Abstract
Purpose: Brain-derived neurotrophic factor (BDNF) is an important neurotrophin. The present study investigated the effects of head-out water immersion (HOI) on serum BDNF concentrations.
Methods: Eight healthy men performed 20 min head-out water immersion at 42 °C (hot-HOI) and 35 °C (neutral-HOI). These experimental trials were administered in a randomised order separated by at least 7 days. Venous blood samples were withdrawn at rest, immediately after the 20-min HOI, as well as at 15 and 30 min after the end of the HOI. Serum BDNF and S100β, plasma cortisol, platelet and monocyte counts, and core body temperature (Tcb) were measured.
Results: Tcb was higher at the end of the hot-HOI and 15 min after hot-HOI (p < 0.01), but recovered to pre-HOI level at 30 min after hot-HOI. No change in Tcb was recorded during neutral-HOI. BDNF level was higher (p < 0.05) at the end of the hot-HOI and at 15 min after the end of hot-HOI, and returned to the baseline at 30 min after hot-HOI. S100β, platelet count and monocyte count remained stable throughout the study. Cortisol level was lower at the end of the hot-HOI and returned to pre-HOI level during the recovery period. BDNF and S100β, cortisol, and platelet and monocyte counts did not change throughout the neutral-HOI study.
Conclusions: The present findings suggested that the increase in BDNF during 20-min hot-HOI was induced by hyperthermia through enhanced production, rather than by changes in permeability of the blood–brain barrier (BBB), platelet clotting mechanisms or secretion from monocytes.
Introduction
Brain-derived neurotrophic factor (BDNF) is a structurally related growth factor known to exert many of its effects on neurons primarily through the tyrosine protein kinase receptor (TrkB) [Citation1]. BDNF promotes and improves neuronal plasticity, suppresses neuronal cell death, induces neural regeneration and stimulates neuronal survival, particularly motor and sensory neurons of the peripheral and central nervous systems [Citation2–5]. Moreover, BDNF plays important roles in memory, learning, mood disorders, food intake and energy metabolism [Citation6–8]. Low blood levels of BDNF have been reported in patients with neurodegenerative diseases, including Alzheimer’s disease and major depression [Citation9,Citation10].
A well-known practical factor that increases blood level of BDNF is exercise [Citation11], which should be clinically relevant to the aforementioned disease processes. Exercise includes several physiological factors, such as muscle contraction, increase in ventilation and increase in core body temperature (Tcb) [Citation12]. Goekint et al. [Citation13] demonstrated that exercise at high room temperature increased Tcb and resulted in higher BDNF level than exercise at low room temperature. Based on these results, they concluded that blood BDNF was stimulated by the increase in the Tcb during exercise. However, there is no information on whether BDNF changes during increases in Tcb in humans independent of exercise.
Whole body heat stress, including hot water immersion, induces changes in cardiovascular function [Citation14] and immune function [Citation15,Citation16]. Perhaps it is for these reasons that whole body heat stress is used as treatment of cancer and chronic heart failure. On the other hand, whole body heat stress can have deleterious effects, such as induction of acute inflammatory response through heat stroke [Citation17]. In addition to these major effects, whole body heat stress affects the brain. The brain is reported to be the major source of circulating BDNF, though the platelets, monocytes, muscle, lung and other tissues also produce BDNF [Citation18–20]. Previous studies indicated that BDNF can cross the blood–brain barrier (BBB), and that increases in Tcb enhance the permeability of the BBB [Citation21–23]. The latter can result in a significant increase in serum S100β concentrations, suggesting that BDNF from the brain to blood can be assessed by measuring serum levels of S100β [Citation24]. Other factors such as circulating cortisol level can also alter BDNF levels [Citation25,Citation26], and exposure to heat stress is also known to increase plasma cortisol concentrations [Citation27].
The main hypothesis of the present study was that a rise in Tcb per se is an independent stimulator of blood BDNF level and that the main source of BDNF is within BBB. To test our hypothesis, we measured the concentrations of BDNF, S100β, and cortisol in peripheral blood, together with platelet and monocyte counts, before, during and after 20-min hot water immersion.
