Abstract
Purpose: Doxorubicin (DOX)-loaded phosphatidyldiglycerol-based thermosensitive liposomes (DPPG2-TSL-DOX) combined with local hyperthermia (HT) was evaluated in cats with locally advanced spontaneous fibrosarcomas (soft tissue sarcoma [STS]). The study was designed to evaluate the safety and pharmacokinetic profile of the drug. Results from four dose-levels are reported.
Methods: Eleven client-owned cats with advanced STS were enrolled. Five cats received escalating doses of 0.1–0.4 mg/kg DOX (group I), three received 0.4 mg/kg constantly (group II) and three 0.6 mg/kg (group III) IV over 15 min. HT with a target temperature of 41.5 °C was started 15 min before drug application and continued for a total of 60 min. Six HT treatments were applied every other week using a radiofrequency applicator. Tumour growth was monitored by magnetic resonance imaging (MRI) and for dose level III also with 18F-FDG PET.
Results: Treatment was generally well tolerated and reasons for premature study termination in four cats were not associated with drug-induced toxicity. No DPPG2-TSL-DOX based hypersensitivity reaction was observed. One cat showed simultaneous partial response (PR) in MRI and positron emission tomography (PET) whereas one cat showed stable disease in MRI and PR in PET (both cats in dose level III). Pharmacokinetic measurements demonstrated DOX release triggered by HT.
Conclusion: DPPG2-TSL-DOX + HT is a promising treatment option for advanced feline STS by means of targeted drug delivery. As MTD was not reached further investigation is warranted to determine if higher doses would result in even better tumour responses.
Introduction
Although the reported incidence for feline soft tissue sarcoma (STS) varies considerably it is one of the most common cutaneous neoplasm in cats [Citation1,Citation2]. In the majority, subcutaneous injections provoking abnormal chronic inflammation play an important role in tumorigenesis. Individual genetic characteristics also contribute to the development of the tumour. In some cases, the cause remains unknown. Usually, diagnosis is made through cytologic or histologic examination. Like in human STS, feline STS carry poor prognosis despite the low metastatic risk. This is due to their locally invasive behaviour and poorly defined margins [Citation3], which results in a high relapse rate of 14–50% after surgery alone [Citation4,Citation5]. The risk of relapse is dependent on the surgeon’s experience, the number of previous surgeries, tumour volume and tumour site. Current recommendations still name radical surgery of the primary tumour with margins of at least 3 cm, including deep excision of two muscle layers and bony structures if necessary, as the mainstay of treatment of feline STS [Citation1,Citation6]. Adjuvant or neoadjuvant radiation therapy might prolong the time to local tumour recurrence but a benefit has not been proven consecutively and results in literature are inconsistent [Citation7,Citation8]. Whenever complete excision of the tumour with histologically clean margins is impossible, local long-term control is rare. However, the status of the histologically evaluated margin is a crucial prognostic factor.
In addition to surgery and radiation, systemic chemotherapy is often used as adjuvant treatment option [Citation1]. However, the role of adjuvant chemotherapy has still to be defined as in retrospective series after multimodal treatment no clear benefit could be shown [Citation2,Citation9].
Lack of tumour tropism of classic cytostatic drugs leads to typical dose limiting systemic toxicity as neutropenia, nausea, anorexia, weight loss and organ damage (e.g., myelosuppression, renal failure) in cats. Hence, the application of DOX is limited to a cumulative dose of 180–240 mg/m2 [Citation10–12]. The recommended dose per application is 1 mg/kg although administration of higher doses (25 mg/m2) have been reported [Citation13]. For non-resectable STS treated with DOX (1 mg/kg) or non-thermosensitive PEGylated liposomal DOX (1–1.5 mg/kg), temporary tumour response could be documented in less than 40% of cases [Citation14].
Some studies have reported that liposomal DOX leads to less side effects in comparison to non-liposomal DOX [Citation10]. Unfortunately, no improvement in therapeutic efficacy has been reported [Citation10]. This was also shown in treatment of human malignancies [Citation15] because these nanoparticles lack the ability to selectively deliver the encapsulated drug to the tumour tissue in sufficient concentrations [Citation16].
In humans, adding regional hyperthermia (HT) to chemotherapy in the treatment for STS results in significantly better local progression-free survival and disease-free survival compared with chemotherapy alone [Citation17]. The improvement of the therapy was related to several synergistic factors induced by HT. One is owed to the fact that HT leads to cell death and necrosis by direct thermal toxicity [Citation18]. Selective effects of HT are described in vivo at temperatures between 40 and 43 °C. The characteristically chaotic vasculature of solid tumours and the resulting hypoxic areas within the tumour tissue make tumour cells more sensitive to HT. Furthermore, HT increases drug efficacy by increased perfusion and thereby improved drug uptake but also by increased reactivity of the drug with their cellular target structures [Citation19]. Release of heat shock proteins carrying tumour antigens is mediated by heat-induced necrosis and thought to trigger immune responses [Citation18]. Combining DOX with HT leads to synergistic effects that offer new possibilities in the treatment of high risk STS in humans and dogs [Citation18–20].
An important additional improvement can be achieved by combining local HT and thermosensitive liposomes to achieve intravascular drug release in the tumour [Citation21]. TSL are composed of phospholipids that change from solid gel to liquid ordered phase state at their phase transition temperature (Tm), which directly affects the membrane permeability for encapsulated drugs [Citation22]. To ensure stable drug retention at body temperature combined with a fast drug release under HT, TSL formulations are commonly designed with a Tm between 39 and 42 °C. Effectiveness in the treatment of solid tumours was shown in various rodent studies with distinct TSL formulations [Citation23–25]. Moreover, a TSL formulation with encapsulated DOX was tested in a canine clinical phase I study [Citation20]. The lipid composition was 1,2-dipalmitoyl-sn-glycero-3-phosphocholine (DPPC)/1-palmitoyl-sn-glycero-3-phosphocholine/1,2-distearoyl-sn-glycero-3-phosphoethanolamine-N-polyethylene glycol 2000 90:10:4 (mol/mol) (PEG/Lyso-TSL). The authors reported that toxicity was comparable to that of free (non-liposomal) DOX, apart from hypersensitivity reactions and rare cases of acute death because of liver failure. Furthermore, a tumour response rate of 30% was documented. Currently, a modified formulation is tested in human clinical trials [Citation26–28].
