Abstract
Objective
To prospectively evaluate the efficacy of microwave ablation (MWA) for benign breast lesions (BBLs) ≥2 cm and explore the possible factors associated with the volume reduction rate (VRR) of ablated lesions.
Materials and methods
From November 2013 to December 2017, a total of 80 patients with 104 biopsy-proved BBLs larger than 2 cm in size underwent MWA. After the procedure, patients were followed up via physical and imaging examination consisting of contrast-enhanced ultrasound (CEUS) and magnetic resonance imaging (MRI). Possible factors associated with 12-month VRR were assessed, including basic patient characteristics, index lesions and parameters of ablation technique.
Results
The mean tumor size was 2.6 ± 0.6 cm (ranging 2.0–6.3 cm). Of the 104 lesions, 70 were fibroadenomas, 27 adenosis and 7 fibrocystic changes. Post-procedure CEUS or contrast-enhanced MRI showed that all lesions were completely ablated. No immediate or delayed complications were observed. All patients were followed up for more than 12 months (median follow-up 12.5 months). After MWA, the ablated lesion volume decreased significantly by 12 months (p < 0.001), with a mean volume reduction of 80.2 ± 13.1%. Multiple linear regression analysis showed that location adjacent to areola (β = 7.5, 95%CI: 1.0–13.9, p = 0.025) and location adjacent to skin (β = −7.4, 95%CI: −12.7 to −13.9, p = 0.007) were independent factors respectively associated with the increased and decreased 12-month VRR.
Conclusion
For BBLs larger than 2 cm, US-guided MWA is a favorable treatment modality, with BBLs adjacent to the areola being associated with more significant 12-month VRR after MWA.
Introduction
Asymptomatic benign breast lesions (BBLs), consisting of cysts, fibroadenomas and fibrocystic lesions, are often managed with watchful waiting [Citation1,Citation2]. Despite the documented safety of this management, in clinical practice, many BBLs are eventually treated with surgical excision due to progressive enlargement, failing to regress, breast deformity or patient discomfort during follow-up [Citation3,Citation4].
Conventional excision is safe and effective for managing BBLs. However, for large BBLs, obvious scars due to extended incision or misshapen breasts resulting from the collapsing cavity may impose psychological burdens on patients with cosmetic expectations [Citation5,Citation6]. Aiming to achieve a balance of therapeutic efficiency and cosmetic satisfaction, alternative techniques are in rapid development. As one of the most representative strategies, vacuum-assisted biopsy has been extensively used in the minimally invasive management of BBLs. However, current consensus recommendations state that only <8 cm3 BBLs (approximately 2.5 cm in diameter) should be managed with vacuum-assisted biopsy for BBLs [Citation7]. Moreover, a large number of studies reported that both irregular residuals and significant complications (such as pain, mammary duct injury, hematoma and skin subsidence) are observed in vacuum-assisted biopsies of ≥2 cm BBLs, again suggesting that it may not be suitable for treatment of ≥2 cm BBLs [Citation8–10].
Hence, minimally invasive thermal ablation techniques, including cryoablation, radiofrequency ablation (RFA), microwave ablation (MWA), high-intensity focused ultrasound (HIFU), and laser ablation (LA), are becoming robust options for the treatment of BBLs [Citation11–19]. Although all these techniques have been applied for BBLs, limited research has focused on the ablation of BBLs ≥2 cm in size. Among these methods, cryoablation led to a 65–99% volume reduction for fibroadenomas of 2.1 cm in mean size at 12–30 months follow up [Citation16,Citation20], but it was accompanied by a high incidence of tape blisters [Citation16,Citation18]. RFA plays a major role in breast cancer therapy, but there are limited reports on BBLs, especially for large ones [Citation19,Citation21–25]. The therapeutic efficacy of thermal ablation and volume reduction for large BBLs is therefore still unclear. Theoretically, MWA may be the best-suited technique to potentially achieve complete ablation of large BBLs, owing to its advantages such as higher intra-tumor temperatures, larger ablation volumes, and less ablation duration [Citation24].
