Abstract
Objectives: To report the feasibility and efficacy of percutaneous ablation of small hepatic malignant tumors that are invisible on ultrasound and inaccessible using in-plane CT guidance, using a combination of high-frequency jet-ventilation (HFJV) and electromagnetic (EM) needle tracking.
Methods: This study reviewed 27 percutaneous ablations of small hepatic tumors (<2 cm) performed using EM navigation-based probe placement and HFJV. All lesions were invisible on ultrasound and difficult to reach on CT requiring a double-oblique approach. The primary outcome was technical efficacy, defined as complete lesion coverage, and evaluated on contrast enhanced MRI after 3 and 6 months. Needle placement accuracy, the number of control CT acquisitions, procedure time, complications and radiation doses were assessed.
Results: Twenty-one patients with 27 treated lesions (14 hepatocellular carcinomas and 13 metastases) were included in this study. Mean tumor size was 12 ± 5.7 mm. Thirty-three percent of the lesions were located on the hepatic dome. Complete ablation was obtained in 100% at the 3- and 6-month MRI follow-up. The ablation probe was correctly placed on the first pass in 96%, with a mean path-to-tumor angle of 7 ± 4 degrees and a mean tip-to-tumor distance of 22 ± 19mm. A readjustment for additional overlapping application resulted in complete treatment in 4 patients. Needle placement took a mean 23 ± 12 min with mean radiation doses of 558 mGy*cm. No major complications were reported.
Conclusion: Percutaneous liver ablation of lesions that cannot be seen on US and requiring out-of-plane CT access can be successfully and safely treated using electromagnetic-based navigation and jet-ventilation.
Introduction
According to oncology and hepatology treatment guidelines (EASL and ESMO Clinical Practice Guidelines [Citation1,Citation2]), image-guided ablation using radiofrequency (RFA) or microwave (MWA) is a low-morbidity and liver-sparing treatment option in selected patients with liver tumors [Citation3,Citation4].
One important factor influencing the results of ablative therapy is tumor visibility, which allows accurate placement of ablation probes to obtain complete ablation with sufficient margins [Citation5]. The advantages of ultrasound (US) guided ablation include real-time monitoring of ablation probe placement in the tumor, accessibility and lack of exposure to radiation. For the above reasons, US is the preferred method for needle placement in many centers [Citation6]. However, some lesions are not visible on US, either due to a lack of contrast with the hepatic parenchyma, or because of the small size or depth of the lesion. Treatment can then be performed by computed tomography (CT) guidance with a needle trajectory on the axial plane. Out-of-plane trajectories are often necessary to avoid harming non-target structures (organs or vessels) complicating the procedure and resulting in higher radiation doses [Citation7] as well as a risk of inaccurate needle placement and incomplete treatment [Citation5].
To improve accuracy without increasing radiation exposure in CT-guided interventions, optical and electromagnetic CT stereotactic navigation systems have been developed [Citation8–10]. These systems track the ablation probe in real time with a set of images acquired at the beginning of the procedure allowing out-of-plane and complex targeting. The precision of probe placement can be further improved using high-frequency jet-ventilation (HFJV). Until recently, there was no other way to obtain immobility of targeted lesions located in the liver, lung, or kidney than apnea. The HFJV is a mechanical ventilation method for patients under general anesthesia, made of short and small pulses of air, inducing very small amplitude respiratory movements. Reduced respiratory movements of the liver provide therefore a better matching of the images acquired for navigation and the real position of the target in the liver during the procedure [Citation11]. Computed electromagnetic navigation (EMN) has recently been shown to be beneficial in a randomized study of various procedures compared to simple CT guidance [Citation9]. However, this approach has never been evaluated in the ablation of small liver tumors in difficult locations that are invisible on US.
The primary objective of our study was to evaluate the efficacy of the combination of computed electromagnetic navigation with HFJV for ablation of small US-invisible liver tumors in difficult locations (tumor complete ablation rate at 3–6 months). The secondary endpoint was the accuracy of needle placement, the number of control CT acquisitions, procedure time, overall safety and radiation doses.