In 2004, we introduced a novel TSL formulation composed of DPPC/1,2-distearoyl-sn-glycero-3-phosphocholine (DSPC)/1,2-dipalmitoyl-sn-glycero-3-phosphodiglycerol (DPPG2) 50:20:30 (mol/mol) (DPPG2-TSL) with long-circulating properties in rats and hamsters [Citation29]. DPPG2-TSL showed superior in vitro stability at 37 °C in serum combined with an ultrafast DOX release at 42 °C compared to PEG/Lyso-TSL [Citation30,Citation31]. Moreover, DPPG2-TSL were stabilised over time at 37 °C in presence of serum, whereas PEG/Lyso-TSL were destabilised under these conditions [Citation32]. Effective local delivery of DOX with DPPG2-TSL (DPPG2-TSL-DOX) into rat tumours was recently shown with distinct small animal heating applicators [Citation33] and was quantified with magnetic resonance imaging (MRI) [Citation34]. Superiority of DPPG2-TSL with encapsulated gemcitabine over free drug was proven in a rat model with implanted STS [Citation24].
Objective of the present clinical trial was to investigate the combination of DPPG2-TSL-DOX with local HT in spontaneous tumours of a non-rodent species. Cats with locally advanced STS were chosen, as DOX has shown to be effective in the treatment of feline STS [Citation10,Citation14]. Dose limiting toxicity of DOX was expected to be reduced by liposomal encapsulation. Moreover, subcutaneous feline STS were supposed to be well heatable to the target temperature of 41.5 °C by using a superficial HT applicator. Consequently, DPPG2-TSL-DOX combined with local HT was hypothesised to provide an effective treatment for spontaneous feline STS. Primary endpoints of the study were to determine the safety, feasibility and pharmacokinetic measurements. As secondary endpoints, tumour volume was recorded and MDT should be determined. Ideally, tumour volume should shrink to such a degree that surgical excision becomes a treatment option again. That way, cats with advanced disease could be offered a multi modal treatment approach with chemotherapy, surgery and curative intent radiotherapy (see Figure 4 “Algorithm of the workup for FISS” in [Citation1]), similar to those of initially resectable STS.
Patients and methods
Official announcement was presented and approved by governmental department (AZ: 55.2-1-54-2531-1-08 and AZ: 55.2.-1-54-2532-154-11). Informed consent documents were signed by the owners.
Patient selection
All cats were privately owned and had histologically confirmed STS. Inclusion criteria for the study were locally advanced cancer and an expected survival time of at least six months. Cats with concurrent diseases (renal or cardiac abnormalities, abnormal thyroxin values, infection with feline leukaemia virus or feline immunodeficiency virus) were excluded. Previous treatment as well as recurrent disease was allowed as long as cats had no pre-treatment wit DOX before.
Study design
The cats were enrolled in three different treatment groups: Group I, consisting of five cats, received escalating doses of 0.1–0.4 mg/kg DOX (DPPG2-TSL-DOX). Treatment started with an initial dose of 0.1 mg/kg of DOX for the first three cycles. Dose level was doubled (0.2 mg/kg) for the next two treatment cycles when it was well tolerated. Finally, another dose increase to 0.4 mg/kg was performed for cycles 6 and 7. Three cats of group II and III achieved 0.4 mg/kg and 0.6 mg/kg, respectively, at each treatment cycles. A minimum of at least three cats per dose level were scheduled. A cautious starting dose of 0.1 mg/kg DOX was elected for group I. This corresponds to one tenth of the regular dose of DOX administered to cats. DPPG2-TSL-DOX was infused intravenously over 15 min as soon as an intratumoral temperature of 41.5 °C was achieved.
The treatment protocol consisted of seven treatment cycles under general anaesthesia every other week with routine rechecks directly before and seven days after each treatment cycle (). The first cycle was without administration of local HT to compare pharmacokinetic measurements of DOX with and without simultaneous HT. ECG, pulse oximetry, capnography, blood pressure and temperature measurements were performed continuously during anaesthesia in order to monitor signs of acute hypersensitivity. No antiallergic agents were administered prophylactically. For anaesthesia cats were premedicated with methadone IV. Induction was performed with IV alfaxalone and maintenance was achieved with isoflurane inhalation.
Table 1. Overview of study protocol for the treatment of spontaneous feline soft tissue sarcoma with thermosensitive liposomal doxorubicin (DPPG2-TSL-DOX) and local hyperthermia
Liposomes
Four independently prepared batches of DPPG2-TSL-DOX were used and characterised as described in detail elsewhere [Citation30,Citation31]. Tools to monitor batch quality have been applied in liposomal analytics to exclude inappropriate batches for in vivo application. In brief, analytical methods have been standardised and validated. Traceable, commercially available reference standards were used whenever possible. Control charts were applied as feedback control system for analytical methods. Out-of-specification parameters were applied to exclude inadequate batches. This ensured that only TSL batches with comparable biophysical characteristics and of sufficient quality entered the present study. Final DPPG2 content in the preparations was 31.7 ± 3.4%. Total lipid and DOX concentration was 24.6 ± 7.8 mM and 3.0 ± 1.2 mM, respectively. The final DOX to lipid ratio was 0.12 ± 0.03 (mol/mol). Dynamic light scattering revealed a vesicle size (z average) of 147 ± 13 nm. All preparations were monodisperse with a polydispersity index of 0.11 ± 0.04. No larger aggregates were detected in the intensity distribution. The zeta potential was – 25.0 ± 2.3 mV. In vitro DOX leakage during one-hour incubation at 37 °C in foetal calf serum was 6.8 ± 3.0%. At 42 °C 96.3 ± 9.5% DOX was released over 60 min. Temperature dependent DOX release kinetics measured in HEPES buffer saline (pH 7.4) at temperatures between 37 and 43 °C showed no significant difference between batches and were comparable to previously published data [Citation31]. DPPG2-TSL-DOX were stored at −20 °C prior to application. Since DPPG2-TSL-DOX could be stored for at least 12 months at −20 °C without signs of decomposition (data not shown), batches were used up to 6 months to ensure that DPPG2-TSL-DOX are still within specification.
Patient evaluation
The goal of the study was to investigate practicability and feasibility of simultaneous administration of DPPG2-TSL-DOX and local HT. Assessment of safety and evaluation of intratumoral temperatures as well as pharmacokinetic measurements were primary end points. Efficacy has been evaluated as secondary end point.
In order to document general condition and toxicity data, as well as to assure ability to undergo anaesthesia, physical examination, CBC, clinical chemistry and urinalysis were done on each treatment day. Additional CBC and clinical chemistry were analysed seven days after each treatment cycle. During anaesthesia cats were monitored for acute hypersensitivity reactions by electrocardiogram, indirect blood pressure measurement, pulse oximetry, capnography and temperature probes. Cardiac ultrasound and ECG were performed at the time of enrolment and after the last treatment cycle.