The volume reduction rate (VRR), an objective measurement based on actual volume change of lesions, has been recognized as a crucial indicator and investigated in almost all studies on thermal ablation for benign breast lesions. Therefore, we performed this study to assess the clinical outcome of US-guided percutaneous MWA for BBLs ≥2cm with no less than 12 months follow-up and analyzed the possible factors associated with 12-month VRR.
Materials and methods
Patient enrollment
This prospective study is registered in ClinicalTrials.gov under the identifier number NCT-02860104. This study was approved by our Institutional Ethics Committee. Written informed consent was obtained from each patient before the procedure.
The population eligible for this study included female patients consulting in our interventional ultrasound department, with symptoms of local pain, discomfort or compression probably relating to the breast lesion, from February 2013 to December 2018. The inclusion criteria were as follows: (a) diagnosis of a benign breast lesion according to pathology confirmation from core-needle biopsy; (b) maximum lesion diameter ≥2.0 cm according to ultrasound assessment; (c) ineligibility for surgical resection due to comorbidities or refusal to undergo surgery because of cosmetic concerns; (d) age from 18 to 60 years. Exclusion criteria were: (a) patients with coagulopathy or severe cardiopulmonary diseases; (b) patients with acute or active infection; (c) ongoing pregnancy, lactation, or menstruation.
Pre-procedure evaluation
High–frequency ultrasound (US) and contrast-enhanced US (CEUS) were performed to evaluate lesion characteristics (including mobility, location, shape, number, maximum diameter, and blood supply of index lesions), with a GE LOGIQ E9 scanner (R4; GE Medical Systems US & Primary Care Diagnostics, Wauwatosa, USA) and 5.0–9.0 MHz Convex array multi-frequency transducer. US contrast agent was SonoVue (Bracco Imaging, Milan, Italy).
Contrast-enhanced MRI was performed using a 3.0-T unit (Signa Echo-Speed, GE Medical Systems, USA). The MRI scan consisted of T2W sequences, high-resolution sequences and fast-dynamic-contrast sequences. For contrast-enhanced MRI, gadopentetate dimeglumine (Magnevist; Bayer Schering, Berlin, Germany) was the contrast agent. In this study, contrast-enhanced MRI was performed before the ablation procedure because of the following reasons. First, contrast-enhanced MRI has acquired a central role in the field of diagnosis and evaluation of breast cancer due to its high sensitivity [Citation26]. It has been proven that breast MRI can detect numerous incidental cancers not detected by mammography or missed by conventional ultrasound [Citation27,Citation28]. Hence, for multiple lesions in the breast, contrast-enhanced MRI was performed to exclude the nodules with malignant potential and select the nodules requiring biopsy. Second, because contrast-enhanced MRI is predetermined to be used in evaluating the ablation efficacy after MWA, preoperative contrast-enhanced MRI was performed to provide an effective baseline reference.
Three perpendicular diameters (a-left to right, b-front to back, c-top to bottom) were measured in US imaging and lesion volume calculated according to the following equation: V = 0.525*(a*b*c). For adenosis and fibrocystic changes, despite the lack of lesion capsule, the boundary between these lesions and the surrounding tissue is clear. Therefore, the maximum lesion diameter was measured by the identification of the boundary in US imaging. via sonographic assessment, a BBL <2 mm from the skin, pectoralis or areola, was defined as a lesion adjacent to skin, pectoralis or areola.
MWA procedure
Before the ablation procedure, local anesthesia was administered via subcutaneous injection of a mixture of 2% lidocaine and 1% ropivacaine (1:1) around the mass. The hydro-dissection technique was applied to avoid pain in patients with hyperalgesia and to protect the normal tissue from thermal damage. Before MWA, a 22-gauge needle (Hakko Corporation, Nagano, Japan) with a length of 7 cm was inserted into the site between the index BBL’s margin and the adjacent skin, pectoralis, or areola under US guidance. Then, 10–30 ml saline was injected through the needle to achieve space separation ().
