10 mg/kg, i.p.) and shaved in the abdominal area. A midline incision was made in the abdominal wall and the liver was exposed. A MW needle antenna was inserted into the left lateral lobe, and the ablation was initiated by applying power to the MW generator. To determine the optimal MW power, the output power was set at 20, 25, and 30 W, and the ablation lasted for 60 s. All procedures were performed under strict sterile conditions. After the procedures were completed the incision was closed, and the rats were kept separated under normal housing conditions.
Tissue harvesting and histological procedures
Thermal lesions in unrestricted tissue showed an elliptical cross-section in the longitudinal plane due to the cylindrical symmetry of the antenna when operated in unrestricted electromagnetically homogeneous tissue. Liver specimens were harvested along the long and short axes of the thermal lesions using a hollow needle with a diameter of 2 mm that penetrated the lesion (). Histological examination of tissue was performed by an experienced pathologist. The tissue specimens were fixed in 10% formalin for 24 h, dehydrated in ascending grades of ethanol, and embedded horizontally in paraffin. Serial sections were cut at a thickness of 5 μm, dewaxed, dehydrated, and stained with haematoxylin and eosin (H&E). The slides were examined under a light microscope and photographed with a digital camera (Olympus, Tokyo, Japan) to assess the histological changes in the rat liver and to determine the dimensions of the coagulated area according to the different MW power levels.
Scanning electron microscopy
Immediately following MWA treatment, liver specimens were harvested and sliced into 1-mm pieces. After fixation in 2.5% glutaraldehyde in 0.9% normal saline, these specimens were then post-fixed in 1% osmium tetroxide. The samples were freeze-dried and spatter-coated with gold. Observations were made using a Hitachi-su8010 scanning electron microscope (Hitachi, Japan) operating at 1.0 kV.
Plasmid DNA
The gene markers used to detect gene transfer consisted of two DNA plasmids encoding green fluorescent protein (GFP) and Renilla luciferase. The GFP plasmid, which contained a cytomegalovirus (CMV) promoter, was kindly provided by Weidong Li (Tianjin Medical University General Hospital, Tianjin, China). The Renilla luciferase plasmid, which contained a CMV immediate early enhancer/promoter, was provided by the Tianjin General Surgery Institute. Plasmids were replicated in Escherichia coli DH5α cells and purified using a high-purity plasmid kit (Newbioindustry, Tianjin, China). Plasmid DNA was dissolved in TE buffer, and the quantity and quality were assessed by optical density measurements at 260 and 280 nm.
Gene injection
Two different plasmids, encoding GFP and Renilla luciferase, were used in the gene injection experiments. Immediately after MWA, delivered at 25 W for 60 s, plasmid expressing the target gene (a total of 30 μg in 100 μL) was injected into the lesion at three points with a 29-gauge needle to ensure that the diffusion of the plasmid to the margins of the lesion was relatively homogeneous (). We used an equal amount of normal saline (100 μL) as a control. In another control group, a GFP or Renilla luciferase expression plasmid was injected into normal rat liver (i.e. the reference zone). The rats were euthanised at 1, 3, 5, and 7 days, and the livers were harvested (n = 6 for each day).
Figure 2. Schematic diagram illustrating intra-lesional plasmid injection. MW needle antennas were positioned in the left lateral lobe of the rat liver, and MW energy was delivered via the antennas. An oval lesion was generated as indicated, centred with the antennas. A total of 100 μL plasmid solution was injected into the lesion polar close to liver surface at three points 0.2 cm away from the antenna.
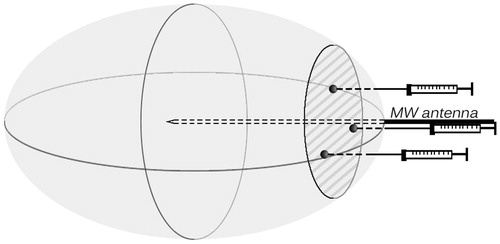
A positive control group was used in which gene transfer into the liver was accomplished by plasmid injection into the portal vein. The rats were anaesthetised before surgery and a midline incision was made to expose the liver. The hepatic medial lobe was inverted to expose the portal vein on the dorsal surface. Plasmid DNA (150 μg dissolved in 2 mL of normal saline per rat) was used at a standard dosage. After temporal ligation of the right gastric vein and the splenic vein, the plasmid was injected into the liver using a 24-gauge needle via the portal vein for 20–25 s; a follow-on ligation of the proximal end of the injection site was performed. All ligatures were released after 120 s. Following immediate compression haemostasis, the rats recovered from anaesthesia and were maintained until euthanasia for liver harvesting.