Toxicity data were evaluated according to the Veterinary Cooperative Oncology Group guidelines [Citation35]. Differentiation was made between local and systemic adverse events. Treatment associated toxicity was differentiated from non-treatment related side effects. In concordance with the official guidelines for regional HT [Citation36], side effects were classified as either acute (during therapy), subacute (up to 6 months after therapy) or late (more than 6 months after therapy).
Hyperthermia administration
All treatment groups obtained local HT with a tumour target temperature of 41.5 °C over 60 minutes simultaneously to DOX administration, by means of a superficial radiofrequency applicator (MA-151, BSD Medical, UT, USA, ). The frequency of the radiofrequency applicator was 915 MHz with a maximum power of 100 Watts. A Bowman temperature probe was inserted in the centre of the tumour. Probe insertion was planned on the basis of MRI or positron emission tomography (PET)/MRI and without online imaging. Preservation of the skin above the tumour was provided by a circulating deionised water cooling system. Additional rectal and oesophageal Bowman thermometry probes measured body temperature. One invasive sensor controlled the intratumoral temperature and a superficially placed sensor the temperature on the skin. The invasive probe was moved manually along a catheter track to obtain a temperature profile across the catheter path. The temperature was recorded once per minute. The power heating of the tumour tissue was adapted automatically based on the recorded intratumoral temperature in order to maintain a constant tumour temperature. The feedback loop of the applicator used a medium target temperature of 41.5 °C. If this temperature was exceeded for a minute than the power was reduced. Therefore, short periods of higher temperatures were possible.
Pharmacokinetic analysis
Plasma samples for pharmacokinetic examination of DOX were collected before administration of DPPG2-TSL-DOX and HT (time 0), and at 7, 15, 30, 45, 60, 75, 105 and 135 min after starting the liposome infusion when intratumoral target temperature was reached. At each time point, one ml of blood was collected via an indwelling venous catheter into an EDTA-containing tube (Multivette 600, Sarstedt, Germany). The catheter was placed either into the cephalic or the femoral vein. The samples were centrifuged at 4000g for 5 min at 4 °C within 30 min after blood withdrawal and frozen at −20 °C until the time of analysis.
Concentration of DOX in plasma was quantified with high performance liquid chromatography (HPLC) as described previously [Citation33] and was the sum of free and liposomal DOX. Nevertheless, the measured values mainly contained liposomal encapsulated drug, since free DOX is either rapidly taken up by the tissue or metabolised [Citation37]. The theoretical maximum plasma concentration for DOX after IV injection (ID) was calculated by assuming that the total plasma volume of a cat is 4% of the body weight [Citation38]. For example, injection of 0.1 mg DOX per kg bodyweight results in an ID of 2.5 μg DOX per ml plasma. Therewith, the measured plasma concentration of DOX was expressed as percentage of the maximum concentration (%ID) and fitted from t = 15 min (end of infusion period) to t = 135 min to a two compartment pharmacokinetic model (OriginPro 8.1.5, OriginLab Corp., MA, USA):**
(1)
where kα and kβ denote the rate constants of elimination. The corresponding plasma half-lives were calculated with EquationEquation. (2)
(2) :
(2)
The area under the curve (AUC0–135 min) was obtained by integrating the measured DOX plasma concentration expressed as μg/ml from t = 0 to t= 135 min.
Tumour response
In order to evaluate tumour response, three different methods to determine tumour volume were compared. In cats of treatment group I and II, manual calliper measurement and MRI were performed. In group III, additional 18F-FDG PET/MRI was used. Tumour response was classified according to modified RECIST criteria for solid tumours [Citation39], volumetry [Citation40,Citation41] and PERCIST [Citation42]. As in previous studies [Citation16], tumour volume was calculated with EquationEquation 3(3) :
(3)
In cats with tumour sizes extending the size of HT applicator (# 4) target lesions were determined. Because HT here is a locally applied therapy, only one target lesion and no secondary or new lesions were included in the evaluation (# 7).
Standardised calliper measurements were performed before every treatment cycle and on each recheck seven days later. MRI measurements were done by a board certified radiologist before the first and after the last treatment cycle. The same imaging setup, MRI system and sequence protocol was used for both MRI examinations of a single animal. With both methods, three dimensions of the tumour or target lesion were determined.
In group III 18F-FDG PET/MRI measurements were performed before the first, after the second and after the last treatment cycle. The additional measurement after the second treatment cycle was used to evaluate early treatment response. Therefore, SUVpeak mean was determined for metabolic response assessment.
Imaging
Thoracic radiographs and abdominal ultrasound were performed to exclude metastatic disease whereas MRI was performed to assess the tumour response. Imaging after completion of treatment protocol was performed within 8–22 days after the last treatment cycle. Median period of time between the last treatment cycle and reimaging was 16 days. MRI was carried out at 1.5 Tesla (Magnetom Symphony, Siemens Healthcare, Germany) (# 1–8) and 3.0 Tesla (PET-MRI Biograph mMR, Siemens Healthcare, Germany) (# 9–11). For MRI measurements, a standardised protocol including T2- weighted imaging pre contrast media injection and T1-weighted imaging pre and post contrast media and with and without fat suppression was established. Images were acquired in axial and sagittal slice orientation with 3 mm slice thickness. Orthogonal maximum tumour diameters in three dimensions were determined in contrast agent enhanced T1-weighted images.
For PET/MRI 18FDG uptake in normal tissue (muscle, lymph node) and suspected tumour tissue area was compared. Tumour tissue was determined as volume of interest for semi quantitative evaluation. Maximum standardised uptake value (SUVmax), mean SUV value (SUVmean) and metabolically active tumour volume was measured. Tumour tissue was defined by the voxels exceeding the SUV threshold values. To determine an additional semi quantitative parameter SUVpeak was measured. Therefore, the hottest point within the tumour tissue was determined as a 1 cm radius and named region of interest (ROI). Mean SUV of this ROI was recorded as SUVpeak mean.
Maximum tolerated dose
Hematological dose limiting toxicities included ≥ grade 3 thrombocytopenia, febrile neutropenia or neutropenia ≥grade 3 or ≥14 days in duration, ≥grade 4 anaemia. Non-hematological dose limiting toxicities included ≥ grade 2 hypersensitivity or any other toxicity > grade 3.