Figure 1. US imaging of hydro-dissection technique. (A–B) The fluid (yellow arrow) was firstly infused between BBL and skin (A), between BBL and pectoralis (B) by the fine needle (red arrow). (C) After hydro-dissection was successfully performed, the MW antenna was placed into the BBL bottom (red arrow). Then hyper-echogenicity gradually increased from the irradiating segment of the antenna (yellow arrow) at the beginning of the MWA session.
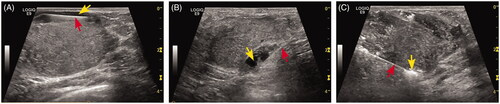
Before conducting MWA, a skin incision to insert the microwave antenna was performed. Microwave ablation was performed with a KY-2,00,02,450-MHz microwave system (Canyon Medical, Nanjing, China) and a 16-gauge, internal-cooled antenna (active tip -3mm, shaft length -10cm). Under real-time ultrasound guidance (LOGIQ E9 scanner, GE Medical Systems US, Wauwatosa, USA), the antenna was percutaneously inserted along the long axis of the index BBL and placed in the deepest section of the target lesion. For all BBLs, only one antenna was applied. As in our previous studies, the moving shot technique was applied [Citation29,Citation30]. When a heat-generated hyperechoic area around the tip was detected in US imaging, the applicator tip was pulled back slowly along the long axis of the needle until the tip arrived at the lesion margin. Then, the applicator tip was re-positioned in a more superficial section and the moving procedure repeated. The antenna tip was fixed at each ablating site for 5–10 s and then slowly moved along the long axis of the needle from the deepest to the shallowest section until the hyperechoic area completely encompassed the index BBL. A power output of 20 W and 30 W was applied during the ablation procedure. For sections with rich vascularity, the antenna was moved more slowly, with a 30 W power output.
After the procedure, cold packs were placed in the ablated regions for two hours. For half an hour, patients were constantly monitored in the observation room.
Follow-up and imaging evaluation
One day after MWA, a CEUS examination was performed to evaluate the ablation zone. Because US cannot accurately observe the spatial distribution of blood flow within the lesion, in the early stage of MWA for benign breast lesion, there is limited clinical experience in evaluating ablation efficacy using CEUS. However, CEMRI can be used to detect the spatial relationship between the lesion and the surrounding tissue, as well as the spatial distribution of blood flow, which is suitable for objectively evaluating the peri-ablation zone. Therefore, we applied CEMRI 1-3 days after the ablation to assess the ablation efficacy. Contrast-enhanced MRI has the distinct advantage in assessment of the ablation zone [Citation31], so all patients underwent an MRI scan 1-3 days after the ablation. According to our previous studies, on contrast-enhanced MRI/US imaging, irregular peripheral nodular enhancement was considered as a residual unablated section of the BBL [Citation28], and then an additional MWA session was performed. If the ablated BBL was replaced by a well-defined non-enhanced zone, the index BBL was considered to completely ablated. For patients with complete ablation, follow-up was performed by physical examination and high-frequency US at 3-month and then at 6-month intervals. In this study, all patients were followed up at least 12 months. Clinical symptoms and complication were also recorded during follow-up. As the ablated lesion gradually decreased in size over time, the volume reduction ratio (VRR) was calculated using the following equation: VRR (%) = [(initial volume – final volume) × 100]/initial volume. The lesion volume at 3, 6 and 12 months after MWA was calculated according to the same equation used in the pre-procedure evaluation, applying the diameters measured by US examination.
Clinical symptoms, complications and cosmetic outcomes were simultaneously recorded. Cosmetic characteristics including breast shape, incision scar, pigmentation, nipple and areola sensation were observed and reported as either excellent, good, acceptable, and poor [Citation32]. Palpability was ranked by the physician in three grades (1, lesions still palpable without changes in size; 2, lesions still palpable but with gradual size decrease; 3, lesions cannot be palpated).