Material and methods
Patient selection
This institutional review board-approved study (ID 2018–00660) analyzed cases gathered from our prospective anonymized database of percutaneous ablations between June 2017, date of the acquisition of the IMACTIS-CT® electromagnetic navigation system (Saint Martin D’hères, France) in our center, and January 2018.
Each case was evaluated by a multidisciplinary board and patients with primary or secondary liver tumors were selected for ablation according to oncological guidelines [Citation3,Citation4].
Inclusion criteria were small tumors (≤2 cm) treated by local ablation using EMN and HFJV that could not be seen on US and which were inaccessible by in-plane CT.
Ablation procedure description
Written informed consent was obtained in all patients. Operators were either senior interventional radiologists or fellows under supervision.
All procedures were performed under general anesthesia. Patients were placed in the supine position on the CT Table (128-multidetector CT, Phillips Ingenuity, Best, The Netherlands). After induction and orotracheal intubation, automatic HFJV was initiated at a ventilation frequency of 150 to 250 per minute and a driving pressure of 1–2 bar (Mansoon, Acutronic Medical System AG, Hirzel, Switzerland).
First, we performed 2D ultrasound planning with a 3.5-Mhz, curved, array probe, with and without harmonic imaging (IU22 Phillips Healthcare, Best, The Netherlands) to confirm that lesions could not be visualized. Triple-phase CT-scan (unenhanced, hepatic arterial and portal phases) was then performed following iodinated nonionic contrast injection in all patients (300 mg of iodine per ml at a flow rate of 3 mL/s). Once the target lesion was identified on CT, the series that provided the best tumor visualization was transferred to the navigation system. In lesions that were not visible in any series, a hepatic intra-arterial injection of iodized oil (Lipiodol, Guerbet, France) using a mobile C-arm fluoroscopy was performed to make them detectable. The CT series obtained immediately after were then transferred to the navigation system.
The IMACTIS-CT® navigation system was used with an electromagnetic locator composed of a magnetic receiver inside a needle holder, and a magnetic transmitter attached to the patient’s skin and detected on CT images with automatic recording of magnetic and CT coordinates (. The needle used by the operator was equipped with an electromagnetic receiver. The position and orientation of the needle holder and trajectory were displayed in real-time on two perpendicular 2D reconstructed CT images extracted from the 3D CT volume chosen by the operator. When 2D reconstructed images were used to plan the trajectory (to determine the optimal route before skin penetration), the radiologist performed the procedure with navigation assistance from the planned needle insertion point until the target was reached.
Figure 1. The IMACTIS-CT® navigation system is composed of a magnetic transmitter attached to the patient’s skin for patient referential (A), a needle holder with an electromagnetic locator (B) and a station with a touch screen (C). The needle used by the operator is equipped with an electromagnetic receiver that detects the position and the orientation of the needle guide and displays the anticipated trajectory of the needle in real-time on two perpendicular 2D reconstructed CT images extracted from the 3D CT volume.
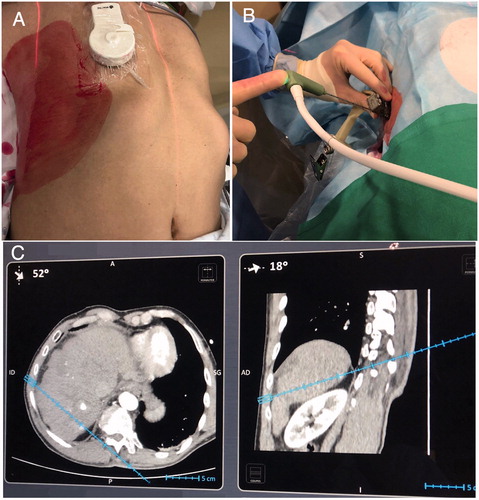
Our aim was to place the applicator approximately 1 cm from the tumor by EMN. A “first-pass” control CT was then acquired to confirm that the path corresponded to the planned trajectory and that tumor margins were adequate. If the position was considered to be correct, the applicator was advanced further (. Otherwise it was partially retrieved and readvanced as necessary. The final position of the applicator was confirmed by the final CT acquisition. When the needle required repositioning, CT acquisitions covering the needle path were repeated at the operator’s discretion.
