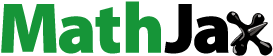
Abstract
Background
Clinical differentiation between pigmented basal cell carcinoma (BCC) and seborrheic keratosis (SK) can sometimes be difficult. Noninvasive diagnostic technologies, such as thermal imaging, can be helpful in these situations. This study explored the use of dynamic thermal imaging (DTI), which records thermal images after the application of external thermal stimuli (heat or cold) for the differential diagnosis of pigmented BCC and SK.
Materials and methods
Twenty-two patients with pigmented BCC and 15 patients with SK participated in this study. Dynamic thermal images of lesions (pigmented BCC or SK) and control sites (contralateral normal skin) were recorded after the heat and cold stimuli. Temperature changes in the region of interest (ROI) are plotted as a thermal response graph. After fitting an exponential equation to each thermal response graph, the rate constants were compared between groups (pigmented BCC versus control, SK versus control).
Results
The thermal response graphs revealed that the average temperature of pigmented BCC showed faster thermal recovery to baseline than the control site. There was a significant difference in the rate constants of the fitted exponential equations between the pigmented BCCs and the control sites (p<.001). However, we did not find a significantly different thermal recovery pattern between SK lesions and control sites.
Conclusions
DTI can be used as a diagnostic tool for distinguishing pigmented BCC from SK by comparing thermal recovery patterns between target lesions (pigmented BCC or SK) and the control site.
Introduction
Basal cell carcinoma (BCC) is the most common malignancy worldwide, and its incidence is increasing [Citation1]. Pigmented BCC lesions are particularly common in Asians, accounting for more than 50% of all BCCs, compared to only 6% in Caucasians [Citation2,Citation3]. Sometimes clinical differentiation between pigmented BCCs and benign pigmented lesions such as seborrheic keratosis (SK) can be difficult [Citation2,Citation3]. Before histological confirmation, noninvasive diagnostic techniques, including dermoscopy, can be helpful in these situations [Citation4]. Unfortunately, dermoscopic findings can be misleading in certain circumstances, and other auxiliary diagnostic tools may be necessary [Citation5].
Thermal imaging or thermography is an emerging noninvasive in vivo diagnostic technique used in skin tumor research [Citation6]. It is a sensing method that records the infrared radiation emitted by a target (e.g., skin), usually acquired by non-contact thermal cameras [Citation6,Citation7]. It is based on the hypothesis that the temperature distribution of the skin surface displayed in thermal imaging reflects the pathophysiological changes in the underlying structure [Citation6–8]. Thermal imaging can be divided into two subtypes: static (passive) and dynamic (active) [Citation7,Citation8]. The static method acquires thermal imaging without prior stimulation [Citation6–8]. On the other hand, dynamic thermal imaging (DTI) records thermal imaging after the application of external thermal stimuli (heat or cold) [Citation6–8]. With such stimuli, it is possible to enhance the thermal contrast and improve the accuracy of thermal imaging [Citation6,Citation7].
Most studies used DTI to detect malignant melanomas or distinguish melanomas from benign melanocytic nevus [Citation7–10]. Only a few studies have applied this technology to BCCs. To the best of our knowledge, no studies have focused on pigmented BCCs. We previously applied the DTI technique to a photocarcinogenesis mouse model and actinic keratosis (AK) patients [Citation11,Citation12]. This study attempted to explore the use of our modified DTI system as a diagnostic tool for distinguishing pigmented BCC from SK.
Methods
Patients
From February 2020 to December 2020, we recruited voluntary participants from patients who visited the Department of Dermatology at Korea University Guro Hospital with suspected pigmented BCC or SK lesions. We excluded patients with co-existing inflammatory or other malignant skin diseases and those who had received therapeutic or cosmetic laser treatment or phototherapy. We also excluded patients with severe hyperkeratotic or irritated lesions. Patients did not receive any compensation for participation in this study (Supplementary Figure 1).