Statistical analysis
All continuous variables are presented as mean ± standard deviation (SD) or median, and all categorical variables as frequency or percentage. ANOVA analysis was used to determine the difference between variables before and after the procedure. Multiple linear regression models were used to explore the risk factors associated with the 12-month VRR. All analyses were performed using the statistical software packages R (http://www.R-project.org, The R Foundation) and Empower Stats (http://www.empowerstats.com, X&Y Solutions, Inc., Boston, MA). A two-sided significance level of 0.05 was used to evaluate statistical significance.
Results
A total of 80 patients with 104 lesions were consecutively recruited and underwent US-guided percutaneous MWA with at least 12 months follow-up. The detailed clinical data of these patients are shown in . The mean age was 31.6 ± 9.7 years, ranging from 18 to 57 years. The median follow-up was 12.5 months, ranging from 12 to 18 months. Before ablation, BBLs could be palpable in 97.1% (101/104) of cases. All 104 index BBLs were histologically confirmed by core biopsy and successfully ablated. In terms of lesions location, there were respectively 35, 13, 16 and 40 lesions adjacent to the skin (33.7%), adjacent to pectoralis muscle (12.5%), adjacent to areola (15.4%) and in the parenchyma (36.4%). Core-needle biopsy followed by histopathologic examination showed that 70 lesions were fibroadenomas and 34 lesions were other benign breast diseases, including adenosis and fibrocystic changes.
Table 1. Basic characteristics of patients.
Treatment effectiveness
Only one lesion with a maximum diameter of 63 mm was ablated after two sessions, while the other 103 index lesions were ablated in a single session. The median ablation time was 3.8 min (ranging 1.0–18.5 min). The hydrodissection technique was applied for 75 lesions. The mean number of antenna insertions was 1.2 ± 0.5 (1.0–3.0). On US imaging, the hyperechogenicity of the ablative zone gradually decreased in the following 4–5 min after MWA and heterogeneous hypoechogenicity was observed. shows a representative case before and after the MWA procedure. Complete ablation was achieved in all BBLs as shown with CEUS and contrast-enhanced MRI. No enhancement was observed in the ablated BBL during follow up.
Figure 2. Imaging evaluation before and after MWA in one representative case. (A) BBL with 6.0 cm × 3.5 cm × 4.9 cm size showing hypoechogenicity with a clear margin on US. (B) Color Doppler flow imaging (CDFI) before MWA showing the arterial blood flow signal in the BBL parenchyma. (C) A hyper-signal in the BBL parenchyma on MRI T2-weighted images before MWA. (D–E) Contrast-enhanced MRI in axial and sagittal sections showing a hyper-enhancement in the BBL parenchyma in the arterial phase before MWA. (F) 3D visualization showing the spatial structure of BBL. (G) BBL before MWA showed hyper-enhancement in the arterial phase of CEUS. H, after MWA, a hypoechoic ablation zone with a size of 5.0 cm × 3.6 cm × 4.5 cm is shown in the tumor region by CEUS. (I) Hypo-signal in the BBL parenchyma on MRI T2-weighted images after MWA. (J–K) Contrast-enhanced MRI in axial and sagittal sections showing no enhancement in the BBL parenchyma in the arterial phase after MWA. L, 3D visualization showing the spatial structure of the ablation zone. M-P, after 12 months follow-up, the ablation debris decreased gradually to a size of 1.1 cm × 0.4 cm × 1.2 cm on T2-weighted images (yellow arrow in M) and arterial phase (yellow arrows in N, O) of MRI images and the US image (yellow arrows in P).