Figure 2. Dynamic T1-weighted MR sequences with contrast administration in arterial (left) and venous (right) phases showed a small (1 cm) segment VIII lesion with typical pattern of HCC, in a cirrhotic patient of 73 year old A. The lesion was not visualized on US and unenhanced CT (left). An intra-arterial hepatic injection of iodized oil (Lipiodol) was performed to make the lesion detectable (right) B. A one-plane trajectory access was impossible on CT-guidance. Therefore, a RF electrode was inserted using EMN and HFJV. A first-pass control CT (coronal reconstructions) was performed before the target had been reached to confirm both the correct path of the applicator and the distance we needed to advance C. The probe was then advanced accordingly on EMN until the target was reached, as confirmed on coronal pre-ablation CT acquisition D. A single ablation was performed and cauterization of the electrode track had been used. Axial post-ablation CT confirmed an ablation area that covered the entire lesions E. Three-month (left) and 6-month (right) contrast enhanced MRI confirmed a complete ablation without residual enhancement F. HCC: hepatocellular carcinoma; CT: Computed Tomography; EMN: electromagnetic navigation; HFJV: high-frequency jet-ventilation; RF: radiofrequency.
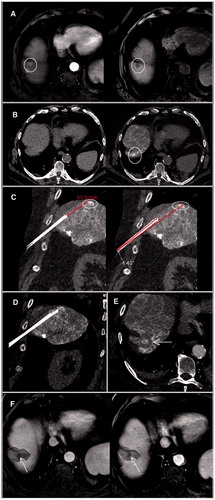
The ablation was then performed using a radiofrequency probe (Cool-tip Cluster Electrode, Covidien, Dublin, Ireland) or microwave system (Certus 140 with PR 15 antennas, NewWave, Medical Madison, Medical Madison, WI), depending on the size of the lesion and the operator’s preference. Microwaves were preferred for lesions located close to blood vessels to reduce potential heat-sink effects. Manufacturer’s standard ablation protocols were used.
An immediate follow-up triple-phase CT-scan was performed to evaluate treatment success. The patient was then transferred to the recovery unit and was normally discharged from hospital the following day.
A follow-up contrast enhanced MRI was performed 3 and 6 months after the intervention including diffusion-weighted sequences, T2-weighted spin echo, as well as T1-weighted sequences with and without contrast administration.
Assessment of endpoints
Patient characteristics (age, sex, the presence of cirrhosis, prior local and general treatments) were recorded prospectively in our database. Tumor characteristics such as maximum diameter, location depth (distance of the lesion to the needle skin entry point), distance to the capsule, affected liver segment and lesion visibility on unenhanced and/or enhanced CT were registered. Hepatic location such as dome location less than 1 cm away from the diaphragm, subcapsular location within 10 mm of the liver capsule and lesions abutting a prior ablation zone (<1 cm) were also noted in the database.
Primary technical efficacy was the assessment of complete ablation, evaluated at 3 and 6 months on contrast enhanced MRI and defined as an ablation zone covering the entire tumor without residual disease or local recurrence [Citation12].
Secondary outcome was the accuracy of applicator placement, mainly assessed by the initial path evaluated on first-pass CT, at a time when the applicator was not yet supposed to reach the tumor (); the angle between the needle trajectory and the line connecting the entry point on the skin and the center of the lesion, the distance between the distal mechanical tip of the applicator and the center of target, and the need for a repositioning of the needle because of an incorrect initial path, were recorded.