After obtaining consent from the patient, we obtained clinical photographs of the lesion (pigmented BCC or SK). We then moved the patient to a room under ambient conditions (23 °C and 50 ± 5% humidity). After a period of acclimatization (approximately 5–10 min), DTI of the suspicious lesion and control site (normal skin) was performed. As in the previous study, we chose clinically normal skin on the contralateral side as the control site [Citation12]. For instance, if the suspected pigmented BCC was on the left infraorbital cheek, we chose the clinically normal skin on the right infraorbital cheek as a control. After DTI completion, the patient was moved to the procedure room for a routine skin biopsy. An experienced dermatopathologist reviewed the histopathological slides using hematoxylin and eosin staining. We only included patients with histopathologically confirmed pigmented BCC and SK for the final data analysis. The Institutional Review Board (IRB) of Korea University Guro Hospital approved this study protocol (2016GR0140 version 1.2).
Dynamic thermal imaging
We used a modified version of the DTI system used in previous studies [Citation11,Citation12]. Thermal images were obtained using a thermal imaging camera (FLIR® A615, FLIR Systems, Wilsonville, OR), which has an infrared resolution of 640 × 480 pixels. This camera measures the temperature variation of the subject with a frame rate of 50 Hz, a noise equivalent temperature difference (NETD) <0.05 °C × at 30 °C and accuracy of ± 2% of the overall temperature reading. For thermal provocation (heat and cold stimuli), we used a thermoelectric cooler (TECH3S, Thorlabs, Newton, NJ) and a resistance temperature detector (TH100PT, Thorlabs). In addition, we used a heater controller (TC200-EC, Thorlabs) to ensure that the stabilized heat and cold stimuli were applied to the target. The size of the heating and cooling regions was approximately 2.0 × 2.5 cm. This was sufficient to cover the target lesion (pigmented BCC or SK, typically about 1.0 cm in diameter) with 0.5–1.0 cm beyond the target’s clinical margin.
The patient remained in a supine position during the acquisition of thermal imaging. Initially, the lesion (pigmented BCC or SK) was heated to the target temperature (40 °C). After reaching the target temperature, the heater was immediately removed, and the thermal change of the lesions was recorded for another five minutes. After approximately a 5 min resting period, the lesion was cooled until the target cooling temperature (15 °C) was reached. Similar to the heat stimulus, we recorded the thermal change of the lesion for five minutes after removing the cold stimulus. The same procedure was repeated for the control sites (). All acquired thermal images were analyzed using the FLIR systems image processing software. We manually selected the region of interest (ROI) and analyzed the thermal changes in the ROI before and after the thermal stimulus.
Figure 1. Representative clinical photographs and thermal images. (A) Pigmented basal cell carcinoma (BCC); clinical photo (left), the thermal image immediately after removal of the heat stimulus (middle), and the thermal image immediately after removal of the cold stimulus (right). (B) The contralateral control site of pigmented BCC; clinical photo (left), the thermal image immediately after removal of heat stimulus (middle), and the thermal image immediately after removal of cold stimulus (right). (C) Seborrheic keratosis (SK); clinical photo (left), the thermal image immediately after removal of heat stimulus (middle), and the thermal image immediately after removal of cold stimulus (right). (D) The contralateral control site of SK; clinical photo (left), the thermal image immediately after removal of heat stimulus (middle), and the thermal image immediately after removal of cold stimulus (right).
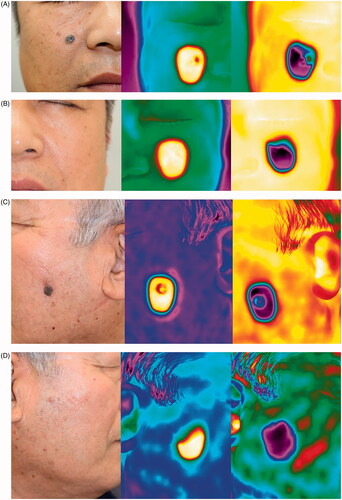
Statistical analysis
The temperature change in the ROI was calculated and plotted as a graph (thermal response graph). Two thermal response graphs for each target were drawn: one after the heat stimulus (heat thermal response graph) and the other after the cold stimulus (cold thermal response graph). Then, an exponential equation was fitted to each thermal response graph using GraphPad Prism version 9 program (GraphPad Software, San Diego, CA, USA). For heat thermal response graphs, we used the following exponential equation with x as time (second) and y as temperature (°C) ():
Figure 2. The heat (A) and cold (B) thermal response graphs (the first 12 s) of a 57-year-old male patient with pigmented basal cell carcinoma (BCC) on his right cheek. The red line depicts the thermal change of pigmented BCC and the blue line depicts the change on the control site (left normal cheek). Faster thermal recovery to baseline temperature (dashed line) was observed for pigmented BCC compared to the control site. For analysis, an appropriate exponential equation (dotted line) was fitted to each thermal response graph. The rate constants were compared between the groups (pigmented BCC versus control) for statistical analysis. The heat (C) and cold (D) thermal response graphs (the first 12 s) of an 80-year-old male patient with seborrheic keratosis (SK) on his left cheek. The red line depicts the thermal change of SK and the blue line depicts the change of the control site (right normal cheek). At glance, there was no difference in the thermal recovery pattern between SK and control site. The above-mentioned method was applied for statistical analysis.