Methods
Participants
Eight healthy male volunteers (age 25.4 ± 3.3 years, height 172.8 ± 0.9 cm, weight 66.2 ± 3.9 kg and body mass index 22.2 ± 0.9 kg/m2) participated in this study. We interviewed the subjects to obtain medical history and current life style. All subjects had not participated in any regular exercise for more than one year. Subjects who were on medications for any medical or psychological condition and/or smokers were excluded from this study. The Research Ethics Committee of Wakayama Medical University approved the protocol of this study in advance. Informed written consent was obtained from all participants.
Experimental protocol
The subjects were instructed to refrain from strenuous physical exercise and alcohol drinking the day before the experiment and also to refrain from taking any fluids and food after 2200 the day before the experiment, with the exception of tap water, until the completion of the experiment. They reported to the laboratory between 900 and 1300 on the day of the experiment. Each subject wore a swimming trunk and rested in a thermoneutral room (28 °C) for at least 30 min, then prepared for ECG recording and blood pressure (BP) monitoring, as well as Tcb recording. Before the experiment, the subject voided, and then the 1-h control period began while sitting in a room outside the immersion tank. The immersion tank was 250 cm long, 100 cm wide and 120 cm deep. To determine the effects of head-out water immersion (HOI) in hot water at rest, each subject sat in the hot water (42.0 °C, hot-HOI) or thermo-neutral water (35.0 °C, neutral-HOI) HOI tank. The first test was completed under either hot-HOI or neutral-HOI conditions, administered in a randomised order, and then the second test was conducted under the other condition at least 7 days later. All subjects were allowed to drink water throughout the study. Tcb was measured throughout the experiment with a copper–constantan thermocouple inserted into the oesophagus at the level of the atrium. ECG was monitored throughout the experiment. Systolic and diastolic BP was measured by a sphygmomanometer three times at each measurement period. After confirmation of stable heart rate (HR) and BP, the above variables were measured in sitting resting position during 10-min rest, 20-min HOI and 30-min recovery (i.e. total experimental period = 60 min). Special care was taken to keep the position of the BP cuff at the water level during HOI in the sitting position. The mean arterial pressure (MAP) was calculated as one-third of pulse pressure plus diastolic pressure. BP was measured at 5, 25, 30, 40 and 55 min from the start of experiment. Venous blood samples (8 ml each) were collected through an intravascular catheter at before, at the end, 15 and 30 min after the end of HOI. The blood samples were used for measurements of serum BDNF and S100β (4 ml), plasma cortisol (2 ml) and blood cell counts (2 ml).
Analysis of blood samples
Total blood cell count was determined using a cell counter. Hematocrit (Hct) was measured by centrifugation. Other venous blood samples were drawn into pre-chilled serum venipuncture tubes and glass tubes containing ethylenediaminetetraacetic acid. The tubes were spun immediately at 3500 rpm for 10 min at 4 °C. The prepared sera and plasma were stored at −80 °C until analysis. Plasma cortisol levels were assayed using a competitive solid phase 125I radioimmunoassay technique (Dainabot Lab, Tokyo, Japan). BDNF was measured by enzyme-linked immunosorbent assay (ELISA) for BDNF (R&D Systems, Minneapolis, MN) with assay sensitivity <20 pg/ml and intra- and inter-assay coefficients of variability (average CV of different concentrations) of 5.0 and 9.0%, respectively. S100β was assayed using ELISA for S100β (BioVendor, Candler, NC) with assay sensitivity <5 pg/ml and intra- and inter-assay coefficients of variability (average CV of different concentrations) of 3.1 and 5.2%, respectively. BDNF and S100β immunoassays were performed in duplicate according to the recommendations of the manufacturers, by investigators blinded to the clinical data and origin of the blood sample.
Statistical analysis
All results were expressed as mean ± SD. Significant changes in each parameter relative to before HOI, at the end of HOI, 15 and 30 min after the end of HOI were examined by repeated measures ANOVA. Differences between hot-HOI and neutral-HOI were examined for statistical significance using repeated measures ANOVA. A value of p less than 0.05 was considered statistically significant.