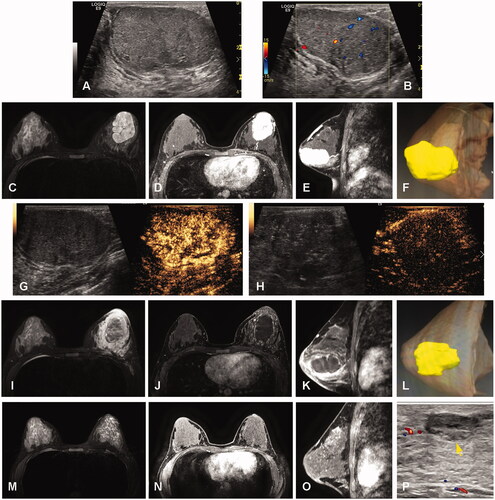
Volume reduction rate
Changes in the volume of ablated lesions at each follow-up are shown in . Nearly all cases achieved satisfying outcomes accompanied with significant volume reduction at 1 day (p < 0.02), 3 months (p < 0.001), 6 months (p < 0.001) and 12 months (p < 0.001) after MWA. In total, VRRs after ablation were 20.3 ± 3.8%, 49.8 ± 24.3%, 64.1 ± 20.4% and 80.2 ± 13.1% at the 1-day, 3-month, 6-month and 12-month follow-up respectively with significant differences between any two time points (p < 0.001, ). For BBLs of size between 2.0-3.0 cm, the VRRs in 1 day and 3, 6, 12 months after procedure were 20.3 ± 3.7%, 49.5 ± 25.3%, 63.1 ± 20.8% and 79.6 ± 13.5%, respectively; for BBLs in size of ≥3.0 cm, the VRRs in 1 day and 3, 6, 12 months after procedure were 20.4 ± 4.1%, 50.8 ± 20.9%, 67.8 ± 18.5% and 82.5 ± 11.7%, respectively. No differences were found in VRR at the follow-ups between the subgroups of lesions between 2.0 and 3.0 cm and lesions ≥3.0 cm in size (p > 0.05, ).
Figure 3. BBLs evaluation of volume reduction ratios (VRR) during follow up. (A) VRR detection at follow-up of 1 day and 3, 6, 12 months respectively. (B) VRR comparison in different BBLs sizes at the follow-up of 1 day and 3, 6, 12 months respectively. Data shown as mean ± SD. ***p < 0.001, #p > 0.05 by ANOVA multiple comparisons.
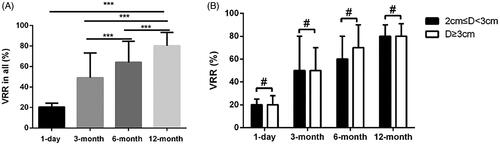
Table 2. Volumes and VRRs of BBLs before and after MWA.
Factors associated with 12-month VRR
The factors associated with 12-month VRR in univariate and multivariate analyses are shown in . In univariate analysis, age >35 years (p = 0.01) and location adjacent to the areola (p = 0.02) were associated with increased 12-month VRR (p < 0.05), whereas lesion location adjacent to skin (p < 0.001) was associated with decreased 12-month VRR. Maximum lesion diameter, pre-ablation volume, lesion location adjacent to pectoralis muscle, ablation more than one mass, ablation power, ablation energy, application of hydro-dissection and insertions number were not significantly associated with 12-month VRR (p > 0.05). Multivariate analysis showed that lesion location adjacent to the areola was associated with increased 12-month VRR (coefficient, 7.5; 95%CI: 1.0, 13.9; p = 0.025) while lesion location adjacent to skin was associated with decreased 12-month VRR (coefficient, −7.4; 95%CI: −12.7, −2.1; p = 0.007).
Table 3. Univariate and multivariate analysis of association between 12-months VRR and variables.
Cosmetic satisfaction
In terms of cosmetic satisfaction, 73/80 (91.3%) patients reported excellent cosmetic outcome and 7/80 (8.7%) patients reported good cosmetic outcome. At a median follow-up of 12.5 months, 30/104 (28.8%) ablated BBLs were not palpable and 71/104 (68.2%) ablated lesions showed a volume reduction. 75/80 (93.8%) patients reported that the ablated BBLs became gradually softer and 5/80 (6.2%) patients reported that the ablated BBLs remained as hard as before ablation.
Complications
For further evaluation, pain severity was divided into slight pain (score 1–3), moderate pain (score 4–6) and severe pain (score 7–10) according to the visual analogue scale (VAS) [Citation33]. As a result, 32 (40%) patients reported slight pain and no one reported severe pain. Moderate pain was reported in 48 (60%) patients, but the ablation procedure was tolerated well by all patients without the administration of tranquilizers before or after ablation. Although after ablation, slight skin redness and subcutaneous edema were observed in most patients, no immediate or delayed complications (skin burn, pectoralis injury, infection and nipple discharge) occurred after ablation.