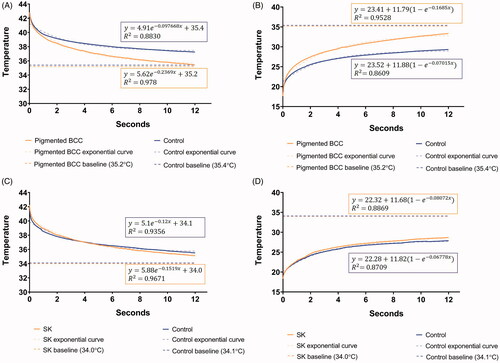
As for cold thermal response graph, the following exponential equation is used ():
The rate constant (value ‘k’) was used to compare the thermal recovery pattern (faster or slower) between the lesion and control site as in previous studies. First, the distribution of the rate constants was tested for normality using the Kolmogorov–Smirnov test and Shapiro–Wilk test. We used the unpaired Student’s t-test to compare the rate constants between the lesion (pigmented BCC or SK) and the control site. Statistical analyses were performed using SPSS version 20.0 (IBM, Armonk, NY, USA).
Results
Patient characteristics
A total of 37 patients (22 with pigmented BCC and 15 with SK) were included in the study. All diagnoses were histopathologically confirmed. There were 23 men and 14 women in the study group. The median patient age was 72 years (range, 38–93 years). The demographic data of each patient group (pigmented BCC and SK) are shown in . All patients successfully completed the DTI process and did not show serious complications, except for a transient mild heating or cooling sensation.
Table 1. Demographic data of the study population.
Dynamic thermal imaging of pigmented basal cell carcinoma
Before heat and cold stimuli, the baseline thermal condition of a typical pigmented BCC was approximately 0.2 °C lower than its control site. However, the static thermal imaging results were highly variable among the subjects. After the heat and cold stimuli, we were able to draw separate thermal response graphs of the ROI for each pigmented BCC and control site. In general, the average recorded temperature of the ROI for pigmented BCC returned to baseline approximately 17 s after the target temperature (40 or 15 °C) was reached. However, it took a longer time for the control site to return to the baseline temperature. Using the thermal response graphs, we observed that the temperature of pigmented BCC showed faster thermal recovery to baseline compared to the control site (). As described above, exponential equations were fitted to each thermal response graph, and the rate constants were compared between groups (pigmented BCC and control sites). The rate constants showed a normal distribution according to the Kolmogorov–Smirnov and Shapiro–Wilk tests (p>.05). In the heat thermal response graph, the mean rate constant of pigmented BCC lesions was 0.246 ± 0.0256 (mean ± standard deviation [SD]), and that of the control sites was 0.120 ± 0.0265 (mean ± SD). A significant difference in the rate constants between the pigmented BCC lesions and control sites in the heat thermal response graph was observed (p<.001). In the cold thermal response graph, the mean rate constant of the pigmented BCC lesions was 0.145 ± 0.0359 (mean ± SD), and that of the control sites was 0.0736 ± 0.0184 (mean ± SD). There was also a statistically significant difference between the mean rate constants of the pigmented BCC lesions and control sites observed in the cold thermal response graph (p<.001) ().
