Abstract
Background and objective
Warm exposure places high demands on thermoregulation mechanisms, which depend on the effectiveness of the microvascular function. The associations between the microcirculation and metabolism in warm environments have received little attention. The purpose of this study was to explore skin blood flow (SkBF) in response to food intake in a warm environment compared to control.
Methods
Thirty-two healthy, acclimated-to-warm-environment and physically active participants were recruited (20 females and 12 males). They participated in two sessions (warm environment: 31 °C and control: 20 °C, presented in randomized order). SkBF was measured before and after standardized food intake through the acquisition of perfusion signals by laser Doppler flowmetry (Periflux System 5000), following a local heating protocol.
Results
SkBF was affected by the environmental temperature, showing an increase in the warm environment compared to control (all p < .001). SkBF was significantly affected by food intake (all p < .007), being reduced after meals. In the men’s group, SkBF was reduced in both environmental temperatures after meals. In women, meals affected SkBF at 20 °C but not in the warm environment.
Conclusion
These results may indicate a competition between thermo- and glyco-regulation in a warm environment to the detriment of glucose homeostasis in women.
Introduction
Exposure to heat places a high demand on thermoregulatory mechanisms, whose effectiveness is based on microvascular function [Citation1]. Due to the vasomotor capacity of the vessels, blood is redistributed according to thermal changes. Increasing and decreasing blood flow in response to changes in both core and skin temperatures contributes to the matching of heat elimination with heat production. The adaptation of the skin blood flow plays a major role in human thermoregulatory control [Citation2]. Therefore, an alteration in this control has important clinical implications and can substantially impair the ability to maintain normal body temperatures.
Microvascular dysfunction can contribute to metabolic disorders, although it is more likely to be consecutive to them. This is the case in diabetes − particularly long-term or untreated diabetes corresponding to poor glycemic control − which leads to declines in vascular health, with occurrences of both peripheral microvascular and macrovascular dysfunction. Impaired vasodilation and the subsequent insufficient skin blood flow and sweating responses explain in part the reduced capacity to dissipate heat [Citation3].
Most of the observations have concerned men. Nevertheless, some authors have identified thermal response patterns specific to women along with differences in exogenous heat load, heat loss and endogenous heat load during exercise, which have been related to their body surface to body mass ratio, subcutaneous fat content, and lower exercise capacity [Citation4].
In healthy people, it is well known that carbohydrate metabolism is altered with warm exposure [Citation5–7]. However, both (1) the effects of heat exposure on metabolism and the subsequent impact on microvascular function and (2) the reverse situation of the effects of heat-related microvascular alterations on metabolic dysfunction have been poorly investigated. Little research has associated these two situations, although millions of people are concerned.
The purpose of this study was twofold: to investigate the effect of warm exposure on the skin blood flow as a reflection of microvascular function in young healthy men and women in fasting condition, and to compare the skin blood flow in these same participants in response to food intake (i.e., pre- versus postprandial) in warm and control conditions.
Materials and methods
Study design
After inclusion, each participant was examined on two separate occasions (2 − 7 days apart) in either a control environment (wet-bulb globe temperature (WBGT): (20 ± 1 °C) or a warm environment (31 ± 2 °C). The environmental temperatures were continuously measured during the trials with a HD32.2 device (Delta Ohm, Padova, Italy) and the order of the exposures was randomized.
Sessions started between 6.30 and 8.00 AM after a minimum 12 h overnight fast. The participants were instructed to abstain from high-intensity exercise and alcohol intake the day before each session. The clinical measurements were performed within 15 min of arrival at the lab unless additional time was required to obtain a resting state. Then, a skin blood flow (SkBF) measurement protocol, including baseline values and the response to local heating, was applied before and after a standardized meal proportional to each participant’s mass.
Participants
Thirty-two (20 females and 12 males) healthy, acclimated-to-tropical-climate and physically active young adults were recruited. To participate in this study, they had to have lived in the West Indies for at least 6 months. Inclusion criteria were the following: age ≥18 and ≤30 years, body mass index (BMI) ≥18·0 and ≤25·0 kg.m−2, acclimated to tropical climate, nonsmoking, normotensive, and practicing moderate or intensive physical activity three times a week. Exclusion criteria were the following: chronic or acute disease, medication use, and history of heat exhaustion or food allergy. All procedures used in this study were in accordance with institutional guidelines and the Helsinki Declaration of 2013. The study procedures were approved by the Human Subject Review Committee (CPP 17.10.10). The protocol (TDEX) was registered in EudraCT (2017-A02226-47).