Results
Changes in Tcb, MAP and HR
Tcb increased (p < 0.05) at the end of hot-HOI to 39.5 ± 0.6 °C and was still elevated at 15 min after the end of hot-HOI, but recovered to before HOI level at 30 min after the end of hot-HOI (. Tcb at neutral-HOI did not change during the entire study. Tcb at hot-HOI at the end of HOI and 15 min after the end of HOI were significantly (p < 0.05) higher than that at neutral-HOI (. The HR increased from 64.9 ± 8.2 before HOI to 112.0 ± 11.3 bpm at the end of hot-HOI (p < 0.05) but diminished to 78.8 ± 13.0 bpm at the end of the study (). The HR at neutral-HOI did not change throughout the study (). The HR at hot-HOI was significantly (p < 0.05) higher at the end of HOI than that of neutral-HOI (). MAP remained constant throughout the study of hot-HOI and neutral-HOI ().
Figure 1. Changes in core body temperature in head-out water immersion. Data are mean ± SD. *p < 0.05, compared with before immersion. #p < 0.05, hot-HOI vs. neutral-HOI. pre: before head-out water immersion, post: at the end of head-out water immersion, post15: at 15 min after the end of head-out water immersion, post30: at 30 min after the end of head-out water immersion. Core body temperature (Tcb) at hot-HOI was significantly higher (p < 0.05) at post and was still elevated at post15, but recovered to pre level at post30. Tcb did not change during the entire neutral-HOI arm of the study. Tcb at hot-HOI at post and post15 were significantly (p < 0.05) higher than those at neutral-HOI.
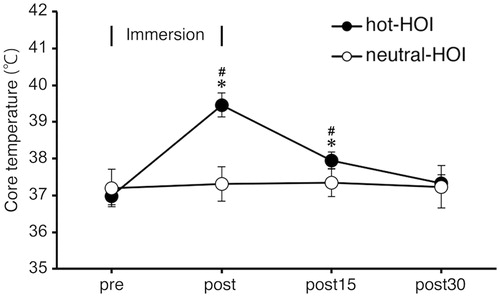
Table 1. Change in heart rate (HR) and mean arterial pressure (MAP) in head-out water immersion.
Changes in serum BDNF and S100β
Serum BDNF concentration was significantly higher (p < 0.05) at the end of hot-HOI and still high at 15 min after the end of hot-HOI, compared with the before hot-HOI. However, the level at 30 min after the end of hot-HOI was similar to that before hot-HOI level (. No change was observed in serum BDNF concentration throughout the study in the neutral-HOI. Serum BDNF of hot-HOI at the end of HOI, 15 and 30 min after the end of HOI were significantly (p < 0.05) higher than that of neutral-HOI (. On the other hand, serum S100β did not change throughout the hot-HOI and neutral-HOI studies, and was not significantly different between hot-HOI and neutral-HOI at the four different time points (.
Figure 2. Changes in serum BDNF in head-out water immersion. The centre of each bar represents the mean value for each time period and the length of the bar represents the SD value. *p < 0.05, compared with before immersion. #p < 0.05, hot-HOI vs. neutral-HOI. Each figure should show one open symbol (neutral HOI) and one solid symbol (hot-HOI). See for definitions. Serum BDNF concentration was significantly higher (p < 0.05) at post and was still high at post15, but returned to pre level at post30. No change was observed in serum BDNF concentration throughout the study in the neutral-HOI. Serum BDNF levels of hot-HOI at post, post15 and post30 were significantly (p < 0.05) higher than those of neutral-HOI.