Evaluation of GFP expression
To assess liver cells that expressed GFP, resected specimens and frozen sections of liver injected with GFP were analysed by inverted fluorescence microscopy. Livers were obtained from 24 rats in each group and examined, with six rats sacrificed per day on post-operative (PO) days 1, 3, 5, and 7. Normal saline-injected livers were used as controls. Resected livers were embedded in optimal cutting temperature (OCT) compound and frozen in liquid nitrogen. Serial sections (5 μm) were cut on a cryostat microtome and placed on glass slides, which were incubated at room temperature for 15 min and fixed in ice-cold acetone for 10 min. For evaluation of transfection efficacy, liver specimens were thawed and homogenised in lysis buffer. Then the homogenate was centrifuged (10,000 rpm ×10 min) to recover the supernatant, which was submitted to another centrifugation. Fluorescence intensity was analysed on a microplate reader (Safire 2, Tecan, Mannedorf, Switzerland) at λEX = 485 nm and λEM = 530 nm.
Luciferase assay
Rats injected with the Renilla luciferase plasmid were examined for liver luciferase activity. The animal groupings and specimen collections were the same as those used for the GFP plasmid. A portion of the specimens, frozen in liquid nitrogen, was pulverised and homogenised in 1× lysis buffer. The homogenate was submitted to three freeze-thaw cycles and centrifuged (10,000 g × 3 min). The resulting supernatant was used for measurements. The protein content was measured using a bicinchoninic acid (BCA) protein assay and was adjusted for each sample before measurement. Luciferase activity was assessed using a luciferase reporter gene assay kit (AAT Bioquest, Sunnyvale, CA) on a microplate reader. Luciferase expression data were expressed as relative light units per mg protein (RLU/mg protein). Experiments were duplicated at least three times for each individual transfected liver.
Statistical analysis
Data are expressed as means ± standard deviations (SD). Statistical analyses were performed using SPSS 17.0 (IBM, Armonk, NY, USA). The data were analysed using the ANOVA. A value of P < 0.05 was considered statistically significant.
Results
Tissue demarcation
Microscopically, the site induced by MWA could be classified into four zones under high-powered magnification (×200) using the same classification system as previously described for LITT [Citation9]. The application zone referred to the tissue directly adjacent to the applicator. The liver architecture was overtly damaged, cellular structure was not visible at the application site, and minimal carbonisation was present (). The central zone was adjacent to the application zone, comprising the main part of the lesion. The hepatocytes within the central zone showed evidence of thermal insult; they appeared shrunken and partly separated from their cell units due to loss of cell contact. Most parenchymal cells underwent coagulative necrosis, with incomplete changes in architecture and nuclear hyperchromatism (). The transition zone, i.e. the surrounding liver tissue, was located between the damaged and undamaged liver tissue and exhibited signs of subacute haemorrhage immediately after MWA. The reference zone corresponded to undamaged liver tissue. In the transition zone, cellular swelling was observed immediately after ablation. The hepatic architecture remained almost unchanged histologically. The transition zone was distinguished by a haemorrhagic border containing numerous erythrocytes (). Various histological changes observed under light microscopy differentiated the transition zone from the other areas.
Figure 3. Histological appearance of liver tissues immediately after microwave ablation. (A) The tissue directly adjacent to the needle during microwave ablation. (B) The central zone consists of damaged liver tissue. (C) Normal architecture and inflammatory cells are observed in the transition zone. (d) Normal liver tissue. Magnification ×200.