Clinical measurements
Data on body composition were collected by bioelectrical impedance analysis using an InBody 720 analyzer with InBody 3.0 software (BioSpace, Seoul, South Korea) to determine body fat mass. BMI was calculated from weight and height data. Tympanic temperature was measured with a digital ear thermometer (Omron Gentle Temp 520) in all experimental sessions in pre-and postprandial states. Systolic and diastolic blood pressures were measured with a tensiometer (Omron M6, Healthcare Co., Ltd., Kyoto, Japan) before each skin blood flow measurement in the two experimental sessions. Glycemia was monitored twice (pre- and postprandial) during the experimental sessions with an Accu-Chek Performa glucometer (Roche Diagnostics, Mannheim, Germany).
Skin blood flow
SkBF and SkBF response to local hyperthermia were measured by laser Doppler flowmetry using the Periflux System 5000 (Perimed, Järfälla, Sweden) and its associated software Perisoft (Perimed), which enables continuous SkBF recording (mV). Laser Doppler flowmetry provides continuous real-time measurement of local microcirculatory blood flow. A temperature-controlled skin probe was attached to the distal pad of the middle finger of the left hand with adhesive tape.
Local hyperthermia protocol
After 5 min of recording (baseline phase, recording the baseline level), skin temperature was raised to 42 °C at a rate of 0.1 °C/s [Citation8] for 20 min. This local hyperthermia produces profound vasodilation, also known as cutaneous thermal reactive hyperemia. Local hyperthermia results in a biphasic increase in blood flow: (1) a rapid increase with a peak (peak phase, after local hyperthermia) reached within the first 5 min of hyperthermia, related to axon reflex, and followed by a brief nadir, and (2) a prolonged plateau (plateau phase, after local hyperthermia), mainly induced by nitric oxide (NO) [Citation9].
Meal protocol
The participants’ diet was controlled during the two experimental sessions. After the first measure of SkBF, they were invited to eat. They were presented with a tray of ham and cheese sandwiches of known energy composition, along with a glass of tropical juice (Banga®), with the quantity equal to 4 mL/kg body weight. The meal lasted 20 min. They were instructed to eat everything.
Statistical analyses
Repeated measures analyses of variance were performed for the relevant variables as follows: with three factors: environmental temperature with two levels (warm and control), prandial state with two levels (pre- and post-), and measurement phases with three levels (baseline, peak, and plateau), or with two factors: environmental temperature with two levels (warm and control) in prandial state with two levels (pre- and post).
As the environmental temperature x meal x sex interaction effects on the main variables were significant, the men’s and women’s data were separated for independent statistical analyses in order to enhance understandability.
Data were tested for sphericity using Mauchly’s test and if the assumption of sphericity was violated, the Greenhouse–Geisser correction was used to adjust the degrees of freedom. Post-hoc Scheffé’s tests were performed in cases of a significant interaction effect. All results were analyzed with the SPSS 23 software package (SPSS Inc, Chicago, IL, USA). Statistical significance was set at p < .05. All data are reported as mean ± SD unless otherwise stated.
Results
presents the participants’ anthropometric characteristics. Data are presented separately based on sex.
Table 1. Participants’ anthropometric characteristics.
Glycemia
Glycemia () was affected by the environmental temperature (p < .001 in women and p < .001 in men), with higher values observed in warm temperature than in control temperature. There was a significant simple effect of meal (all p < .012 in women and men). The temperature x meal interaction was significant in both women and men (all p < .015), with a larger pre − postprandial increase at 31 °C than at 20 °C.
Table 2. Glycemia and mean arterial blood pressure before and after the meal in the two environmental conditions.
Blood pressure
Mean blood pressure was not affected by environmental temperature, the meal or their interaction (all p > .11 in women and men; ).
Skin blood flow
Regarding the comparison between the warm and control environments before the meal, SkBF was affected in all phases by the environmental temperature (single effect of environmental temperature: p < 0.001) in the women () and men (), with higher values at 31 °C than at 20 °C. SkBF was also measured in response to the local heating protocol: we observed a simple effect of phase (baseline, peak and plateau) characterizing the reactivity to local heat stress in both women (p < .001) and men (p < .001). The phase x environmental temperature interaction was significant in women (p = .002) and men (p = .001).