Figure 3. Statistical comparison of rate constants between groups. Comparison between rate constants calculated from both (A) heat and (B) cold thermal response graphs of pigmented basal cell carcinoma (BCC) lesions and their control sites shows a significant difference (*p<.001). On the other hand, a comparison between rate constants calculated from both (C) heat and (D) cold thermal response graphs of seborrheic keratosis lesions and their control sites shows no significant difference (p > .05).
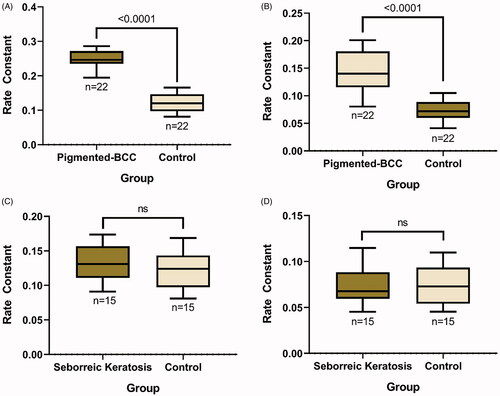
Dynamic thermal imaging results of seborrheic keratosis
The baseline thermal condition of a typical SK was approximately 0.1 °C lower than its control site. Therefore, the static thermal imaging results of SK and pigmented BCC were similar. Similar to pigmented BCCs, the static thermal imaging results were highly variable among the subjects. After the heat and cold stimuli, we were able to draw thermal response graphs of the ROI for each SK and control site. We did not observe any different thermal recovery patterns between the SK and control sites in the thermal response graph (). Further analysis by fitting exponential equations showed that the mean rate constant of the SK lesions was 0.132 ± 0.0272 (mean ± SD) and that of the control sites was 0.124 ± 0.0273 (mean ± SD) in the heat thermal response graph. In the cold thermal response graph, the mean rate constant of the SK lesions was 0.0734 ± 0.022 (mean ± SD), and that of the control sites was 0.0754 ± 0.0214 (mean ± SD). There were no significant differences in the thermal recovery patterns between SK and the control sites in either the heat or cold thermal response graphs ().
Discussion
BCC is the most common skin cancer, and its incidence is continuously rising in the USA, Europe, Australia and Asia [Citation1]. The cost burden of BCC is also increasing [Citation1]. In Korea, the incidence of BCC has increased dramatically in recent years [Citation13], and the importance of early BCC detection has gained attention. In Asians, pigmented BCC accounts for more than 50% of all BCC cases [Citation3]. For instance, 78% of BCCs were pigmented according to a single-center retrospective study in Korea [Citation14]. The BCC pigmentation is due to increased melanin deposition and increased melanocytes [Citation15]. However, the exact roles of melanin and melanocytes in BCC pathogenesis have not been clearly elucidated. A recent study showed that pigmented BCCs in Asians tend to be less aggressive than non-pigmented BCCs [Citation16]. Although pigmented BCCs may have a more favorable prognosis, it can sometimes be clinically difficult to differentiate from other tumors, such as SK [Citation2,Citation17]. We performed a pilot study to evaluate the usefulness of pre-biopsy DTI in the differential diagnosis of pigmented BCC and SK.
Although skin biopsy is the standard of care for diagnosing BCC [Citation4], noninvasive diagnostic tools can be helpful for clinicians to guide patients to undergo invasive biopsy. Dermoscopy, reflectance confocal microscopy, optical coherence tomography (OCT), Raman spectroscopy, high-resolution ultrasonography and thermal imaging are examples of noninvasive diagnostic techniques used for the diagnosis of BCC [Citation4,Citation6]. With remarkable technological advancements in thermal cameras, thermal imaging has gained attention in skin tumor research [Citation6,Citation7]. Historically, thermal imaging was first introduced to the skin cancer field by Brasfield et al. [Citation18], who applied this technique to various benign and malignant tumors, including melanoma. In static thermal imaging, benign skin tumors are generally known to show hypothermic patterns that appear colder than the surrounding normal skin [Citation8]. In contrast, malignant lesions are hyperthermic compared to their surroundings, often referred to as a ‘hot spot’ [Citation8]. Unfortunately, the static method only provides the spatial thermal distribution of the target, and early research has shown poor results with a high rate of false negatives [Citation7,Citation19,Citation20]. With the introduction of DTI (thermal imaging before and after applying external thermal stimuli), it became possible to analyze both spatial and temporal variations in the temperature of the subject, resulting in improved diagnostic accuracy [Citation7,Citation20]. Currently, with DTI and digital imaging processing, we can selectively analyze thermal images from a specific time after a thermal stimulus and discard unnecessary temperature variations of the target [Citation7,Citation11,Citation12,Citation20–22]. Researchers are now using DTI not only as a diagnostic supporting tool but also in monitoring treatment success during patient follow-up [Citation6,Citation23].