Results
Patient demographic and baseline characteristics
Between June 2012 and May 2013, eleven privately owned cats, four neutered males and seven spayed females, with a mean age of 12 years (range: 8–14 years) and mean weight of 4.4 kg (range: 3.5–7.1 kg), with histologically confirmed STS were enrolled in the study (). Six of the cases were primary tumours while five were recurrent disease. Previous therapy in cats that presented with recurrent disease consisted of surgery. One of them also had received post-operative radiation therapy. No previous treatment with chemotherapeutic drugs was reported. Tumour site was interscapular (n = 6), lateral chest wall (n = 3, next to the proximal extremity in # 8) and the lumbar region (n = 2). Three-view thoracic radiographs and abdominal ultrasound did not reveal metastatic lesions in any of the cats.
Table 2. Baseline characteristics of all cats with locally advanced soft tissue sarcoma assigned to treatment with liposomal doxorubicin (DPPG2-TSL-DOX) and simultaneously administered local hyperthermia.
Study treatment
Seven cats (# 1, 4, 6, 7, 9, 10 and 11) received the treatment protocol as scheduled including one cycle without HT for measurement of pharmacokinetic profiles without HT and six treatment cycles of DPPG2-TSL-DOX combined with local HT. Four cats terminated the protocol earlier.
Study cats received a total of 50 cycles of DOX (DPPG2-TSL-DOX) and simultaneous local HT (one cat received one cycle, two cats received two cycles, one cat received three cycles and seven cats received six cycles).
Safety
Mild to moderate systemic toxicity was recorded (). Neutropenia was documented in one cat (# 8) and thrombocytopenia in four cats (# 3, 5, 10, 11). Eight cats out of 11 showed anaemia (# 1–3, 5–9). More than half of the cats lost weight (# 3, 5–7, 9, 11) during the course of the study while only three cats of these showed reduced appetite (# 5, 6, 11). Application of liposomes was well tolerated in all cats.
Table 3. Toxicity data. Classification and grading of adverse events.
Local side effects as mild oedema and skin burn, and moderate inflammation following pruritus (# 4) occurred in two cases (# 1, 11). However, one cat of treatment group I (# 5) and one cat of group II (# 8) developed severe tumour necrosis and ulceration. In the letter case, limb amputation was necessary. The other cat was euthanized due to additional problems like tumour progression and tumour cachexia.
Intratumoral temperature
The characteristic temperature values of the HT treatment cycles are shown in . Median temperature within the tumour (T50) was determined for each cat during each cycle. Administered T50 over all cats and all treatment cycles was 41.7 ± 0.6 °C. During the first treatment cycle of all cats when DPPG2-TSL-DOX was given in combination with local HT (n = 11), a T50 of 41.5 ± 0.5 °C was recorded. Similarly, a T50 of 42.4 ± 1.2 °C was achieved for the last treatment cycle (n = 7). Identification of T90, the temperature at which 90% of the recorded values are equal or greater resulted in 40.3 ± 0.9 °C for all treatments. For all of the first combined treatment cycles the T90 was 40.7 ± 1.2 °C and for the last T90 = 41.5 ± 1.3 °C, respectively. Median time to achieve the target temperature was 8 min (range: 6–9 min). No elevation of body temperature during local HT was documented.
Table 4. Intratumoral temperature values of cats with locally advanced soft tissue sarcoma treated with liposomal doxorubicin (DPPG2-TSL-DOX) and simultaneously administered hyperthermia.
Pharmacokinetic results
summarises the pharmacokinetic parameters of DOX encapsulated in DPPG2-TSL in the cats. A huge variation between individual cats was observed especially at the lowest dose level (). Coefficient of variation (CV) was 51.5%, 58.7% and 73.7% for Cmax, %ID15min, and AUC1–135 in cats receiving 0.1 mg/kg DOX without HT, respectively. In contrast, CV was 28.4%, 25.0% and 35.0% in cats receiving 0.4 mg/kg DOX without HT, respectively. Increasing the dose of DOX in cats also resulted in an increase in calculated pharmacokinetic parameters. Cmax, tα and AUC1–135 min increased 7.1 fold, 1.8 fold and 8.5 fold, respectively, when the dosage was increased four-fold from 0.1 to 0.4 mg/kg DOX without HT.
Figure 2. Pharmacokinetic profiles of DOX after application of DPPG2-TSL-DOX. (A) DOX plasma concentration in cats receiving no local hyperthermia treatment. A huge variation in the plasma concentration of DOX was observed in the lower dose level (0.1 mg/kg), that was not observed in the higher dose level (0.4 mg/kg). (B) DOX plasma concentration in one representative cat treated without (open diamonds) local hyperthermia in the first treatment cycle and with (closed diamonds) local hyperthermia in the second cycle.
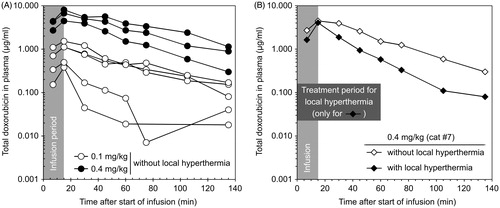
Table 5. Pharmacokinetic parameters of doxorubicin encapsulated into DPPG2-TSL obtained from cats with locally advanced soft tissue sarcoma.
Comparison between plasma DOX concentrations in cats treated with and without application of local HT clearly demonstrated the effect of HT on triggered drug release from the liposomes (). Plasma DOX concentration showed a mono-exponential or bi-exponential elimination over time in cats treated without or with local HT, respectively. In this context, tα decreased when cats were treated with HT. For example, tα was 22.5 ± 13.2 min for cats receiving 0.1 mg/kg DOX without HT and decreased to 10.3 ± 5.1 min with HT, respectively.
Efficacy
Tumour response data are shown in .
Table 6. Tumour response in cats with locally advanced soft tissue sarcoma treated with thermosensitive liposomal doxorubicin (DPPG2-TSL-DOX) at four different dose levels and simultaneous local hyperthermia.
Calliper measurements: Mean tumour volume before start of treatment was 63 cm³ (median of 38 cm³; range: 4 cm³ (# 8) to 260 cm³ (# 1)). For group I, mean tumour volume was 98 cm³ (42 cm³; 38–260 cm³), whereas these values were 17 cm³ (17 cm³; 4–30 cm³) in group II, and 51 cm³ (66 cm³; 22–107 cm³) in group III. In four cats, treatment had to be terminated earlier. In three cats, discontinuation was indicated clinically because of progressive tumour growth (# 3, 5) or tumour ulceration and tissue necrosis (# 8). In the fourth case (# 2) the owner withdrawn consent. In 1 cat (# 10), partial response (PR) (79% reduction in tumour volume) was achieved, five cats had stable disease (8%, 24%, 38%, 46%, 53% reduction in tumour volume in # 1, 6, 9, 7 and 4, respectively) and 1 cat (# 11) that finished the protocol showed progressive disease (174% increase in tumour volume). In three cats (# 1, 6 and 10) surgical excision after the full treatment protocol was possible because tumour size was reduced compared to the initial tumour volume.