Figure 1. Skin blood flow (PU) and its determinants in response to local heating in women before (solid line) and after (dashed line) the meal at 20 °C (gray line) and at 31 °C (black line). (A) Skin blood flow (PU) phases (p < .001); temperature (p < .001); temperature x phase (p = .002); meal x temperature (p = .01); all other p > .13. (B) Velocity (VU) phases (p < .001); temperature (p < .001); meal x temperature (p < .001); all other p > .29. (C) Concentration of moving blood cell (CU) phases (p < .001); temperature x phase (p = .02); all other p > .20. Post-hoc velocity results are identified as follows: *Significant difference as compared with baseline phase in the same meal state and environmental condition. #Significant difference as compared with the pre-prandial state in the same environmental condition. +Significant difference as compared with the control temperature.
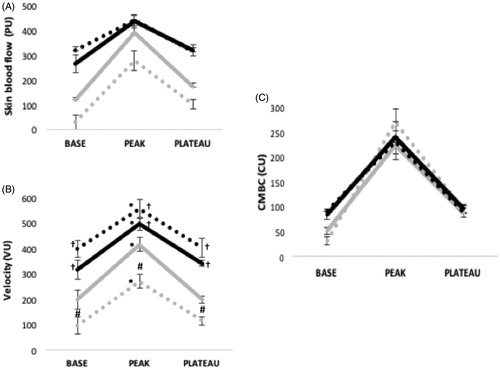
Figure 2. Skin blood flow (PU) and its determinants in response to local heating in men before and after food intake (A) Skin blood flow phases (p < .001); temperature (p < .001); meal (p = .007); temperature x phases (p < .001); all other p > .40. (B) Velocity (VU) phases (p < .001); temperature (p <.001); meal (p < .001); temperature x phase (p < .001); meal x temperature (p = .001); meal x phase (p < .001); temperature x phase x meal (p = .60). (C) Concentration of moving blood cell (CU) phases (p <.001); temperature (p = .006); temperature x phase x meal (p < .001), all other p > .16.
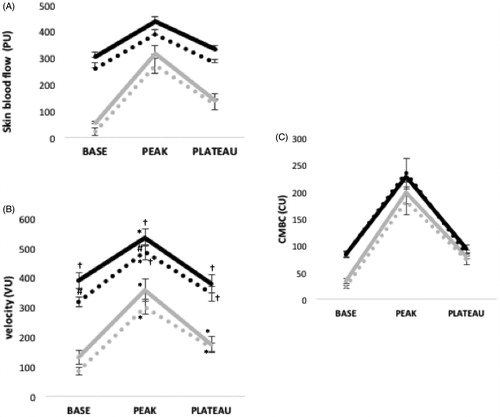
The meal effect was significant in men (p = .007). In the women’s group (p = .017), there was an interaction effect for meal x environmental temperature (p < .001).
The analysis of variance for the men’s and women’s SkBF variations in response to the local heating protocol pre- and postprandial and in the two environmental conditions revealed no other significant interaction, such as meal x environmental temperature x phase (p > .14) or other interactions where the meal was implied.
Determinants of skin blood flow
The velocity and concentration of moving blood cells (CMBC) were affected in all phases by the environmental temperature (single effect of environmental temperature: p < .001 in women and men, ), with higher values at 31 °C than at 20 °C. Velocity was the only determinant significantly affected by the meal in both women (p = .032) and men (p < .001). CMBC was not modified from pre- to post-meal (p > .11 in women and men). There was a simple effect of phases in both groups for the two determinants (all p < .001).
The meal x environmental temperature x phase interaction was significant for velocity (p = .041 and p < .001 for women and men, respectively). The post-hoc results are presented in and . The only significant interaction for CMBC was the phase x environmental temperature interaction in women (p = .009).
Discussion
In this study, we confirmed that food intake reduces the skin blood flow, as previously observed in a control environment. The originality of the present study lies in the observation of microvascular adaptations in response to food intake in a warm environment. Our main observations are as follows: (1) the skin blood flow in men was higher in the warm environment than in the control environment in both the pre- and postprandial states, with similar drops from the pre- to post-state; and (2) the food intake of the women affected the blood flow level and reactivity, taken as reflections of microvascular function, in the control condition but not in the warm environment, where the skin blood flow remained unchanged after the meal compared to before.
