Abstract
Aim: In oncology, thermal therapy is the application of external heat to fight cancer cells. The goal of whole-body thermal treatment (WBTT) is to raise the patient’s core temperature to 39–42 °C, and represents the only thermal treatment modality that can act on both the primary tumor and distant metastases. However, WBTT carries potential risks for toxicity when applied without accurate thermometry and monitoring.
Methods: ElmediX has developed a medical device, HyperTherm, to deliver long-term controlled and accurate WBTT (41.5 °C, up to 8 h). The safety of the device and thermal treatment protocol was initially evaluated in minipigs, and we present the confirmation of tolerability of WBTT in dogs with advanced cancer, in combination with a reduced dose of radiotherapy or chemotherapy.
Results: Thermometry in liver, rectum, and tumor confirmed a homogeneous heating of these body parts. Monitoring of clinical parameters showed acceptable and reversible changes in liver, cardiac, muscle and coagulation parameters, as was expected. Combination of WBTT with both radiotherapy and chemotherapy only caused some low-grade adverse events.
Conclusion: We conclude that our findings support the safe use of HyperTherm-mediated WBTT for canine patients with advanced malignancies. They also tend to support a genuine therapeutic potential for long-term WBTT which needs to be confirmed on a larger dog patient population. Combined with previously reported safety results in minipigs, these contribute to support the ongoing clinical evaluation of WBTT in advanced human cancer patients.
Introduction
Therapeutic hyperthermia or thermotherapy is generally regarded as a treatment in which mean body temperature is higher than normal [Citation1]. Many types of devices, technology platforms and procedures to apply heat and heating protocols have been developed [Citation2–4]. In contrast to local or regional thermal treatment, whole-body thermal treatment (WBTT) raises the patient’s core body temperature and is therefore the only thermal treatment modality that has the potential to act on both primary tumor and distant metastases. WBTT seems to be promising as an adjuvant cancer therapy for several reasons. First, tumor cell-based experiments have confirmed a time- and temperature-dependent cytotoxic effect when temperatures between 41 °C and 47 °C were applied [Citation5]. The same cell-killing effect is generally assumed in in vivo situations [Citation6]. Moreover, several clinical studies underlined the importance of duration and extent of supranormal temperature achieved during thermal treatment [Citation7,Citation8]. Second, WBTT somewhat mimics the physiological response of fever, and can be associated with the same therapeutic benefits: e.g., stimulation of the immune system, enhancing immune surveillance and contributing to long term protection from tumor growth and development of metastases [Citation9]. Third, WBTT has been shown to be a powerful adjuvant to chemotherapy and radiotherapy, by inducing changes in the oxidative metabolism and thereby sensitizing cancer cells to the cytotoxic effects of these treatment modalities [Citation5,Citation10]. In addition, this adjuvant effect to chemotherapy has been attributed to the ability of systemic thermal treatment to modulate the tumor microenvironment through increased blood flow and vessel permeability, decreased negative interstitial pressure, and modulation of the extracellular matrix architecture [Citation5].
Beside its promising benefits, WBTT also represents the most drastic form of thermal therapy, due to the need to apply it under sedation or general anesthesia and the potential risks of toxicities like neuropathy, hepato- and nephrotoxicity as well as severe cardiovascular stress [Citation11]. Therefore, WBTT requires accurate thermometry to ensure optimal therapeutic temperature while simultaneously preventing side effects and damage to healthy tissue [Citation2]. A classical WBTT procedure will heat the body to a high temperature (41–42 °C) for a short period of time (30 min to 2 h) to limit toxicity. Recently, there has been a shift toward fever-range WBTT, which is in essence a treatment with longer duration at lower temperatures (39–40 °C for 6–8 h) [Citation2,Citation3]. With the latter protocol, researchers observed improvement of anti-tumor effects without toxicity to normal tissues, as well as a delay in tumor growth and a significant reduction in the incidence of lymph node metastasis [Citation12].
Here, we have evaluated a novel WBTT procedure in canine cancer patients. Dogs with naturally occurring cancer mimic human disease much better than rodents, especially the aspect of the complex biology of cancer metastasis and recurrence [Citation13]. Because of long-time parallel evolution of the two species, the canine and human genome show much greater similarities when compared to the murine genome [Citation14]. Additionally, canine patients have an intact immune system and are exposed to much of the same environmental risk factors. All these factors show that strong similarities exist in fundamental features of cancer biology between dog and human cancers and therefore the dog patient is a viable surrogate species for the conduct of safety, tolerability and preliminary efficacy in human clinical trials [Citation14]. Apart from their valuable contribution in comparative oncology, dogs are also frequently used for WBTT studies [Citation15–24], suitable anesthesia protocols [Citation17], temperature distribution [Citation18,Citation19], combination with different chemotherapy (CT) [Citation20–22,Citation25,Citation26] and combination with radiation therapy (RT) [Citation23,Citation24].