As mentioned above, DTI is based on the hypothesis that the surface (e.g., skin) temperature distribution reflects pathophysiological changes in subsurface structures [Citation6–8]. When a malignant skin tumor develops, the tissue becomes thermodynamically different from healthy tissue, which leads to different thermal responses to external thermal stimuli (heat or cold) [Citation7,Citation11,Citation12]. Abnormal cell proliferation, excess metabolism and increased blood flow in malignant tissue are suggested as pathophysiological changes responsible for altered DTI results [Citation7]. Our previous study showed that increased blood vasculature during skin carcinogenesis is the key change attributable to a faster thermal response after external stimuli [Citation11]. A recent study using dynamic OCT showed that blood vessels in BCC lesions appeared significantly more superficial than those in AK, squamous cell carcinoma (SCC), and adjacent normal skin [Citation24]. We believe that this superficially located neoplastic microvasculature contributes to different DTI results of pigmented BCCs compared to normal controls. On the other hand, there were no significant differences between DTI results from SKs (known to lack neoplastic microvasculature) and controls. Therefore, we propose to use the difference in DTI results between the target lesion (pigmented BCC or SK) and control as a diagnostic tool to differentiate pigmented BCC from SK.
To our knowledge, only a few clinical studies have applied DTI to BCCs. In two studies, the authors grouped BCCs with melanomas and SCCs as ‘malignant conditions’, and DTI results were not analyzed specifically for BCC [Citation25,Citation26]. One study performed dynamic video thermography on BCCs and AKs [Citation27]. Inconsistent with this study, this previous article showed that BCC lesions generally did not show fast thermal recovery time after the cold stimulus [Citation27]. This may be due to the differences in DTI recordings, methods of analyses or characteristics of study patients. For instance, Di Carlo et al. [Citation27] defined positive fast thermal recovery only when the temperature of the lesion returned to baseline for less than two seconds. On the other hand, we used a specific value (rate constant) calculated from the thermal response graph for comparison between the lesion and normal control. Further studies are required to validate the use of DTI in diagnosing BCC, including pigmented types.
An interesting characteristic of this study is that both heat and cold stimuli were used and analyzed during the DTI process. Most previous studies utilized only one type of thermal stimulus during DTI [Citation7,Citation8,Citation11,Citation12,Citation20,Citation25–27]. With DTI data from both heat and cold stimuli, it is possible to strengthen the differential diagnosis of pigmented BCCs and SKs compared to DTI data from single stimuli (heat or cold). We believe that future DTI studies should use both heat and cold stimuli whenever possible.
Disclosure statement
No potential conflict of interest was reported by the author(s).
Additional information
Funding
References
- Cameron MC, Lee E, Hibler BP, et al. Basal cell carcinoma: epidemiology; pathophysiology; clinical and histological subtypes; and disease associations. J Am Acad Dermatol. 2019;80(2):303–317.
- Tay SH, Chan MMF, Oh CC. Dermoscopy for pigmented basal cell carcinoma in Asian patients. Hong Kong J Dermatol. 2019;27(1):5–11.
- Gloster HM, Jr., Neal K. Skin cancer in skin of color. J Am Acad Dermatol. 2006;55(5):741–760.
- Cameron MC, Lee E, Hibler BP, et al. Basal cell carcinoma: contemporary approaches to diagnosis, treatment, and prevention. J Am Acad Dermatol. 2019;80(2):321–339.
- Gao YY, An XJ, Yang J, et al. Seborrheic keratosis mimicking basal cell carcinoma under dermoscopy: a case report. Chin Med J. 2020;133(17):2139–2140.
- Magalhaes C, Vardasca R, Mendes J. Recent use of medical infrared thermography in skin neoplasms. Skin Res Technol. 2018;24(4):587–591.