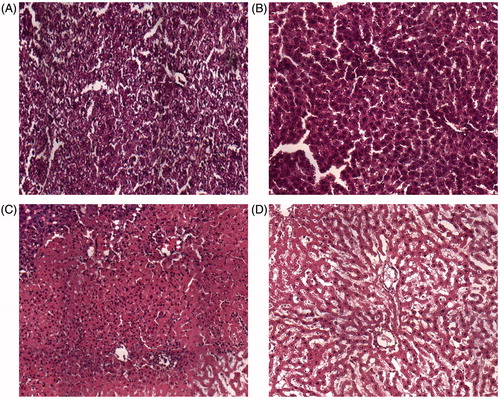
Changes in cell morphology
Scanning electron microscopic observations of hepatocytes in each zone are shown in . Untreated intact hepatocytes had regular orbicular shapes with numerous microvilli on the cell surfaces (), whereas cells in the transition zone presented decreased numbers of microvilli and slightly disrupted cell surfaces. Furthermore, most of cells in this area showed evidence of shrinking, and the endothelial structures adhered to the hepatocytes and exhibited partial damage (). Complete hepatocyte destruction was observed in the central zone ().
Figure 4. Scanning electron microscopy of liver tissue. Liver specimens were collected immediately after microwave ablation at 25 W for 60 s. (A) Normal liver tissue in an untreated area. (B) Hepatocytes in the transition zone. (C) Liver tissue in the central zone underwent coagulative necrosis, and no intact cells were observed.
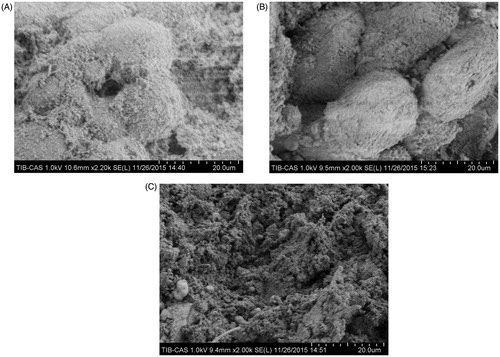
The dimensions of the ablation zone
To determine the optimal MW power to be applied to the rat liver, three different power levels ranging from 20 to 30 W were used. The dimensions of the ablation zones were then measured after 24 and 72 h. At 24 h post-ablation, the mean lengths of the ablation zones (application zone plus central zone) were 1.13 ± 0.16, 1.17 ± 0.10 and 1.78 ± 0.19 cm for 20, 25 and 30 W, and the mean widths were 1.10 ± 0.11, 1.12 ± 0.20, and 1.50 ± 0.11 cm for 20, 25 and 30 W, respectively. At 72 h post-ablation, the mean lengths at 20, 25 and 30 W were 1.13 ± 0.14, 1.33 ± 0.20 and 1.67 ± 0.27 cm, and the mean widths were 1.10 ± 0.16, 1.40 ± 0.18, and 1.6 ± 0.22 cm, respectively. Definite transition zones were apparent in H&E sections in all groups. The results suggested that the dimensions were enlarged with increasing MW power. The MWA applied at 30 W on the left lateral lobe of the rat liver resulted in a larger ablation zone than the ablations applied at 20 and 25 W. Therefore, livers were treated at 25 W for 60 s in the subsequent experiments to avoid extensive liver injury due to high MW energy.
Enhanced transgene expression in the transition zone
To confirm GFP plasmid expression after gene transfer, a group of rats was injected with GFP plasmid via the portal vein, which was considered the positive control group. In rats that underwent MWA, normal saline was used as the negative control. Microscopically, extremely weak fluorescence was detected in the background of whole liver specimens after normal saline injection post-ablation. The fluorescence intensity levels in the transition zone of the intra-lesional injection group, along with the PV injection group, were higher than those of the normal liver injection group. Green fluorescence was high at PO day 3 in both groups and tended to decrease by PO day 5 (). The gene expression levels in the transition zone after intra-lesional injection were markedly higher than those in gene-injected normal liver on both PO day 1 and PO day 3. Furthermore, the mean fluorescence intensity was significantly lower on PO day 1 (10754.2 ± 507.42) than on PO day 3 (24163.3 ± 1522.90, P < 0.001, ).