Discussion
In general, common reasons for patients with BBLs to ask for medical intervention include the palpability discomfort associated with these lesions and progressive enlargement [Citation14,Citation15]. However, considering the increasing incidence of complications [Citation8,Citation34,Citation35] and the extended incisions needed [Citation14], surgical resection is difficult to accept for most patients. Ultrasound-guided microwave ablation has been proven to conformally ablate lesions without normal tissue injury and thus provides an alternative treatment modality for BBLs [Citation29,Citation30]. In this study, no obvious scar or breast deformation was observed in any case of ablation, suggesting that the superiority of MWA in improving cosmesis remains even for BBLs ≥2 cm. Therefore, US-guided MWA is a satisfactory method to treat larger BBLs in patients with concerns or anxiety regarding postoperative recovery and cosmetic effects.
Our previous studies reported that 12-month VRR of the BBLs <2 cm was about 85% after MWA, while the VRR for larger BBLs was not defined [Citation28,Citation29]. In this study, focusing on BBLs ≥2 cm, we explored the effectiveness of MWA with a relatively long follow-up (≥12-month). All lesions were completely ablated without injuries of skin, areola or pectoralis. After ablation, the mean VRR was 80.2 ± 13.1% at the 12-month follow-up. This outcome is similar to the result reported in a study on cryoablation (82.4%) [Citation15], but superior to those reported in a study on LA (40–50%) [Citation36] or HIFU (50%) [Citation37]. The reason for less volume reduction in LA and HIFU may be related to their lower complete ablation rates for BBLs ≥2 cm, both below 80%. Besides, due to the faster-rising temperature rate of MWA, the procedure duration in our study (median ablation time 3.8 min, ranging 1.0–18.5 min) is shorter than that for cryoablation (24–30 min) [Citation15,Citation20], LA (6–30 min) [Citation36] and HIFU (80–210 min) [Citation19]. Hence, MWA can produce effective shrinkage in large BBLs within a short ablation time.
Because VRR is the most worrisome effect of MWA for patients with large BBLs, we further explored VRR’s associated factors. Our results revealed that location adjacent to the areola was associated with an increase in 12-month VRR. Although there is no published study analyzing the risk factors related to the VRR of ablated BBLs, this result may be due to an increasing arterial supply in the nipple-areola complex, beneficial for local recovery and debris absorption [Citation38]. This result suggests that large BBLs adjacent to the areola tend to achieve more volume reduction, thus remarking the superiority of MWA in large BBLs in comparison to surgery. Because of the complex anatomy and vessel distribution in areola sections, resecting BBLs adjacent to the areola is prone to cause more complications than resecting BBLs in other sections during surgery. In this study, no complications occurred in the BBLs adjacent to the areola after MWA. Hence, for large BBLs adjacent to areola, MWA enables a favorable VRR without compromising treatment safety.
Moreover, our study also suggested that location adjacent to the skin was associated with a decrease in the 12-month VRR. A possible reason may be that the contraction progress was influenced by stretch from the periphery tissue (such as Cooper ligament or superficial fascia), which have yet to be proven with further and thorough studies.
To achieve the desired treatment effect for larger BBLs, several critical technical points should be noted. First, to ensure a complete ablation, comprehensive and careful US detection before the procedure and real-time US guiding during the entire procedure is necessary. Second, for BBLs adjacent to the skin, areola or pectoralis, hydro-dissection should be applied to avoid the thermal injury to surrounding tissues. Third, to achieve maximum debris absorption after MWA, the power output should be controlled in the 20–30 W range and combined with the pull-back technique.
Limitations of this study include the relatively small number of patients with BBLs ≥3cm. However, thanks to the prevalence of breast cancer screening, benign breast lesions ≥3cm in size are uncommon. In addition, the association between VRR and vascular supply of the ablated BBLs and the influence of MWA treatment on lactation were not analyzed in this study, but should be examined in further study.