Participants were exposed to control (20 °C) and warm (31 °C) environments for the investigation of microvascular function before and after food intake. Although the effects of a passive exposure to warmth have been extensively documented, the effects of warm exposure on the vascular function have thus far been studied without taking the effect of food intake into account. It is widely accepted that adaptation to a warm environment is characterized by an increase in skin blood flow [Citation2, Citation10]. In this study, the effect of the environmental temperature on both men and women was significant, SkBF being higher at 31 °C than at 20 °C () in relation with significant increases in both its determinants: velocity and CMBC. These data support previous work demonstrating that SkBF increases during passive body heating to favor heat loss [Citation2]. This increase in SkBF has been described as being the result of a temperature-dependent redistribution of total blood flow [Citation2], with a reduction in splanchnic and renal blood flow simultaneous to an increase in cardiac output upwards of 60% [Citation10,Citation11]. The central role of the vascular endothelium in regulating vascular homeostasis is largely mediated by NO, the major endogenous vasodilator [Citation12]. This study focused not only on the effects of warm exposure on skin blood flow taken as a reflection of microvascular function in fasting condition, as discussed above, but also on the interaction between the environmental temperature and food intake on skin blood flow.
Figure 4. Skin blood flow (PU) and its determinants in response to local heating in men before and after the meal at 20 °C (gray line) and at 31 °C (black line).
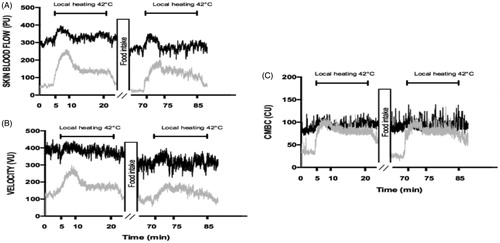
Regarding the latter, different results were observed in men and women. We showed that food intake in men reduced SkBF, particularly due to decreased velocity. Comparable results regarding the meal effect have been shown after an oral glucose tolerance test (OGTT) [Citation13] or after a meal [Citation14,Citation15] in several studies using a technique similar to the one used in this study: laser Doppler flowmetry with a local hyperthermia protocol. Other studies using different methods of microvascular exploration have shown greater discrepancies, although most have reported results similar to ours, with a postprandial peripheral reduction in SkBF. For example, a load of 75-g glucose attenuated flow-mediated dilatation one hour after ingestion in healthy subjects [Citation16]. Comparable results were also observed in a study comparing the effects of an OGTT on flow-mediated dilatation in participants with normal glucose tolerance, those with impaired glucose tolerance, and patients with diabetes [Citation17]. It should be noted that this microvascular impairment is acute, however, as four hours after a meal, microvascular function returns to baseline [Citation18]. Postprandial hyperglycemia induces oxidative stress and pro-inflammatory responses, reducing the bioavailability of NO [Citation19], which can contribute to explaining this phenomenon.
Although many studies have concluded on the deterioration of micro- and macrovascular function after food intake, other studies have shown that a meal does not affect skin microcirculation [Citation20]. Some authors have even reported an increase in blood flow after a meal [Citation21–24] or a standardized glucose load [Citation25,Citation26]. Several factors may explain these discrepancies between our results and theirs, such as the characteristics of the participants, the meal, and the use of insulin or glucose clamps.
It should also be noted that the techniques used (plethysmography, laser Doppler flowmetry, etc.) all result in different but equally relevant microcirculation information, providing potentially complementary results. Most studies that have observed an increase in postprandial blood flow have used plethysmography, a method based on evaluating venous and arterial volumes, and this technique has mainly been used in skeletal muscle, such as those of the forearm and calf, which comprise muscle, soft tissue and skin [Citation21–24]. The postprandial state is a systemic metabolic condition with specific differential effects in the various vascular territories. Thus, it is not surprising that different techniques provide apparently divergent results that may, nevertheless, reflect a single reality.
It has been well documented under normal metabolic conditions that insulin stimulates the perfusion of skeletal muscle and subcutaneous adipose tissue through improved capillary recruitment, thereby increasing blood flow mainly after meal ingestion [Citation26].