Figure 5. Fluorescence micrographs of GFP expressed in rat liver from PO days 1–7. Liver specimens were obtained separately for frozen section examination and were assessed under a fluorescence microscope. Magnification ×200.
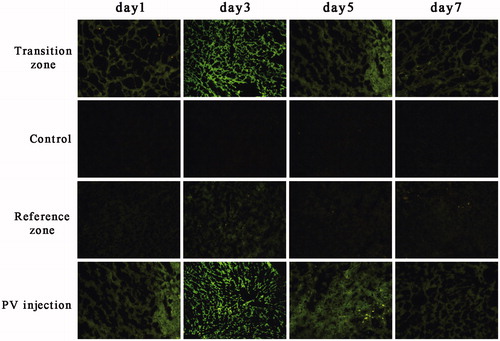
Figure 6. GFP expression after gene transfer. Rat livers underwent microwave ablation followed by immediate gene injection, with fluorescence intensity measured on PO days 1 and 3. Data are expressed as the means ± SDs, and n = 6 for all groups. ***P < 0.001.
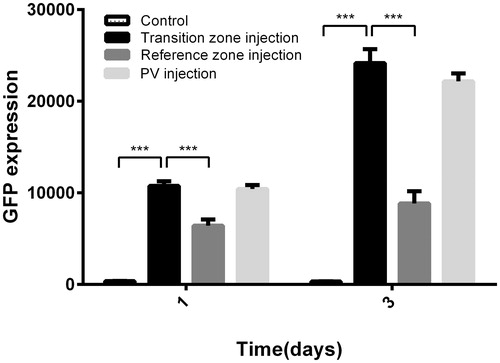
Liver luciferase activity was measured on various days following in vivo transfection. In this experiment, 100 μL of 300 μg/mL Renilla luciferase plasmid was used, and hepatocytes were successfully transfected with this plasmid. At 24 and 72 h post-ablation, gene expression levels in the transition zone were significantly higher than those in the reference zone (P < 0.001); in addition, a high expression level was detected after intra-portal delivery of the plasmid DNA. The luciferase activity in the transition zone was significantly increased compared to the control groups from PO day 1 to PO day 3 and decreased thereafter ().
Figure 7. Luciferase activity in all groups at PO days 1 and 3 (A) and the time course after luciferase plasmid transfer in the transition zone (B). Luciferase expression data are expressed as relative light units per mg protein (RLU/mg protein). Data are expressed as the means ± SD, and n = 6 for all groups. ***P < 0.001.
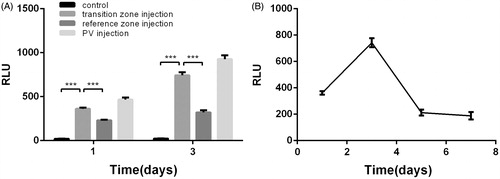
Discussion
Currently, surgical resection is the optimal treatment for primary and secondary malignant liver tumours. It is widely recognised that complete surgical resection of liver tumours prolongs survival in eligible candidates [Citation10]. However, fewer than 30% of liver cancer cases are eligible for resection. Thermal ablation, which can reduce haemorrhage to the maximum extent, has been demonstrated to be a safe and effective treatment for patients with liver metastases [Citation4,Citation10]. The main advantage of MWA is its capacity to create complete coagulative necrosis by heat regardless of the presence of fibrous tissue or a septum [Citation11]. This advantage likely results from the fact that MWA, unlike RFA, does not rely on passage of electric current between electrodes; therefore, it is not impeded by tissue desiccation and charring, both of which decrease electrical conductivity. In addition, no ‘heat sink’ effect or heat loss from adjacent blood vessels occurs, which is the case with RFA; this effect decreases the effectiveness and increases the time required for ablation. However, the surrounding ablative margin, due to the insufficiency of the temperature to kill tumour cells, is likely to increase the risk of residual tumour cells or tumour recurrence.
In our study we treated normal livers in male SD rats with MWA using a power of 20, 25 or 30 W for 60 s. Animals were sacrificed 24 and 72 h post-ablation, and the dimensions of the MW-ablated lesions were evaluated by measuring their lengths and widths in the longitudinal plane. We demonstrated that increased tissue coagulation could be observed over a range of MW power. More importantly, definite transition zones were observed in H&E sections in all groups and showed no differences in thickness. Given that extensive necrosis of the liver tissue may lead to serious complications associated with liver injury, we used a power of 25 W for 60 s as the optimised ablation parameters.