Conclusions
US-guided percutaneous MWA has proved to be a promising treatment for BBLs ≥2cm. It not only achieves satisfactory effects without damage to normal tissue, but also produces significant shrinkage of ablated BBLs with adequate breast appearance. Besides, BBLs adjacent to the areola may be more suitable for undergoing MWA because of its higher 12-month VRR compared to those in other locations.
Disclosure statement
No potential conflict of interest was reported by the author(s).
Additional information
Funding
Reference
- Pearlman M, Griffin J, Swain M, et al. Practice bulletin no. 164: diagnosis and management of benign breast disorders. Obstet Gynecol. 2016;127:e141.
- Lavoue V, Fritel X, Antoine M, et al. Clinical practice guidelines from the French College of Gynecologists and Obstetricians (CNGOF): benign breast tumors – short text. Eur J Obstet Gynecol Reprod Biol. 2016;200:16–23.
- Dupont WD, Page DL. Risk factors for breast cancer in women with proliferative breast disease. N Engl J Med. 1985;312(3):146–151.
- Moskowitz M, Gartside P, Wirman JA, et al. Proliferative disorders of the breast as risk factors for breast cancer in a self-selected screened population: pathologic markers. Radiology. 1980;134:289–291.
- Jacob MM. Application of reduction mammaplasty in treatment of giant breast tumour. Br J Plast Surg. 2000;53(3):265–266.
- Lai HW, Lin HY, Chen SL, et al. Endoscopy-assisted surgery for the management of benign breast tumors: technique, learning curve, and patient-reported outcome from preliminary 323 procedures. World J Surg Oncol. 2017;15(1):19.
- Hahn MM, Krainick-Strobel T, Toellner J, et al. Interdisciplinary consensus recommendations for the use of vacuum-assisted breast biopsy under sonographic guidance: first update 2012. Ultraschall Med. 2012;33(4):366–371.
- Sperber F, Blank A, Metser U, et al. Diagnosis and treatment of breast fibroadenomas by ultrasound-guided vacuum-assisted biopsy. Arch Surg. 2003;138(7):796–800.
- Shu L, Jiali Z, Fulin Z. Efficacy of ultrasound-guided vacuum-assisted mammotome excision for management of benign breast diseases: analysis of 1267 cases. J South Med Univ. 2017;37(8):1121–1125.
- Shaylor SD, Heller SL, Melsaether AN, et al. Short interval follow-up after a benign concordant MR-guided vacuum assisted breast biopsy-is it worthwhile?. Eur Radiol. 2014;24(6):1176–1185.
- Kovatcheva R, Guglielmina JN, Abehsera M, et al. Ultrasound-guided high-intensity focused ultrasound treatment of breast fibroadenoma—a multicenter experience. J Ther Ultrasound. 2015;3(1):1–8.
- Bo RY, Kim HJ, Shin KM, et al. Serial ultrasound findings after laser ablation for benign breast lesions on long-term follow-up: implications for evaluation of procedural success. Photomed Laser Surg. 2015;33(8):404–408.
- Fine RE, Staren ED. Percutaneous radiofrequency-assisted excision of fibroadenomas. Am J Surg. 2006;192(4):545–547.
- Zhou W, Wang R, Liu X, et al. Ultrasound-guided microwave ablation: a promising tool in management of benign breast tumours. Int J Hyperthermia. 2016;10:1–8.
- Kaufman CS, Bachman B, Littrup PJ, et al. Cryoablation treatment of benign breast lesions with 12-month follow-up. Am J Surg. 2004;188(4):340–348.
- Kaufman CS, Littrup PJ, Freeman-Gibb LA, et al. Office-based cryoablation of breast fibroadenomas with long-term follow-up. Breast J. 2005;11(5):344–350.
- Zhang W, Li JM, He W, et al. Ultrasound-guided percutaneous microwave ablation for benign breast lesions: evaluated by contrast-enhanced ultrasound combined with magnetic resonance imaging. J Thorac Dis. 2017;9(11):4767–4773.
- Caleffi M, Filho DD, Borghetti K, et al. Cryoablation of benign breast tumors: evolution of technique and technology. Breast. 2004;13(5):397–407.