Overall, it seems that food intake coincides with a reduction in blood flow in the extremities in favor of splanchnic territories favorable to digestion and muscle for nutrient storage. In agreement with the literature, we demonstrated a decrease in fingertip skin blood flow. Thus far, the effects of food intake have only been studied without taking into account the effect of environmental temperature.
We showed that food intake in men affected skin blood flow in the warm environment. To the best of our knowledge, this is the first demonstration of such a phenomenon. The vascular impact of a meal has been widely described; however, all the studies were focused on the control climate. Only one study investigated the interaction between food intake and the effect of a hot temperature [Citation14]. The authors concluded that thermotherapy, with the participants wearing a suit perfused with 50 °C water, counteracts the effects of a fatty meal on microvascular function [Citation14]. Others have also provided evidence in favor of controlled thermotherapy as a treatment to prevent vascular dysfunction. It would appear that the physiological adaptations observed during physical activity are shared with passive heat exposure, as both increase the bioavailability of NO [Citation27] and increase blood markers like heat shock proteins [Citation28]. It is nevertheless essential to point out that this is a heat treatment dedicated to preventing and treating pathologies.
There is also evidence of the potentially deleterious effect of chronic heat exposure on carbohydrate metabolism, even in healthy subjects, one example being the recent study showing that carbohydrate metabolism was disrupted in this condition [Citation29]. Since common glycoregulatory hormones and biomarkers do not explain the exaggerated increase in blood glucose in warm environmental temperature, it has been suggested that insulin resistance could develop in this condition [Citation30]. In this regard, it would be interesting to investigate the contribution of microvascular function to this metabolic impairment.
In the women’s group, an interaction effect between the environmental condition and food intake on SkBF was observed. The post-hoc analyses revealed that food intake affected SkBF only in the control environment and not in the warm condition, in which it remained unchanged from pre- to postprandial state.
Specific thermoregulatory responses during both exercise in hot environments and passive heat exposure have been reported [Citation4,Citation31], although the thermoregulation processes are substantially similar in women and men. In our study, the postprandial skin blood flow in women was unchanged from the pre-prandial SkBF in the warm condition, whereas it was decreased in the control environment. This suggests that in the heat, evacuation has the greatest physiological priority in women and it may even overcome digestion efficiency in warm environments.
The aim of this study was to investigate the microvascular function in control and warm environments in response to food intake. We described an unsurprising altered postprandial microvascular function in the control environment. More complexity was observed in the warm environment. In men, the skin blood flow perfusion evolution from pre- to postprandial state was the same but with higher values. In women, the data suggested that thermoregulation is given priority, potentially to the detriment of glucose homeostasis.
Disclosure statement
No potential conflict of interest was reported by the author(s).
References
- Charkoudian N. Skin blood flow in adult human thermoregulation: how it works, when it does not, and why. Mayo Clin Proc. 2003;78(5):603–612.
- Johnson JM, Minson CT, Kellogg DL. Cutaneous vasodilator and vasoconstrictor mechanisms in temperature regulation. Compr Physiol. 2014;4(1):33–89.
- Kenny GP, Sigal RJ, McGinn R. Body temperature regulation in diabetes. Temperature. 2016;3(1):119–145.
- Gagnon D, Kenny GP. Does sex have an independent effect on thermoeffector responses during exercise in the heat? J Physiol. 2012;590(23):5963–5973.
- Akanji AO, Oputa RN. The effect of ambient temperature on glucose tolerance. Diabet Med. 1991;8(10):946–948.
- Dumke CL, Slivka DR, Cuddy JS, et al. The effect of environmental temperature on glucose and insulin after an oral glucose tolerance test in healthy young men. Wild Environ Med. 2015;26(3):335–342.
- Faure C, Charlot K, Henri S, et al. Impaired glucose tolerance after brief heat exposure: a randomized crossover study in healthy young men. Clin Sci. 2016;130(12):1017–1025.
- Del Pozzi AT, Miller JT, Hodges GJ. The effect of heating rate on the cutaneous vasomotion responses of forearm and leg skin in humans. Microvasc Res. 2016;105:77–84.
- Minson CT, Berry LT, Joyner MJ. Nitric oxide and neurally mediated regulation of skin blood flow during local heating. J Appl Physiol. 2001;91(4):1619–1626.
- Crandall CG, Wilson TE. Human cardiovascular responses to passive heat stress. Compr Physiol. 2015;5(1):17–43.