Figure 3. First-pass control CT (coronal reconstruction) acquired after applicator insertion using EMN system and HFJV, for a 4-mm liver metastasis located on the dome, in a 73-year-old man with colon cancer. The needle path was considered adequate to reach the target, with an angle between the needle trajectory and the line connecting the entry point and the center of the lesion of 5.7 degrees; the tip-to-tumor distance was 29 mm A. The probe was then advanced accordingly on EMN. A second control CT showed that the target had been reached B. EMN: electromagnetic navigation; HFJV: high-frequency jet-ventilation; CT: Computed Tomography.
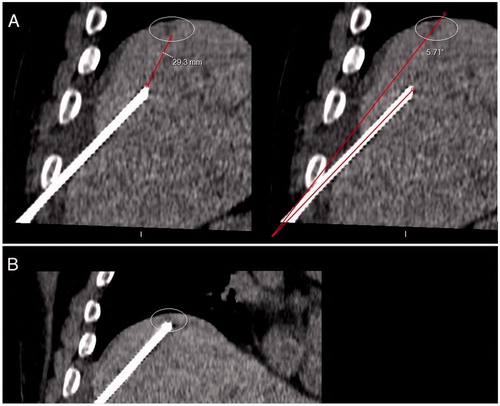
Accuracy was also assessed by the need for a second overlapping ablation.
The number of control CT acquisitions during needle placement was noted.
Needle placement time was calculated from planning to the last pre-ablation CT acquisition. The entire procedure time was also recorded.
Total radiation dose (total dose-length product [DLPtotal]) was extracted from scanner-generated patient protocols. The interventional dose (interventional dose-length product [DLPinter]) corresponding to the needle placement was calculated from all intermediate Control-CTs protocols.
Safety outcomes were procedure-related adverse events according to the CIRSE scale [Citation13].
Results
Patients
Percutaneous ablation was performed in 21 patients (27 lesions), including 15 men and 6 women (mean age, 67 years old) with 14 hepatocellular carcinomas and 13 metastases. None of the patients had ascites. Patient and lesion characteristics are summarized in .
Table 1. Patients and lesions characteristics.
Lesion characteristics
Mean tumor size was 12 ± 5.7 mm. Skin to lesion distance was 111 ± 31mm and the mean distance to the capsule was 11.9 ± 17.1 mm. Nine lesions (33%) were located on the dome. Eighteen lesions (67%) were subcapsular, and six (22%) were located abutting a prior ablation zone.
Only 6 lesions (22%) were detectable on unenhanced CT. Fourteen (52%) were only visible following iodinated contrast administration. An intra-arterial injection of iodized oil was necessary in the 7 lesions that could not be seen on enhanced CT, all of which were HCC.
Procedure
Radiofrequency ablation was performed in 25 lesions, using a Cool-tip cluster radiofrequency ablation electrode (E Series, Covidien, Dublin, Ireland). Two ablations were performed by microwave antenna with PR 15 antennas (Certus 140, NewWave, Medical Madison, WI). This included one lesion abutting the inferior vena cava requiring ablation combined with inferior vena cava balloon occlusion to minimize the heat-sink effect. Mean ablation time per lesion was 10 ± 5 min and 10 ± 6 min for RFA and MWA, respectively. The mean number of applications per lesion was 1.14 ± 0.35.
Outcomes
The applicator was properly inserted on the first pass in 26 out of 27 cases (96%), and the needle reached the target or its margins without withdrawing the probe for repositioning (). Mean path-tumor angle was 7 ± 4 degrees, with a mean tip-to-tumor distance of 22 ± 19 mm.
Table 2. Characteristics and results of liver ablations performed for 21 patients with a total of 27 liver tumors.
Incorrect applicator placement was observed in one patient. The angle path-tumor was 15°. The probe was retrieved and repositioned by EMN.
Ablation margins on immediate post-ablation CT were considered sufficient after one application in 23 lesions (85%). Secondary applicator readjustment was performed by EMN because of uncertain margins in 4 patients and complete treatment was obtained.