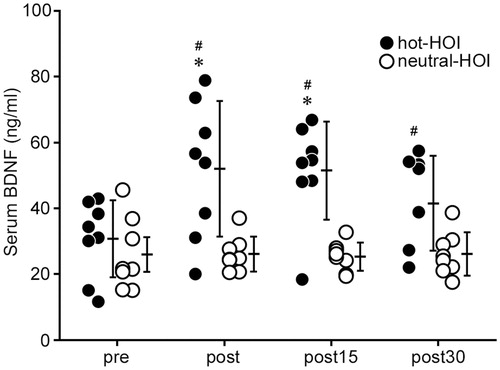
Changes in blood cell counts and plasma cortisol
Red and white blood cell counts, haemoglobin, and haematocrit, did not change throughout the study. The numbers of platelets, erythrocytes, leukocytes and monocytes remained stable throughout the study of hot-HOI and neutral-HOI, and there were no significant differences in each of these parameters among hot-HOI and neutral-HOI at the four different time points (). Plasma cortisol level decreased (p < 0.05) at the end of hot-HOI but returned to before HOI level at the two recovery time points, while it did not change throughout the neutral-HOI study (. However, plasma cortisol was not significantly different between hot-HOI and neutral-HOI at the four different time points (.
Figure 4. Changes in plasma cortisol in head-out water immersion. *p < 0.05, compared with before immersion. See and for definitions. In the hot-HOI experiment, plasma cortisol level decreased (p < 0.05) at post but returned to pre level at the two recovery time points, while it did not change throughout the neutral-HOI study. Plasma cortisol was not significantly different between hot-HOI and neutral-HOI at the four different time points.
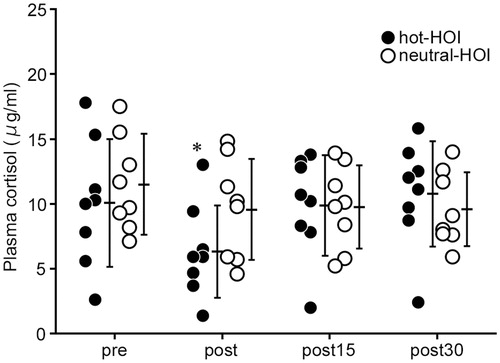
Table 2. Blood cell counts, platelet and monocytes during head-out water immersion.
Discussion
The major findings of the present study were as follows; (1) Body immersion in hot water (42 °C), but not lukewarm water (35 °C), for 20 min significantly increased serum BDNF, (2) the increase in serum BDNF was associated with a significant increase in Tcb resulting from immersion in hot water, (3) the rise in Tcb and serum BDNF levels were not associated with similar changes in serum S100β, (4) plasma cortisol level fell significantly at the end of the hot-HOI, and (5) platelet and monocyte counts remained stable throughout the study. The present findings suggest that hyperthermia resulting from immersion in hot water significantly and independently stimulates serum BDNF levels in humans and the brain is not the likely source of BDNF.
Exercise is known to induce a cascade of molecular and cellular processes that support brain plasticity and BDNF is assumed to play a crucial role in these induced mechanisms. Several animal studies have demonstrated that exercise increases various neurotrophic factors, including BDNF [Citation11]. Furthermore, serum BDNF is also known to increase under exercise conditions in humans [Citation28]. Some studies suggest that the magnitude of increase in BDNF might be exercise intensity-dependent [Citation28]. In this study, HR reached about 45% HRmax at end of HOI. However, Nofuji et al. [Citation29] reported that 30 min exercise at 60% VO2max increased serum BDNF, though the magnitude of increase was less than that recorded in this experiment. These results suggest that a rise in Tcb can increase serum BDNF with less HR increase that is needed for the same magnitude of increase serum BDNF during exercise.
The exercise-induced increase BDNF was reported recently to be related to a corresponding increase in Tcb [Citation13]. In that study, serum BDNF levels were higher during exercise conducted at 30 °C room temperature, compared to that in a room set at 18 °C (p = 0.02). The results suggested that the rise in Tcb augmented the increase in serum BDNF level during exercise. In fact, exercise increased Tcb, which in turn activated BDNF secretion. However, the present results of increases in BDNF recorded at physical rest conditions strongly suggest that a high Tcb acts independent of exercise to increase serum BDNF levels.