MRI evaluation: Results of the tumour response evaluation in MRI showed initially a mean tumour volume of 78 cm³ (52 cm3; 3.6 cm³ (# 8)–318 cm³ (# 1)). For group I, mean tumour volume was 122 cm³ (75 cm³; 20–318 cm³), whereas these values were 18 cm³ (17 cm³; 4–34 cm³) in group II, and 79 cm³ (66 cm³; 52–118 cm³) in group III. One cat showed partial remission (69% reduction in tumour volume, # 10), two cats stable disease (no change in tumour volume # 7 and 26% increase in tumour volume, # 1) and four progressive disease (47%, 109%, 230%, 500% increase in tumour volume, # 6, 9, 11, 4). Cat # 5 could not be evaluated by MRI due to artefacts induced by a microchip located near the tumour site.
In all cats in dose level III treatment response was evaluated using 18F-FDG PET/MRI. According to PERCIST [Citation42], two cats (# 9, 10) showed a metabolic PR with 31% and 34% decrease in SUVpeak mean after treatment, respectively. In cat # 10, tumour volume was also reduced by 69% in MRI (). After DPPG2-TSL-DOX treatment the tumour of this cat was removed surgically. Histopathologic examination of the tumour revealed extensive tumour necrosis in the tissue close to the applicator. Viable tumour cells were detected on the opposite tumour side farthermost distant of the HT applicator. This pattern correlated well with the penetration depth of the microwave applicator which is approximately 2.5 cm (). Cat #11 showed a slight decrease in SUVpeak mean from 8.9 to 8.0 which corresponds to metabolic stable disease.
Figure 3. (A) # 10: PET/MRI fusion images demonstrating tumour response after two and after six treatments with DOX 0.6 mg/kg in DPPG2-TSL-DOX. (B) Macroscopic view and microscopic pictures (HE, ×50 magnification) of the subsequently resected tumour with complete necrosis and chronic inflammation (above) and residual malignant spindle cells (bottom). Complete tumour necrosis in the tumour part near to the applicator indicating insufficient heating in the basal part.
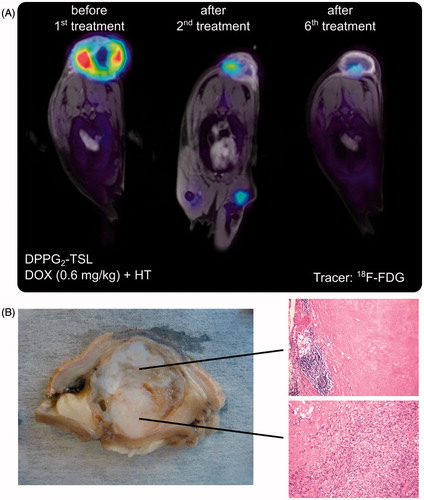
Discussion
Practicability and feasibility
Treating feline STS with DPPG2-TSL-DOX and simultaneous local HT was proven to be safe and feasible. In addition, this study showed tumour response in some cats even at low dose levels. The efficacy of this treatment was not within the scope of this study, but promising preliminary results have been seen and further studies are needed to evaluate treatment response. Temperature data demonstrated adequate tumour heating in all cats [Citation36]. Referring to the values for T90 and knowing the fast drug release from DPPG2-TSL-DOX in vitro at temperatures >39 °C, maximum intravascular drug release in the tumour was assumed. However, not all cats showed a decrease in tumour volume. Limited assessment of temperature distribution within the tumour might play an important role. By using a single temperature probe in the centre of the tumour, a large volume of the tumour still remains unevaluated. As feline STS tend to be heterogeneous in texture it can be assumed that temperature data recorded along the path of the probe did not represent the heat distribution throughout the whole tumour volume. In order to minimise the risk of tumour ulceration, as a common complication, implantation of additional temperature probes was avoided. Non-invasive 3D thermometry (e.g., by MRI) could overcome this limitation. Dedicated MRI HT hybrid systems have been developed to optimise temperature recording over the entire tumour volume during HT but were not available for our study.
Toxicity
Only a few of the documented toxicity events were considered to be related to either DPPG2-TSL-DOX administration and/or local HT (). No treatment associated systemic side effects were observed. The cytopenia seen in cases # 3 and # 8 and the anaemia seen in several cats were suspected not to be related to STS therapy as e.g., cat # 8 already showed neutropenia grade 1 at the time of initial examination. Development of grade 3 thrombocytopenia was documented in cat # 3 with concurrent tumour progression and worsening of general condition after the second treatment cycle. Either immune-mediated origin or intratumoral haemorrhage was assumed in this case, rather than myelosuppression. However, no further examination was pursued. Decreased platelet counts in cat # 5, 10 and 11 were supposed to be caused by technical problems that occurred during the blood drawings as cats in comparison to dogs show a higher tendency of aggregate formation. However, no further investigation was performed. Eight cats showed decreasing haematocrit and haemoglobin values during therapy. Grade 1 and 2 anaemia was typically seen. Regenerative and non-regenerative anaemia was documented. Since the largest drop in haematocrit occurred after MRI anaesthesia before starting the treatment in more than half of the study population (# 1, 2, 4 (drop within reference range), 5, 6), no association with DPPG2-TSL-DOX or HT was present. At this time point the cats had neither been treated with DPPG2-TSL-DOX nor with HT. Manifestation of the myelosuppressive effect of DOX would be expected around the life span of feline erythrocytes, which is about 70 days. In cats with regenerative anaemia, neither evidence of haemorrhage nor of haemolysis was present. Heinz body anaemia might have been a plausible cause in # 3, 7, 8 and 9. Oxidative damage to erythrocytes by alfaxalone cannot be excluded although this has not been reported so far. For non-regenerative anaemia, the effect of chronic disease also has to be taken into consideration. Subclinical gastrointestinal haemorrhage was unlikely as the mean corpuscular volume was unremarkable. No clinical signs of anaemia were observed. The majority of cats showed normal haematocrit values at the control visits four weeks after the end of treatment. DPPG2-TSL-DOX every other week showed notably less toxicity than classic DOX which has been reported to cause in up to 20% of the cats anorexia and in 11% vomiting if applied in a three weeks schedule [Citation13].