Probe placement took a mean 23 ± 12 min, with a mean 3.2 (range 1–7) short control CT acquisitions and a mean interventional radiation dose of 558 ± 526 mGy*cm.
Complete ablation was observed in 100% of cases at the 3- and 6-month MRI follow-up. Six patients (22%) presented with new hepatic lesions at 3 months and 15 (55%) at the 6-month MRI.
Complications
Procedure-related complications occurred in 2 patients, both were local infections (post ablation abscess) that developed 4 and 30 days after ablation. One of the two cases was a diabetic patient that was treated with RFA for 5 liver metastasis of a colon tumor. Four lesions were treated by US-guidance (located in segments II, VIII, V, and subcapsular in segment VII) and one subcapsular lesion of segment VI by EMN-CT-guidance. This patient also developed nosocomial pneumonia and pleural effusion. The second complication was a local post-ablation abscess that developed 30 days after ablation of a segment IV HCC. Both complications were successfully treated with antibiotics and required no invasive treatment. None of the patients presented with any long-term disabilities.
No complications were reported related to the use of HFJV or EMN.
Discussion
In a randomized study comparing EMN and conventional CT guidance, EMN was shown to be more accurate (distance error) and to require fewer CT acquisitions [Citation9].
The present study evaluated a more difficult clinical problem. Instead of simple procedures under local anesthesia, we studied complex ablations of small lesions that were not visible on US and inaccessible with in-plane CT guidance.
We usually use US guidance for needle placement for percutaneous liver ablation in our center. However, in certain cases conventional US guidance is insufficient, for example in isoechoic lesions, when there is no discrimination with surrounding cirrhotic nodules in cirrhosis, in lesions deep in the liver or on the hepatic dome, or in tumors abutting a prior ablation [Citation14]. Kim JE et al. showed that US guidance was unfeasible in approximately one third of HCC ablations [Citation15]. The main reason was tumor invisibility (74%), usually due to small tumor size (RR = 0.823, p < .001) [Citation15]. In the current study, treated lesions were small with a mean diameter of 12 ± 5.7 mm. Most of the treated lesions were also deep in the liver (mean distance to the skin was 111 ± 31 mm) and 33% were located on the dome of the liver below the diaphragm. In many centers, these lesions, which are difficult to access on conventional CT-guidance because of out-plane trajectories, may be unsuitable for percutaneous ablation, and if ablation is performed, may have a high risk of incomplete margins and failure [Citation16]. Lesions that cannot be seen with conventional US may also be visualized by contrast-enhanced ultrasound (CEUS) [Citation17]. However, ablation may still be difficult due to the short duration of the different vascular phases with CEUS, requiring rapid needle insertion. Moreover, small lesions may not be visible on CEUS.
Real-time MR-guided ablation [Citation18] or fusion imaging guidance using real-time US associated with CT or MR images may also be used in tumors that are not clearly seen with conventional US [Citation19]. However, these techniques are not widely available.
All of the cases in our study were successfully treated under EMN and HFJV, with 100% complete ablation at 3 and 6 months. These success rates are similar to those obtained following unselected tumor ablation. Rhim et al. treated 674 HCC with RFA with a success rate of 96.7% [Citation20]. Most of the ablation procedures in that study (87%) were performed under US guidance and results were assessed by four-phase dynamic contrast-enhanced CT immediately, and at 1- and 3-month intervals.
The accuracy of needle tracking using EMN and HFJV was well demonstrated in our study and was within the range reported elsewhere for similar navigation systems [Citation21,Citation22]. Almost all needle pathways were right on the first pass with a mean needle-to-tumor center angle of 7 degrees, for a needle that was already close to the target (mean distance 22 mm), as measured at a time when the applicator was not yet supposed to reach the tumor.
Angular displacement errors reported in previous reports are similar (2.7 [16] and 4.7 [9] degrees), even if the angles were measured when the needle had reached the lesion.