Rasmussen et al. [Citation28] measured brain BDNF level during exercise in humans and mice. In humans, blood samples were collected from the radial artery and the internal jugular vein during 4 h rowing exercise in 8 subjects, and a 2–3 fold release of BDNF from the brain compared with rest conditions was observed during exercise (p < 0.05). In mice, the brains were dissected and analysed for BDNF mRNA expression following treadmill exercise. The results showed that the BDNF mRNA expression levels in the hippocampus and cortex peaked at 2 h after exercise. These results suggest that the brain is the major but not the sole source of circulating BDNF. In comparison, the present result of the rise in BDNF during hot-HOI was observed under resting conditions, suggesting that the regulatory system responsible for the increase in Tcb induced the rise in BDNF, and that such system is different from that involved in exercise-induced rise in BDNF.
Previous animal studies suggested the presence of a BDNF transport system across the BBB, which allows BDNF to cross the BBB in both directions [Citation21,Citation22]. Other studies established the serum profile of S100β, a glial protein, as a highly sensitive marker of brain tissue damage in various neurologic diseases, such as traumatic brain injury and stroke [Citation30]. Increased transfer across the BBB is associated with a significant rise in serum S100β concentration, confirming S100β as a marker of BBB permeability. Watson et al. [Citation23] reported that a rise in Tcb during exercise was associated with increased serum S100β concentrations and increased BBB permeability. On the other hand, the circulatory level of S100β is known to increase after tissue damage, especially BBB and skeletal muscle. Moreover, S100β gene expression is detected in various peripheral tissues and organs, including adipose tissue and skeletal muscles [Citation31]. For example, the increase in serum S100β correlated strongly and creatine kinase in marathon runners at post race [Citation32]. Especially in healthy human, the source of increased serum S100β concentrations could be also increased production by damaged peripheral tissues and organs. In our study, hot-HOI was not associated with a similar increase in serum S100β level, suggesting that the hot-HOI-related increase in BDNF was neither associated with increased BBB permeability nor damage of peripheral tissue and organs.
Our study showed a significant fall in blood cortisol level during heat stress. This finding is in part consistent with the finding of Laatikainen et al. [Citation33], who also showed a decrease in plasma cortisol level in individuals exposed to heat stress. However, the fall reported in that study was small and statistically insignificant compared with the baseline (no heat exposure). The different extent of fall in blood cortisol level between the two studies could be due to methodological differences. For example, the above study was conducted in females while our study included male subjects only. Furthermore, the above study exposed the subjects to 120-min heat environment while our subjects were exposed to 20-min hot water immersion. Whether these methodological differences solely explain the different results need to be assessed in another study. Cortisol has a negative influence on the expression of BDNF in the brain [Citation34]. Increased release of glucocorticoids during exercise [Citation34] could restrain the initial exercise-induced increase in BDNF, for example, glucocorticoids suppress BDNF expression in the hippocampus [Citation35]. In the present study, plasma cortisol levels were lower when measured at the end of the 20-min hot-HOI and recovered to the baseline level at 15 min after the end of the hot-HOI. In comparison, BDNF was higher at the end of the 20-min hot-HOI and at 15 min after the end of the hot-HOI, compared with the baseline. These findings suggest that the decrease in plasma cortisol noted in our study probably contributed to the observed increase in BDNF.
The mechanisms involved in the observed increase in BDNF by hot-HOI were not investigated in the present study. However, the lack of statistical changes in haematocrit and erythrocyte count after hot-HOI rules out the role of dehydration in the increase in BDNF following HOI. BDNF is expressed in the majority of body tissues at relatively high levels [Citation36]. Fujimura et al. [Citation35] described the roles of various clotting processes in BDNF release from platelets. In addition, Kerschensteiner et al. [Citation20] reported that BDNF is also produced by activated human monocytes. The present results showed lack of changes in platelet and monocyte counts during hot-HOI. However, these findings do not exclude these structures in the production of BDNF.
Our study demonstrated increases in BDNF levels during hot-HOI. Although such increase was unlikely to be from platelets, BBB and monocytes, we cannot make any conclusions on the source of BDNF responsible for the observed rise during hot-HOI. Nevertheless, the study showed that experimentally-induced hyperthermia (hot-HOI) increased serum BDNF levels in normal subjects.