- Rowell LB. 2011. Cardiovascular adjustments to thermal stress. In Comprehensive Physiology (p. 967–1023). New York: American Cancer Society.
- Kellogg DL, Zhao JL, Friel C, et al. Nitric oxide concentration increases in the cutaneous interstitial space during heat stress in humans. J Appl Physiol. 2003;94(5):1971–1977.
- De Marchi S, Prior M, Rigoni A, et al. Ascorbic acid prevents vascular dysfunction induced by oral glucose load in healthy subjects. Eur J Intern Med. 2012;23(1):54–57.
- Harvey JC, Roseguini BT, Goerger BM, et al. Acute thermotherapy prevents impairments in cutaneous microvascular function induced by a high fat meal. Journal of Diabetes Research. 2016;2016:1–11.
- Rossi M, Lall K, Standfield N, et al. Impaired vasoconstriction of peripheral cutaneous blood flow in Type 1 diabetic patients following food ingestion. Diabetic Med. 1998;15(6):463–566.
- Akbari CM, Saouaf R, Barnhill DF, et al. Endothelium-dependent vasodilatation is impaired in both microcirculation and macrocirculation during acute hyperglycemia. Journal of Vascular Surgery. 1998;28(4):687–694.
- Kawano H, Motoyama T, Hirashima O, et al. Hyperglycemia rapidly suppresses flow-mediated endothelium- dependent vasodilation of brachial artery. J Am Coll Cardiol. 1999;34(1):146–154.
- Zhu W, Zhong C, Yu Y, et al. Acute effects of hyperglycaemia with and without exercise on endothelial function in healthy young men. Eur J Appl Physiol. 2007;99(6):585–591.
- Mah E, Noh SK, Ballard KD, et al. Postprandial hyperglycemia impairs vascular endothelial function in healthy men by inducing lipid peroxidation and increasing asymmetric dimethylarginine:arginine. J Nutr. 2011;141(11):1961–1968.
- Napoli R, Guardasole V, Angelini V, et al. Food and red wine do not exert acute effects on vascular reactivity. Metab Clin Exp. 2004;53(8):1081–1086.
- Eggleston EM, Jahn LA, Barrett EJ. Hyperinsulinemia rapidly increases human muscle microvascular perfusion but fails to increase muscle insulin clearance: evidence that a saturable process mediates muscle insulin Uptake. Diabetes. 2007;56(12):2958–2963.
- Fugmann A, Sarabi M, KarlströM B, et al. Blood flow is an important determinant of forearm glucose uptake following a mixed meal. Acta Diabetologica. 2003;40(3):113–117.
- Høost U, Kelbaek H, Rasmusen H, et al. Haemodynamic effects of eating: the role of meal composition. Clin Sci. 1996;90(4):269–276.
- Mutter AF, Fullwood L, Hawkins M, et al. The integrated response of the cardiovascular system to food. Digestion. 1992;52(3–4):184–193.
- Iredahl F, Högstedt A, Henricson J, et al. Skin glucose metabolism and microvascular blood flow during local insulin delivery and after an oral glucose load. Microcirculation. 2016;23(7):597–605.
- Russell RD, Hu D, Greenaway T, et al. Oral glucose challenge impairs skeletal muscle microvascular blood flow in healthy people. Am J Physiol-Endocrinol Metab. 2018;315(2):E307–E315.
- Brunt VE, Eymann TM, Francisco MA, et al. Passive heat therapy improves cutaneous microvascular function in sedentary humans via improved nitric oxide-dependent dilation. J Appl Physiol. 2016;121(3):716–723.
- Iguchi M, Littmann AE, Chang S-H, et al. heat stress and cardiovascular, hormonal, and heat shock proteins in humans. J Athl Train. 2012;47(2):184–190.
- Maley MJ, Hunt AP, Stewart IB, et al. Passive heating and glycaemic control in non-diabetic and diabetic individuals: a systematic review and meta-analysis. PLoS One. 2019;14(3):e0214223.
- Antoine-Jonville S, El Khoury D, Faure C, et al. Metabolic response to oral glucose tolerance test performed in neutral and warm environmental temperature. Int J Hyperther. 2019;36(1):624–631.
- Gagnon D, Jay O, Lemire B, et al. Sex-related differences in evaporative heat loss: the importance of metabolic heat production. Eur J Appl Physiol. 2008;104(5):821–829.