For six of the cats (# 3, 5–7, 9, 11) weight loss during the time of therapy was documented. Progressive tumour growth and worsening of general condition were reported together with continuously reduced appetite in # 3, 5 and 11. Cat # 6 was known for stress induced hyporexia before, whereas the origin of weight loss remained unknown in case # 7 and # 9. Increased stress level related to the regular veterinary visits might have led to increased calorie consumption. Anorexia as a side effect of chemotherapy typically occurs within the first days after DOX administration and is self-limiting or responsive to antiemetic treatment.
No acute toxicity caused by the application of liposomal DOX which has been described previously in humans [Citation43], dogs [Citation20] and cats [Citation10] was recognised. Premedication with corticosteroids or antihistamines was neither performed nor necessary.
Local side effects within the treatment field of HT were acceptable. Licking and scratching was often reported in association with tumour growth and in one cat (# 5) promoted development of skin necrosis. Cat # 8 showed tissue necrosis without any sign of automutilation. The very small tumour size and the atypical localisation on the skin fold behind the elbow might have led to undetected high temperatures in the healthy tissue surrounding the tumour. Additionally, changes in tissue characteristics (e.g., impaired wound healing) due to previous radiation therapy in this area might have caused tissue damage as this is well-documented in human medicine [Citation44]. Even though repeated probe implantation represents an extra risk for bacterial infection, this was excluded by bacterial culture of a swab.
Pharmacokinetic measurements
As expected from results with gemcitabine encapsulated in DPPG2-TSL in rodents [Citation24], the pharmacokinetic profile of DOX in cats receiving no HT treatment showed mono-exponential clearance (, ). With addition of HT, clearance became bi-exponential (, ), indicating heat-triggered, intravascular drug release in the tumour blood vessels. The effect of HT on DOX release was clearly demonstrated by a technical malfunction when tumour heating was interrupted accidentally and the drop of plasma DOX level decreased until the tumour was heated again (data not shown). Highest plasma DOX concentration (Cmax) was usually measured after 15 min corresponding with the end of the infusion of DPPG2-TSL-DOX and which therefore correlated with %ID15min. The continuous infusion over 15 min might be the reason why %ID15min never corresponded to the initially administered drug dose. Increasing DPPG2-TSL-DOX dose consistently resulted in higher plasma concentrations, tα, and AUC1–135 min in all cats. Although in group I the dose was increased fourfold, AUC1–135 min increased by the factor 8.5, which can be explained by limited absorption capacity of the mononuclear phagocytic system [Citation45]. Extended plasma concentration and prolonged tα is measured when this capacity is exceeded resulting in an increased AUC.
The observed huge difference in the pharmacokinetic profile between cats receiving the lowest dose was previously shown by others with a PEGylated liposomal drug formulation [Citation46]. The authors correlated this difference to the age and body composition of the cat [Citation46].
Dose escalation and maximum tolerated dose
As this was a real patient study with cats from private owners, a very low and presumably safe dose of 0.1 mg/kg DPPG2-TSL-DOX was elected as starting dose in the first cats. Results from group I indicated that tumour response would only be achieved with higher dosages. No systemic side effects occurred. In order to protect large number of cats from being treated at insufficient low doses an intraindividual dose escalation was performed until 0.4 mg/kg DPPG2-TSL-DOX was reached. Although local toxicity was recorded at 0.4 mg/kg and 0.6 mg/kg (# 1, 4, 5, 8, 11), it is unclear whether this represents dose-limiting side effects. In addition, differentiation between side effects due to HT itself and intratumoral DOX accumulation by HT is difficult to assess at that time. As no treatment related relevant systemic toxicities during this first phase were observed the dose level 0.4 mg/kg was repeated in three previously non-treated cats at a constant dose level (group II). This was done to detect possible cumulative toxicities at this particular dose level. Again, as no relevant toxicities at this dose level were recorded, three additional cats have been treated with 0.6 mg/kg DPPG2-TSL-DOX. In summary, there was one group at the start of the study where an intrapatient dose escalation was performed. Additional study groups following the classical dose regime of a phase I study will be investigated subsequently. Further data from treatment group II and III and their similar toxicity pattern showed that MTD for DPPG2-TSL-DOX was not reached in this study. According to another study [Citation45], MTD of encapsulated drugs has to be assumed to be similar to the dose of its corresponding free formulation. For DPPG2-TSL-DOX MTD still has to be investigated.
Efficacy
Tumour response did not represent a primary end point in this study. As expected, manual and MRI measurements of tumour volume to assess response varied widely. MRI documented tumour response as follows: SD in 2 cats (# 1, 7) and PD in 4 cats (# 4, 6, 9 and 11). Considering the overall response, cat # 1, 4, 6, 7 and 9 were recorded as responders according to manual measurements on the contrary. In MRI and PET/MRI tumour growth was observed especially in areas of the tumour that were not covered by the HT applicator because of their large size or extension into the depth (# 3, 4, 6, 11). This confirmed the importance of imaging for designing a suitable treatment regime and maximising therapeutic success, as other studies already reported [Citation3]. EquationEquation (3)(3) could be an additional source of error in assessment of tumour volume, as in general the real tumour geometry corresponds poorly to ellipsoid shape. In this study, most tumours appeared irregular formed and heterogeneously structured consisting of vital tissue, cystoid and necrotic structures. A single maximum diameter (RECIST) may also not be sufficient to characterise these tumours. Volumetric analysis by delineating the tumour on the MRI images could improve correlation with real tumour size.
In the present study, no other parameters than tumour volume were documented for the whole study population to monitor tumour response. Since tumour necrosis, perfusion and diffusion as additional parameters [Citation47] were not taken into account, tumour response might have been underestimated. To overcome this limitation, we used 18F-FDG PET/MRI for assessment of tumour metabolism in cats of group III to evaluate tumour response. The data showed clear metabolic response via 18F-FDG PET/MRI already after the second treatment cycle in two cats (# 9, 10).
The follow up data were recorded for all cats, even so this was not an endpoint of the study.
After completion of the protocol, owners were offered to choose standard treatment for feline STS. The standard therapy for feline STS at our hospital includes surgery followed by curative or palliative radiation depending on the margins evaluated by histological examination.
Due to owners’ decision, seven cats received no further treatment (# 2–5, 7, 9, 11). In this group, three cats (# 3, 5, 11) had progressive disease and prognosis even with treatment was guarded. Three cats (# 6, 8, 10) had surgery after completion of the study. Overall survival of cats receiving surgery alone was 11 months. Out of this group, one cat survived 19 months.