Conclusions
The present study demonstrated that 20-min hot-HOI resulted in a significant increase in serum BDNF level in young healthy men. The results suggest that elevation of Tcb is an independent factor responsible for the increase in serum BDNF level in humans.
Acknowledgements
We thank the medical staff at Nachikatsuura balneologic town hospital for their assistance, especially Prof. Toshikazu Kondo, Mr. Yuta Sakurai, Mr. Hiroshi Yamamoto, and Ms. Mami Yamashiro. We also thank Dr. Faiq G. Issa (Word-Medex Pty Ltd, Sydney Australia, www.word-medex.com.au) for the careful reading and editing of the manuscript.
Disclosure statement
The authors declare no conflict of interest. The authors alone are responsible for the content and writing of the paper.
Additional information
Funding
References
- Soppet D, Escandon E, Maragos J, et al. (1991). The neurotrophic factors brain-derived neurotrophic factor and neurotrophin-3 are ligands for the trkB tyrosine kinase receptor. Cell 65:895–903.
- Thoesen H. (1995). Neurotrophins and neuronal plasticity. Science 270:593–8.
- Bibel M, Barde YA. (2000). Neurotrophins: key regulators of cell fate and shape in the vertebrate nervous system. Genes Dev 14:2917–37.
- Poo MM. (2001). Neurotrophins as synaptic modulators. Nat Rev Neurosci 2:24–32.
- Carter AR, Chen C, Schwartz M, et al. (2002). Brain-derived neurotrophic factor modulates cerebellar plasticity and synaptic ultrastructure. J Neurosci 22:1316–27.
- Nakagawa T, Tsuchida A, Itakura Y, et al. (2000). Brain-derived neurotrophic factor regulates glucose metabolism by modulating energy balance in diabetic mice. Diabetes 49:436–44.
- Duman RS. (2002). Synaptic plasticity and mood disorders. Mol Psychiatry 7:S29–S34.
- Unger TJ, Calderon GA, Bradley LC, et al. (2007). Selective deletion of Bdnf in the ventromedial and dorsomedial hypothalamus of adult mice results in hyperphagic behavior and obesity. J Neurosci 27:14265–74.
- Karege F, Schwald M, Cisse M. (2002). Postnatal developmental profiles of brain-derived neurotrophic factor in rat brain and platelets. Neurosci Lett 328:261–4.
- Laske C, Stransky E, Leyhe T, et al. (2005). Stage-dependent BDNF serum concentrations in Alzheimer's disease. J Neural Transm (Vienna) 113:1217–24.
- Neeper SA, Gomes-Pinilla F, Choi J, et al. (1996). Physical activity increases mRNA for brain-derived neurotrophic factor and nerve growth factor in rat brain. Brain Res 726:49–56.
- Ethan RN. (1985). Physiological adaptations to aerobic training: the increase in blood volume induced by aerobic conditioning is a critical factor in improving performance and promoting resistance to fatigue. Am Sci 73:334–43.
- Goekint M, Roelands B, Heyman E, et al. (2011). Influence of citalopram and environmental temperature on exercise-induced changes in BDNF. Neurosci Lett 494:150–4.
- Fippel A, Von Sandersleben A, Bangert K, et al. (2007). Monitoring of whole-body hyperthermia with transesophageal echocardiography (TEE). Int J Hyperthermia 23:457–66.
- Dewhirst MW, Lee CT, Ashcraft KA. (2016). The future of biology in driving the field of hyperthermia. Int J Hyperthermia 32:4–13.
- Fisher DT, Chen Q, Skitzki JJ, et al. (2011). IL-6 trans-signaling licenses mouse and human tumor microvascular gateways for trafficking of cytotoxic T cells. J Clin Investig 121:3846–59.
- van den Tempel N, Horsman MR, Kanaar R. (2016). Improving efficacy of Hyperthermia in oncology by exploiting biological mechanisms. Int J Hyperthermia 32:446–54.