- Teh HS, Tan SM. Radiofrequency ablation - a new approach to percutaneous eradication of benign breast lumps. Breast J. 2010;16(3):334–336.
- Kaufman CS, Bachman B, Littrup PJ, et al. Office-based ultrasound-guided cryoablation of breast fibroadenomas. Am J Surg. 2002;184(5):394–400.
- Earashi M, Noguchi M, Ai M, et al. Radiofrequency ablation therapy for small breast cancer followed by immediate surgical resection or delayed mammotome excision. Breast Cancer. 2007;14(1):39–47.
- Grotenhuis BA, Vrijland WW, Klem TM. Radiofrequency ablation for early-stage breast cancer: treatment outcomes and practical considerations. Eur J Surg Oncol. 2013;39(12):1317–1324.
- Leylek AM, Whitman GJ, Vilar VS, et al. Radiofrequency ablation for breast cancer. Tech Vasc Interv Radiol. 2013;16(4):269–276.
- Liang P, Yu J, Yu XL, et al. Percutaneous cooled-tip microwave ablation under ultrasound guidance for primary liver cancer: a multicentre analysis of 1363 treatment-naive lesions in 1007 patients in China. Gut. 2012;61(7):1100–1101.
- Yu J, Liang P, Yu XL, et al. US-guided percutaneous microwave ablation of renal cell carcinoma: intermediate-term results. Radiology. 2012;263(3):900–908.
- Orel SG, Schnall MD. MR imaging of the breast for the detection, diagnosis, and staging of breast cancer. Radiology. 2001;220(1):13–30.
- Berg WA, Gutierrez L, NessAiver MS, et al. Diagnostic accuracy of mammography, clinical examination, US, and MR imaging in preoperative assessment of breast cancer. Radiology. 2004;233(3):830–849.
- Kuhl CK, Strobel K, Bieling H, et al. Supplemental breast MR imaging screening of women with average risk of breast cancer. Radiology. 2017;283(2):361–370.
- Yu J, Chen B-H, Zhang J, et al. Ultrasound guided percutaneous microwave ablation of benign breast lesions. Oncotarget. 2017;8(45):79376–79386.
- Xu J, Wu H, Han Z, et al. Microwave ablation of benign breast tumors: a prospective study with minimum 12 months follow-up. Int J Hyperthermia. 2018;35(1):253–261.
- Vilar VS, Goldman SM, Ricci MD, et al. Analysis by MRI of residual tumor after radiofrequency ablation for early stage breast cancer. AJR Am J Roentgenol. 2012;198(3):W285–291.
- Mauri G, Sconfienza LM, Pescatori LC, et al. Technical success, technique efficacy and complications of minimally-invasive imaging-guided percutaneous ablation procedures of breast cancer: a systematic review and meta-analysis. Eur Radiol. 2017;27(8):3199–3210.
- Williamson A, Hoggart B. Pain: a review of three commonly used pain rating scales. J Clin Nurs. 2005;14(7):798–804.
- Połom K, Murawa D, Nowaczyk P, et al. Vacuum-assisted core-needle biopsy as a diagnostic and therapeutic method in lesions radiologically suspicious of breast fibroadenoma. Rep Pract Oncol Radiother. 2010;16(1):32–35.
- Plantade R, Hammou JC, Gerard F, et al. [Ultrasound-guided vacuum-assisted biopsy: review of 382 cases]. J Radiol. 2005;86(9 Pt 1):1003–1015.
- Dowlatshahi K, Wadhwani S, Alvarado R, et al. Interstitial laser therapy of breast fibroadenomas with 6 and 8 year follow-up. Breast J. 2010;16(1):73–76.
- Cavallo MB, Pediconi F, Anzidei M, et al. High-intensity focused ultrasound in breast pathology: non-invasive treatment of benign and malignant lesions. Expert Rev Med Devices. 2015;12(2):191–199.
- Petrus V, Frank R. The blood supply of the breast revisited. Plast Reconstr Surg. 2016;137:1388–1397.