We needed to remove and reinsert the applicator in only one case (4%), which was similar to the results of Engstrand et al. [Citation16]. In that case, although the trajectory was close to planned trajectory (angle to the tumor of 15°), the needle progressed in a parallel extra-hepatic pathway, pushing the liver instead of penetrating it. That does also happen using classic CT guidance, especially when the entry point in the liver is tangent to the capsule.
Despite a good initial path, a readjustment of the applicator for additional overlapping ablation was performed in 4 patients. In these cases, the applicator reached the target margins without being removed or drawn back. However, the ablation did not cover the whole expected margins (1 cm all over the lesion), particularly on the opposite margin of the lesion. The high accuracy of needle placement in our study suggests that HFJV could be effective in reducing respiratory-induced liver motion, resulting in more reliable, thus safer, navigation [Citation23].
The use of a navigation system allows the operators to reach the target with few control CT acquisitions (mean 3.2 ± 1.3), keeping the radiation dose as low as possible for both patients and radiologists.
Our total procedure time was similar to that of US guided-ablations. In our study the mean total procedure time was 51 min (range: 23–106) and depended on the number of lesions treated. Seven of the 9 procedures (78%) that lasted more than an hour involved multiple lesions treated in the same session.
The EMN system also has the advantage of treating lesions that cannot be visualized on US and unenhanced CT, which represented 78% of the lesions in our study. With conventional CT guidance, needle insertion is based on nearby anatomic landmarks. With EMN navigation, contrast-enhanced CT acquisitions are memorized in the system, allowing continuous target visualization while advancing the needle. This also provides to clearly distinguish some lesions located at the contact zone of a former ablation zone or scar tissue. The navigation system provides real-time multiplanar interactive imaging with clear visualization of the tumor and the surrounding anatomy and all the features necessary for targeting [Citation24].
No complications directly related to the use of the navigation system or HFJV were reported in the present study. Both adverse events were common ablation-related complications.
This study has several limitations including the relatively small number of patients treated and its retrospective design, which could create a selection bias. Also, there was no control group with ablation performed by conventional CT guidance only. Finally, the follow-up period was relatively short and the study was focused on local recurrence only.
It is important to note that the present study was based on the first ablation cases using EMN-CT guidance. Thus, results could be expected to improve over time as the learning curve increases, especially the procedure time and operator confidence in the navigation system. However, all operators found the system to be easy to manage and simple to use from the outset.
In conclusion, navigation systems are an important development in interventional radiology that can improve the accuracy of complex targeting. These systems can make difficult cases requiring a double-oblique trajectory technically feasible, for example out-of-plane access to deep tumors located near the diaphragm or US-undetectable lesions, as well as increase the success of challenging percutaneous ablations.
Disclosure statement
No potential conflict of interest was reported by the authors.
References
- Galle PR, Forner A, Llovet JM, et al. EASL clinical practice guidelines: management of hepatocellular carcinoma. J Hepatol. 2018;S0168-8278(18):30215–30210.
- Van Cutsem E, Cervantes A, Adam R, et al. ESMO consensus guidelines for the management of patients with metastatic colorectal cancer. Ann Oncol. 2016;27(8):1386–1422.
- Lencioni R, Crocetti L. Local-regional treatment of hepatocellular carcinoma. Radiology. 2012;262(1):43–58.
- Bruix J, Gores GJ, Mazzaferro V. Hepatocellular carcinoma: clinical frontiers and perspectives. Gut. 2014;63(5):844–855.
- Bale R, Widmann G, Stoffner DI. Stereotaxy: breaking the limits of current radiofrequency ablation techniques. Eur J Radiol. 2010;75(1):32–36.
- Liu F, Yu X, Liang P, et al. Contrast-enhanced ultrasound-guided microwave ablation for hepatocellular carcinoma inconspicuous on conventional ultrasound. Int J Hyperthermia. 2011;27(6):555–562.