- Maisonpierre PC, Belluscio L, Squinto S, et al. (1990). Neurotrophin-3: a neurotrophic factor related to NGF and BDNF. Science 247:1446–51.
- Maisonpierre PC, Le Beau ME, Espinosa R, 3rd, et al. (1991). Human and rat brain- derived neurotrophic growth factor and neurotrophin-3: gene structure, distributions, and chromosomal localizations. Genomics 10:558–68.
- Kerschensteiner M, Gallmeier E, Behrens L, et al. (1999). Activated human T cells, B cells, and monocytes produce brain-derived neurotrophic factor in vitro and in inflammatory brain lesions; a neuroprotective role of inflammation? J Exp Med 189:865–70.
- Poduslo JF, Curran GL. (1996). Permeability at the blood–brain and blood–nerve barriers of the neurotrophic factors: NGF, CNTF, NT-3, BDNF. Brain Res Mol Brain Res 36:280–6.
- Pan W, Banks WA, Fasold MB, et al. (1998). Transport of brain-derived neurotrophic factor across the blood-brain barrier. Neuropharmacology 37:1553–61.
- Watson P, Shirreffs SM, Maughan RJ. (2005). Blood–brain barrier integrity may be threatened by exercise in a warm environment. Am J Physiol Regul Integr Comp Physiol 288:R1689–94.
- Kapural M, Krizanac-Bengez L, Barnettt G, et al. (2002). Serum S-100beta as a possible marker of blood-brain barrier disruption. Brain Res 940:102–4.
- Gould E, Cameron HA, Danieles DC, et al. (1992). Adrenal hormones suppress cell division in the adult rat dentate gyrus. J Neurosci 15:3642–50.
- Smith MA, Makino S, Kvetnansky R, et al. (1995). Stress and glucocorticoids affects the expression of brain-derived neurotrophic factor and neurotrophin-3 in the hippocampus. J Neurosci 15:1768–77.
- McMorris T, Harris RC, Swain J, et al. (2006). Effect of creatine supplementation and sleep deprivation, with mild exercise, on cognitive and psychomotor performance, mood state, and plasma concentrations of catecholamines and cortisol. Psychopharmacology 185:93–103.
- Rasmussen P, Brassard P, Adser H, et al. (2009). Evidence for a release of brain-derived neurotrophic factor from the brain during exercise. Exp Physiol 94:1062–9.
- Nofuji Y, Suwa M, Ichimiya A, et al. (2012). Different circulating brain-derived neurotrophic factor responses to acute exercise between physically active and sedentary subjects. J Sports Sci Med 11:83–8.
- Herrmann M, Jost S, Kutz S, et al. (2000). Temporal profile of release of neurobiochemical markers of brain damage after traumatic brain injury is associated with intracranial pathology as demonstrated in cranial computerized tomography. J Neurotrauma 17:113–22.
- Zimmer DB, Cornwall EH, Landar A, et al. (2005). The S100 protein family: history, function, and expression. Brain Res Bull 37:417–29.
- Hasselblatt M, Mooren FC, von Ahsen N, et al. (2004). Serum S100beta increases in marathon runners reflect extracranial release rather than glial damage. Neurology 62:1634–6.
- Laatikainen T, Salminen K, Kohvakka A, et al. (1988). Response of plasma endorphins, prolactin and catecholamines in women to intense heat in a sauna. Eur J Appl Physiol Occup Physiol 57:98–102.
- Schaaf MJ, de Jong J, de Kloet ER, et al. (1998). Downregulation of BDNF mRNA and protein in the rat hippocampus by corticosterone. Brain Res 813:112–20.
- Fujimura H, Altar CA, Chen R, et al. (2002). Brain-derived neurotrophic factor is stored in human platelets and released by agonist stimulation. Thromb Haemost 87:728–34.
- Brenner IK, Zamecnik J, Shek PN, et al. (1997). The impact of heat exposure and repeated exercise on circulating stress hormones. Eur J Appl Physiol Occup Physiol 76:445–54.