Tissue demarcation was observed after MWA in H&E sections. The transition zone, which contained living hepatocytes, could be differentiated from the other zones microscopically. It has been reported that cell membrane permeability can be altered by application of ultrasound to heat tissues. These effects can increase drug/gene uptake [Citation12,Citation13]. Furthermore, heat stress waves resulted in a transient increase in permeability of the cell membrane without affecting cell viability [Citation14]. Here we investigated the changes in cell morphology post-MWA using scanning electron microscopy. The cells in the transition zone exhibited decreased numbers of microvilli and slight disruptions on the cell surfaces induced by thermal damage, indicating that the effect of the thermal damage might result in high permeability for genetic materials. Therefore we assessed the gene delivery efficacy in the transition zone after the administration of MWA followed by intra-lesional gene injection.
GFP and luciferase were highly expressed in the transition zone, and the expression levels were significantly higher than in the control, in which plasmid was injected into normal hepatic tissue (P < 0.001). We assessed gene expression of the luciferase plasmid for up to 7 days post-treatment. Luciferase levels peaked at PO day 3 before decreasing to relatively low amounts at PO days 5–7. The loss of expression observed following plasmid DNA delivery to the liver was suggested to be a two-phase process; the most important reason for this loss of expression was transcriptional suppression [Citation15]. In the current study we demonstrated that MWA not only resulted in lesion necrosis but also enhanced gene transfer efficacy in surrounding liver tissue after intra-lesional plasmid injection. The potential mechanism for MW-mediated gene transfer involves the localised temperature increase in tissue. The temperature increase affects the fluidity of the phospholipid bilayer, which directly results in altered membrane permeability [Citation8]. Our results regarding the effective expression of genes transferred via portal vein injection are consistent with those of previous studies [Citation16,Citation17]. Although the injected gene could be expressed diffusely throughout the liver, large amounts of plasmid solution were required to be injected into the portal vein under high perfusion pressure, and acute injury to the liver could easily occur. Our findings showed that intra-lesional gene injection post-MWA resulted in effective gene expression at the site of the perilesional zone, where cancer recurrence may take place. Compared to the portal vein injection method, the intra-lesional approach was simpler to perform, and injury to the liver could be minimised.
Some immunostimulatory cytokines, such as interleukins (IL), tumour necrosis factor-alpha (TNF-α), interferon (IFN)-α, IFN-γ, and granulocyte-macrophage colony stimulation factor (GMCSF), are effective in eradicating HCC in mouse and rat models. Many studies have demonstrated that the combination of thermal ablation with intra-lesional injection of cytokines, including IL-2, IL-7 and IL-15, induces enhanced anti-tumour responses [Citation18,Citation19]. We envision that combination therapy with MWA and immunostimulatory cytokine gene administration would result in synergistic effects.
Conclusion
Hepatocytes in the transition zone after MWA were effective targets for gene transfer. Meanwhile, direct plasmid injection was simple to perform compared with portal vein injection, and any liver injury caused by the injection of large amounts of foreign DNA was minimised. These findings provide an experimental basis for exploring the use of MWA-assisted target gene transfer for cancer gene therapy.
Acknowledgements
All authors thank Liwei Zhu and Ning Lu for their thoughtful advice and discussions. We also thank Huanhuan Zhai of the Tianjin Institute of Industrial Biotechnology, Chinese Academy of Sciences for her technical assistance.
Disclosure statement
This work was supported by a grant from National Natural Science Foundation of China (no. 81172167). The authors declare that they have no conflicts of interest. The authors alone are responsible for the content and writing of the paper.
References
- Torre LA, Bray F, Siegel RL, Ferlay J, Lortet-Tieulent J, Jemal A. Global cancer statistics, 2012. CA Cancer J Clin 2015;65:87–108.
- Du Y, Su T, Ding Y, Cao G. Effects of antiviral therapy on the recurrence of hepatocellular carcinoma after curative resection or liver transplantation. Hepat Mon 2012;12:e6031.