- Çetingül MP, Herman C. Quantification of the thermal signature of a melanoma lesion. Int J Therm Sci. 2011;50(4):421–431.
- Magalhaes C, Vardasca R, Rebelo M, et al. Distinguishing melanocytic nevi from melanomas using static and dynamic infrared thermal imaging. J Eur Acad Dermatol Venereol. 2019;33(9):1700–1705.
- Santa Cruz GA, Bertotti J, Marin J, et al. Dynamic infrared imaging of cutaneous melanoma and normal skin in patients treated with BNCT. Appl Radiat Isot. 2009;67(7–8): S54–S58.
- Santa Cruz GA, Gonzalez SJ, Bertotti J, et al. First application of dynamic infrared imaging in boron neutron capture therapy for cutaneous malignant melanoma. Med Phys. 2009;36(10):4519–4529.
- Baek YS, Kim J, Han G, et al. Application of dynamic thermal imaging in a photocarcinogenesis mouse model. Int J Hyperthermia. 2018;34(7):961–968.
- Baek YS, Kim J, Song JY, et al. Dynamic thermal imaging on actinic keratosis patients: a preliminary study. Skin Res Technol. 2019;25(2):211–216.
- Oh CM, Cho H, Won YJ, et al. Nationwide trends in the incidence of melanoma and non-melanoma skin cancers from 1999 to 2014 in South Korea. Cancer Res Treat. 2018;50(3):729–737.
- Ro KW, Seo SH, Son SW, et al. Subclinical infiltration of basal cell carcinoma in Asian patients: assessment after Mohs micrographic surgery. Ann Dermatol. 2011;23(3):276–281.
- Brankov N, Prodanovic EM, Hurley MY. Pigmented basal cell carcinoma: increased melanin or increased melanocytes? J Cutan Pathol. 2016;43(12):1139–1142.
- Moon HR, Park TJ, Ro KW, et al. Pigmentation of basal cell carcinoma is inversely associated with tumor aggressiveness in Asian patients. J Am Acad Dermatol. 2019;80(6):1755–1757.
- Kim HS, Kim TW, Mun JH, Song M, et al. Basal cell carcinoma-mimicking lesions in Korean clinical settings. Ann Dermatol. 2014;26(4):431–436.
- Brasfield RD, Laughlin JS, Sherman RS. Thermography in the management of cancer. Ann N Y Acad Sci. 1964;121:235–247.
- Cristofolini M, Perani B, Piscioli F, et al. Uselessness of thermography for diagnosis and follow-up of cutaneous malignant melanoma. Tumori. 1981;67(2):141–143.
- Herman C, Cetingul MP. Quantitative visualization and detection of skin cancer using dynamic thermal imaging. J Vis Exp. 2011;51(51):2679.
- Bonmarin M, Le Gal FA. Lock-in thermal imaging for the early-stage detection of cutaneous melanoma: a feasibility study. Comput Biol Med. 2014;47:36–43.
- Bonmarin M, Le Gal FA. A lock-in thermal imaging setup for dermatological applications. Skin Res Technol. 2015;21(3):284–290.
- Laino L, Elia F, Desiderio F, et al. The efficacy of a photolyase-based device on the cancerization field: a clinical and thermographic study. J Exp Clin Cancer Res. 2015;34:84.
- Sigsgaard V, Themstrup L, Theut Riis P, et al. In vivo measurements of blood vessels' distribution in non-melanoma skin cancer by dynamic optical coherence tomography - a new quantitative measure? Skin Res Technol. 2018;24(1):123–128.
- Godoy SE, Hayat MM, Ramirez DA, et al. Detection theory for accurate and non-invasive skin cancer diagnosis using dynamic thermal imaging. Biomed Opt Express. 2017;8(4):2301–2323.
- Godoy SE, Ramirez DA, Myers SA, et al. Dynamic infrared imaging for skin cancer screening. Infrared Phys Techn. 2015;70:147–152.
- Di Carlo A, Elia F, Desiderio F, et al. Can video thermography improve differential diagnosis and therapy between basal cell carcinoma and actinic keratosis? Dermatol Ther. 2014;27(5):290–297.