- Beyer LP, Pregler B, Nießen C, et al. Stereotactically-navigated percutaneous Irreversible Electroporation (IRE) compared to conventional IRE: a prospective trial. Peer J. 2016;4:e2277.
- Arnolli MM, Hanumara NC, Franken M, et al. An overview of systems for CT- and MRI-guided percutaneous needle placement in the thorax and abdomen. Int J Med Robot. 2015;11(4):458–475.
- Durand P, Moreau-Gaudry A, Silvent AS, et al. Computer assisted electromagnetic navigation improves accuracy in computed tomography guided interventions: A prospective randomized clinical trial. PLoS One. 2017;12(3):e0173751.
- Appelbaum L, Mahgerefteh SY, Sosna J, et al. Image-guided fusion and navigation: applications in tumor ablation. Tech Vasc Interv Radiol. 2013;16(4):287–295.
- Denys A, Lachenal Y, Duran R, et al. Use of high-frequency jet ventilation for percutaneous tumor ablation. Cardiovasc Inter Rad. 2014;37(1):140–146.
- Crocetti L, De Baere T, Lencioni R. Quality improvement guidelines for radiofrequency ablation of liver tumours. Cardiovasc Inter Rad. 2010;33(1):11–17.
- Filippiadis DK, Binkert C, Pellerin O, et al. Cirse quality assurance document and standards for classification of complications: the cirse classification system. Cardiovasc Intervent Radiol. 2017;40(8):1141–1146.
- Rhim H, Lee MH, Kim YS, et al. Planning sonography to assess the feasibility of percutaneous radiofrequency ablation of hepatocellular carcinomas. AJR Am J Roentgenol. 2008;190(5):1324–1330.
- Kim JE, Kim YS, Rhim H, et al. Outcomes of patients with hepatocellular carcinoma referred for percutaneous radiofrequency ablation at a tertiary center: analysis focused on the feasibility with the use of ultrasonography guidance. Eur J Radiol. 2011;79(2):e80–e84.
- Engstrand J, Toporek G, Harbut P, et al. Stereotactic CT-Guided percutaneous microwave ablation of liver tumors with the use of High-Frequency jet ventilation: an accuracy and procedural safety study. AJR Am J Roentgenol. 2017;208(1):193–200.
- Ahn SJ, Lee JM, Lee DH, et al. Real-time US-CT/MR fusion imaging for percutaneous radiofrequency ablation of hepatocellular carcinoma. J Hepatol. 2017;66(2):347–354.
- Clasen S, Pereira PL. Magnetic resonance guidance for radiofrequency ablation of liver tumors. J Magn Reson Imaging. 2008;27(2):421–433.
- Song KD, Lee MW, Rhim H, et al. Fusion imaging-guided radiofrequency ablation for hepatocellular carcinomas not visible on conventional ultrasound. AJR Am J Roentgenol. 2013;201(5):1141–1147.
- Rhim H, Lim HK, Kim YS, et al. Radiofrequency ablation of hepatic tumors: lessons learned from 3000 procedures. J Gastroenterol Hepatol. 2008;23(10):1492–1500.
- Mbalisike EC, Vogl TJ, Zangos S, et al. Image-guided microwave thermoablation of hepatic tumours using novel robotic guidance: an early experience. Eur Radiol. 2015;25(2):454–462.
- Krücker J, Xu S, Venkatesan A, et al. Clinical utility of real-time fusion guidance for biopsy and ablation. J Vasc Interv Radiol. 2011;22(4):515–524.
- Widmann G, Schullian P, Haidu M, et al. Targeting accuracy of CT-guided stereotaxy for radiofrequency ablation of liver tumours. Minim Invasive Ther Allied Technol. 2011;20(4):218–225.
- Ahmed M, Solbiati L, Brace CL, et al. Image-guided tumor ablation: standardization of terminology and reporting criteria-a 10-year update. Radiology. 2014;273(1):241–260.