- Dewhirst MW, Viglianti BL, Lora-Michiels M, Hanson M, Hoopes PJ. Basic principles of thermal dosimetry and thermal thresholds for tissue damage from hyperthermia. Int J Hyperthermia 2003;19:267–94.
- Vogl TJ, Farshid P, Naguib NN, Darvishi A, Bazrafshan B, Mbalisike E, et al. Thermal ablation of liver metastases from colorectal cancer: radiofrequency, microwave and laser ablation therapies. Radiol Med 2014;119:451–61.
- Dewhirst MW, Landon CD, Hofmann CL, Stauffer PR. Novel approaches to treatment of hepatocellular carcinoma and hepatic metastases using thermal ablation and thermosensitive liposomes. Surg Oncol Clin N Am. 2013;22(3):545–61.
- Li M, Yu X, Liang P, Dong B, Liu F. Ultrasound-guided percutaneous microwave ablation for hepatic malignancy adjacent to the gallbladder. Int J Hyperthermia 2015;31:579–87.
- Bhardwaj N, Strickland AD, Ahmad F, Atanesyan L, West K, Lloyd DM. A comparative histological evaluation of the ablations produced by microwave, cryotherapy and radiofrequency in the liver. Pathology 2009;41:168–72.
- Zhou QL, Chen ZY, Wang YX, Liao YY, Yang F, Lin Y. Ultrasound-mediated local drug and gene delivery using nanocarriers. Biomed Res Int 2014;2014:963891.
- Germer C, Isbert CM, Albrecht D, Ritz JP, Schilling A, Roggan A, et al. Laser-induced thermotherapy for the treatment of liver metastasis. Correlation of gadolinium-DTPA-enhanced MRI with histomorphologic findings to determine criteria for follow-up monitoring. Surg Endosc 1998;12:1317–25.
- Liu Y, Li S, Wan X, Li Y, Li B, Zhang Y, et al. Efficacy and safety of thermal ablation in patients with liver metastases. Eur J Gastroen Hepat 2013;25:442–6.
- Dodd GD III, Soulen MC, Kane RA, Livraghi T, Lees WR, Yamashita Y, et al. Minimally invasive treatment of malignant hepatic tumors: at the threshold of a major breakthrough. Radiographics 2000;20:9–27.
- Saad AH, Hahn GM. Ultrasound-enhanced effects of Adriamycin against murine tumors. Ultrasound Med Biol 1992;18:715–23.
- Rychak JJ, Klibanov AL. Nucleic acid delivery with microbubbles and ultrasound. Adv Drug Deliv Rev 2014;72:82–93.
- Tachibana K, Uchida T, Tamura K, Eguchi H, Yamashita N, Ogawa K. Enhanced cytotoxic effect of Ara-C by low intensity ultrasound to HL-60 cells. Cancer Lett 2000;149:189–94.
- Herweijer H, Zhang G, Subbotin VM, Budker V, Williams P, Wolff JA. Time course of gene expression after plasmid DNA gene transfer to the liver. J Gene Med 2001;3:280–91.
- Song S, Noble M, Sun S, Chen L, Brayman AA, Miao CH. Efficient microbubble- and ultrasound-mediated plasmid DNA delivery into a specific rat liver lobe via a targeted injection and acoustic exposure using a novel ultrasound system. Mol Pharm 2012;9:2187–96.
- Jiang J, Yamato E, Miyazaki J. Intravenous delivery of naked plasmid DNA for in vivo cytokine expression. Biochem Biophys Res Commun 2001;289:1088–92.
- Habibi M, Kmieciak M, Graham L, Morales JK, Bear HD, Manjili MH. Radiofrequency thermal ablation of breast tumors combined with intralesional administration of IL-7 and IL-15 augments anti-tumor immune responses and inhibits tumor development and metastasis. Breast Cancer Res Treat 2009;114:423–31.
- Nakayama J, Urabe A, Toyofuku K, Taniguchi S, Hori Y. Suppression of murine melanoma growth with a combination of microwave hyperthermia and local injection of interleukin 2. Br J Dermatol 1994;130:717–24.