One owner (# 1) decided for surgery followed by curative radiation (15 fractions at 3.5 Gy, total dose: 52.5 Gy). Thirty months after completion of therapy, this cat had a recurrent STS at the treatment side. After an additional surgery, the cat was irradiated with a palliative intent radiation (five fractions at 6 Gy, total dose: 30 Gy). Ten months later the cat had to be euthanized due to the diagnosis of renal cancer.
Including cats with advanced disease was a limitation of the study. Therefore, the size of the HT applicator was insufficient in relation to tumour size in some cats (# 1, 3, 4, 5, 11). Furthermore, recurrent STS are known to behave more aggressive than primary tumours and should ideally be excluded to optimise evaluation of tumour response. With the MA-151 surface applicator, a penetration depth of heat of about 3 cm into tissue can be achieved and thus, ideally, tumours with a maximum size of 3–4 cm in diameter should be treated. This was confirmed by shrinkage of tumour volume or reduction of SUVpeak mean in cat # 6, 7, 9 and 10 on MRI/PET/MRI and by comparison of the tumour tissue in the depth in cat # 3, 5, 6, 7. The cats included in the study showed great variation in both, tumour size and location. This heterogeneity is suspected to be the reason why correlation of tumour response and DPPG2-TSL-DOX dose was not possible. Efficacy could not be confirmed in this treatment group, although the first results are promising. However, the priority was to investigate feasibility, compatibility of the intervention and PK profile of DPPG2-TSL-DOX. Efficacy was assessed as a secondary endpoint.
Conclusion
Doxorubicin (DOX) encapsulated into DPPG2-based thermosensitive liposomes (DPPG2-TSL-DOX) combined with local HT was proven to represent a feasible, safe and a promising treatment option in feline STS. Heating of tumour tissue to 41.5 °C was easily achieved, although heterogeneous tissue composition was challenging. Local and systemic toxicity in general only occurred to a mild degree. Previous radiation therapy should be avoided to minimise the risk of severe skin toxicity. Even in the highest dose group of 0.6 mg/kg no systemic side effects were recorded. Plasma measurements of DOX concentration over 135 minutes demonstrated heat-triggered local liposomal drug release.
Very promising therapeutic effects with tumour stabilisation for doses of 0.2 mg/kg and objective tumour responses for doses of 0.6 mg/kg were seen, showing the strong potential of DPPG2-TSL-DOX in combination with local HT for the treatment of feline STS in advanced stage of disease. Further studies at higher doses and with optimised technical equipment and control cohorts are warranted urgently to investigate efficacy of this novel treatment option and to determine potential transmission to human STS patients as well.
Acknowledgements
The authors thank Angela Knauerhase, Lisa Pointner, Kirsten Wachholz, Linus Willerding, Anja Zengerle at the University Hospital of Munich for technical support and Dr. Sennewald GmbH (Munich, Germany) for providing the HT system.
Disclosure statement
Lars H. Lindner and Martin Hossann hold shares of Thermosome GmbH, Planegg/Martinsried, Germany. All other authors declare no conflict of interest.
References
- Martano M, Morello E, Buracco P. (2011). Feline injection-site sarcoma: past, present and future perspectives. Vet J 188:136–41.
- Ladlow J. (2013). Injection site-associated sarcoma in the cat: treatment recommendations and results to date. J Feline Med Surg 15:409–18.
- Zardo KM, Damiani LP, Matera JM, et al. (2016). Recurrent and non-recurrent feline injection site sarcoma: computed tomographic and ultrasonographic findings. J Feline Med Surg 18:773–82.
- Romanelli G, Marconato L, Olivero D, et al. (2008). Analysis of prognostic factors associated with injection-site sarcomas in cats: 57 cases (2001–2007). J Am Vet Med Assoc 232:1193–9.
- McEntee MC, Page RL. (2001). Feline vaccine-associated sarcomas. J Vet Intern Med 15:176–82.
- Phelps HA, Kuntz CA, Milner RJ, et al. (2011). Radical excision with five-centimeter margins for treatment of feline injection-site sarcomas: 91 cases (1998–2002). J Am Vet Med Assoc 239:97–106.
- Davidson EB, Gregory CR, Kass PH. (1997). Surgical excision of soft tissue fibrosarcomas in cats. Vet Surg 26:265–9.
- Eckstein C, Guscetti F, Roos M, et al. (2009). A retrospective analysis of radiation therapy for the treatment of feline vaccine-associated sarcoma. Vet Comp Oncol 7:54–68.
- Cohen M, Wright JC, Brawner WR, et al. (2001). Use of surgery and electron beam irradiation, with or without chemotherapy, for treatment of vaccine-associated sarcomas in cats: 78 cases (1996–2000). J Am Vet Med Assoc 219:1582–9.
- Poirier VJ, Thamm DH, Kurzman ID, et al. (2002). Liposome-encapsulated doxorubicin (Doxil) and doxorubicin in the treatment of vaccine-associated sarcoma in cats. J Vet Intern Med 16:726–31.
- O'Keefe DA, Sisson DD, Gelberg HB, et al. (1993). Systemic toxicity associated with doxorubicin administration in cats. J Vet Intern Med 7:309–17.
- Kent MS. (2013). Cats and chemotherapy: treat as 'small dogs' at your peril. J Feline Med Surg 15:419–24.
- Reiman RA, Mauldin GE, Neal Mauldin G. (2008). A comparison of toxicity of two dosing schemes for doxorubicin in the cat. J Feline Med Surg 10:324–31.
- Barber LG, Sørenmo KU, Cronin KL, Shofer FS. (2000). Combined doxorubicin and cyclophosphamide chemotherapy for nonresectable feline fibrosarcoma. J Am Anim Hosp Assoc 36:416–21.
- O'Brien ME, Wigler N, Inbar M, et al. (2004). Reduced cardiotoxicity and comparable efficacy in a phase III trial of pegylated liposomal doxorubicin HCl (CAELYX/Doxil) versus conventional doxorubicin for first-line treatment of metastatic breast cancer. Ann Oncol 15:440–9.
- Harrington KJ, Mohammadtaghi S, Uster PS, et al. (2001). Effective targeting of solid tumors in patients with locally advanced cancers by radiolabeled pegylated liposomes. Clin Cancer Res 7:243–54.
- Issels RD, Lindner LH, Verweij J, et al. (2010). Neo-adjuvant chemotherapy alone or with regional hyperthermia for localised high-risk soft-tissue sarcoma: a randomised phase 3 multicentre study. Lancet Oncol 11:561–70.
- Issels RD. (2008). Hyperthermia adds to chemotherapy. Eur J Cancer 44:2546–54.
- Issels R, Kampmann E, Kanaar R, Lindner LH. (2016). Hallmarks of hyperthermia in driving the future of clinical hyperthermia as targeted therapy: translation into clinical application. Int J Hyperthermia 32:89–95.
- Hauck ML, LaRue SM, Petros WP, et al. (2006). Phase I trial of doxorubicin-containing low temperature sensitive liposomes in spontaneous canine tumours. Clin Cancer Res 12:4004–10.
- Manzoor AA, Lindner LH, Landon CD, et al. (2012). Overcoming limitations in nanoparticle drug delivery: triggered, intravascular release to improve drug penetration into tumours. Cancer Res 72:5566–75.
- Kneidl B, Peller M, Winter G, et al. (2014). Thermosensitive liposomal drug delivery systems: state of the art review. Int J Nanomed 9:4387–98.
- Needham D, Anyarambhatla G, Kong G, Dewhirst MW. (2000). A new temperature-sensitive liposome for use with mild hyperthermia: characterisation and testing in a human tumour xenograft model. Cancer Res 60:1197–201.
- Limmer S, Hahn J, Schmidt R, et al. (2014). Gemcitabine treatment of rat soft tissue sarcoma with phosphatidyldiglycerol-based thermosensitive liposomes. Pharm Res 31:2276–86.
- Li L, Hagen TLM. Schipper D, et al. (2010). Triggered content release from optimised stealth thermosensitive liposomes using mild hyperthermia. J Control Release 143:274–9.
- Poon RT, Borys N. (2011). Lyso-thermosensitive liposomal doxorubicin: an adjuvant to increase the cure rate of radiofrequency ablation in liver cancer. Future Oncol 7:937–45.
- Wood BJ, Poon RT, Locklin JK, et al. (2012). Phase I study of heat-deployed liposomal doxorubicin during radiofrequency ablation for hepatic malignancies. J Vasc Interv Radiol 23:248.
- Zagar TM, Vujaskovic Z, Formenti S, et al. (2014).Two phase I dose-escalation/pharmacokinetics studies of low temperature liposomal doxorubicin (LTLD) and mild local hyperthermia in heavily pre-treated patients with local regionally recurrent breast cancer. Int J Hyperthermia 30:285–94.
- Lindner LH, Eichhorn ME, Eibl H, et al. (2004). Novel temperature-sensitive liposomes with prolonged circulation time. Clin Cancer Res 10:2168–78.
- Hossann M, Wiggenhorn M, Schwerdt A, et al. (2007). In vitro stability and content release properties of phosphatidylglyceroglycerol containing thermosensitive liposomes. Biochim Biophys Acta 1768:2491–9.
- Hossann M, Wang T, Wiggenhorn M, et al. (2010). Size of thermosensitive liposomes influences content release. J Control Release 147:436–43.
- Hossann M, Syunyaeva Z, Schmidt R, et al. (2012). Proteins and cholesterol lipid vesicles are mediators of drug release from thermosensitive liposomes. J Control Release 162:400–6.
- Willerding L, Limmer S, Hossann M, et al. (2016). Method of hyperthermia and tumour size influence effectiveness of doxorubicin release from thermosensitive liposomes in experimental tumours. J Control Release 222:47–55.
- Peller M, Willerding L, Limmer S, et al. (2016). Surrogate MRI markers for hyperthermia-induced release of doxorubicin from thermosensitive liposomes in tumours. J Control Release 237:138–46.
- VCOG. (2011). Veterinary cooperative oncology group – common terminology criteria for adverse events (VCOG-CTCAE) following chemotherapy or biological antineoplastic therapy in dogs and cats v1.1. Vet Comp Oncol. [Epub ahead of print]. doi: 10.1111/j.1476-5829.2011.00283.x.
- Bruggmoser G, Bauchowitz S, Canters R, et al. (2012). Guideline for the clinical application, documentation and analysis of clinical studies for regional deep hyperthermia. Strahlentherapie Und Onkologie 188:198–211.
- Gong W, Wang Z, Liu N, et al. (2011). Improving efficiency of adriamycin crossing blood brain barrier by combination of thermosensitive liposomes and hyperthermia. Biol. Pharm. Bull 34:1058–64.
- Zutphen LFM. (1995). Grundlagen der Versuchstierkunde Ein Beitrag zum tierschutzgerechten Umgang mit Versuchstieren und zur Qualität tierexperimenteller Ergebnisse; mit 38 Tabellen. Stuttgart, Jena, New York: G. Fischer, 341 p. ISBN: 9783437205323.
- Therasse P, Arbuck SG, Eisenhauer EA, et al. (2000). New guidelines to evaluate the response to treatment in solid tumours. European Organisation for Research and Treatment of Cancer, National Cancer Institute of the United States, National Cancer Institute of Canada. J Natl Cancer Inst 92:205–16.
- Schramm N, Englhart E, Schlemmer M, et al. (2013). Tumour response and clinical outcome in metastatic gastrointestinal stromal tumours under sunitinib therapy: comparison of RECIST, Choi and volumetric criteria. Eur J Radiol 82:951–8.
- Heußel CP, Meier S, Wittelsberger S, et al. (2007). Quantitative CT-Verlaufskontrolle von Lebermalignomen nach RECIST und WHO im Vergleich zur Volumetrie [Follow-Up CT Measurement of Liver Malignoma According to RECIST and WHO vs. Volumetry]. Fortschr Röntgenstr 179:958–64.
- Wahl RL, Jacene H, Kasamon Y, Lodge MA. (2009). From RECIST to PERCIST: evolving considerations for PET response criteria in solid tumours. J Nucl Med 50:1122–50.
- Szebeni J, Muggia F, Gabizon A, Barenholz Y. (2011). Activation of complement by therapeutic liposomes and other lipid excipient-based therapeutic products: prediction and prevention. Adv. Drug Deliv. Rev 63:1020–30.
- Haubner F, Ohmann E, Pohl F, et al. (2012). Wound healing after radiation therapy: review of the literature. Radiat Oncol 7:162.
- Allen TM. (2007). Pharmacokinetics and biopharmaceutics of lipid-based drug formulations. In: Gregoriadis G, ed. Interactions of liposomes with the biological milieu. New York; Liposome Technology, 49–64, ISBN: 9780849397257.
- Zamboni WC, Ramalingam S, Friedland DM, et al. (2009). Phase I and pharmacokinetic study of pegylated liposomal CKD-602 in patients with advanced malignancies. Clin Cancer Res 15:1466–72.
- Baur A, Stäbler A, Wendtner CM, et al. (2003). MR-imaging changes of musculoskeletal soft-tissue sarcomas associated with neoadjuvant chemotherapy and hyperthermia. Int J Hyperthermia 19:391–401.