ElmediX research and development program has already demonstrated the safety and tolerability of HyperTherm-mediated WBTT (41.5 °C, up to 8 h) in minipigs [Citation27]. Additionally, we have determined the optimal temperature range using tumor cell-based studies (data not shown). In this study, we aim to explore the tolerability of WBTT in dogs with naturally occurring advanced cancer either as single therapeutic modality or in combination with a reduced dose of RT or CT. Promising results allowed progression into a first-in-human clinical trial (MATTERS: Safety study of whole-body hyperthermia for advanced cancer patients; NCT04467593. https://clinicaltrials.gov/).
Materials & methods
Patient selection and study design
The study was performed at Oncovet – Veterinary Cancer Center (Lille, France) with the cooperation of Oncovet Clinical Research (OCR). The protocol was approved by OCR’s ethical committee and the informed consent was obtained from the owner of each dog. Adult dog patients were selected based on a diagnosis of advanced and/or metastatic malignancy. All dog patients were examined to confirm normal functioning of the cardiovascular, respiratory and central nervous system, as well as normal liver, renal and bone marrow function.
Based on previous cell-based assays and the minipig study it was foreseen that dog patients would receive two WBTT sessions of 41.5 °C for 8 h with a three-week interval. The first WBTT of the first patient was delivered without any additional treatment; all following thermal treatments were combined with other modalities. External beam megavoltage RT (6 MV, Precise, Elekta) was combined using hypofractionated dose-fractions (5–6 Gy every 1–3 weeks, for a total dose of 10–24 Gy), immediately following the WBTT session, while still under anesthesia, for optimal synergistic effect between the two modalities. All RT treatments were planned using a 3 D conformal technique based on the study staging CT study (Oncentra, Elekta). CT (carboplatin) was administered at a reduced dose (250 instead of 300 mg/m2) [Citation28] during the plateau-phase of the WBTT session. One week after each treatment a follow-up visit was planned, with the final follow-up in week 7 after first WBTT. However, due to the inclusion of different tumor types and careful evaluation of each patient’s clinical conditions and needs, extra treatments with chemotherapy or radiotherapy and/or extra WBTT sessions were given, leading to multiple clinical protocol variations ().
Table 1. Patient characteristics, tumor types and individual treatment regimens.
ElmediX whole-body thermal treatment medical device
To deliver a long-term controlled and accurate WBTT, ElmediX has designed and developed an automated experimental medical device for large animals (‘HyperTherm G3.5’, which has then been further adapted for human clinical use under the name of TempoCure®), which allows very accurate body temperature control (to 0.1 °C) that stems from the interaction between precise sensors and the used hardware/software. A schematic overview of HyperTherm G3.5 level architecture is presented in . Briefly, four units can be identified: (1) the treatment chamber; (2) the sensing unit; (3) the regulation unit; and (4) the data logging unit. The working principle of the device is the regulated convective exchange of heat between air, forcedly circulated in the treatment chamber, and the animal. The sensing unit consists of a set of temperature sensors, placed in the treatment chamber and at strategic anatomical locations on the animal, namely on the skin above the liver, in the liver, in the tumor (when possible) and in the rectum. Originally optical sensors were used, however from the second WBTT session of dog n°4 onwards, it was decided to replace them with thermocouples for more precise and stable temperature control. The sensors collect temperature data which forms the input for the regulation unit. The latter finely tunes the temperature of the air in the treatment chamber by means of an accurate proportional, integral and derivative (PID) controller. At start of thermal treatment, heat was delivered to the dog patient by the circulating warm air, allowing to reach the targeted treatment body temperature in a controlled and safe manner. Thereafter, the device automatically regulates the heat required to maintain the animal at the selected temperature. The PID controller also ensures instant cooling of the circulating air when the threshold temperature was exceeded. All measured temperatures as well as the heating power output of the regulation unit, were visualized in real-time and saved by a data logging unit for off-line processing. Of all temperature sensors, the one that was implanted in the liver was selected for steering of the PID controller, which was validated as the most temperature-sensitive organ during previous minipig experiments [Citation27].
Figure 1. Schematic diagram of ElmediX HyperTherm G3.5 medical device components. Four units can be identified in the device: (1) the treatment chamber; (2) the sensing unit; (3) the regulation unit; and (4) the data logging unit. Hot air is injected at high speed in a treatment chamber. Air temperature is modified, to reach and maintain a liver/body temperature of 41.5 °C. All optical fiber sensor data are logged simultaneously on a personal computer for control and data reporting.
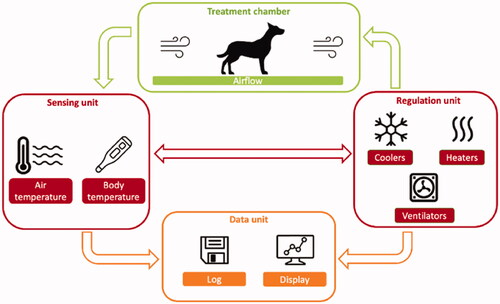
Whole-body thermal treatment procedure, monitoring and sampling
The WBTT was strictly performed under general anesthesia with the supervision of an EBVS European Specialist in Veterinary Anesthesia and Analgesia. Ketamine-propofol mixture (1.0 and 2.0 mg/kg, respectively) was used to induce the anesthesia. Dog patient was placed inside the HyperTherm and respective sensors were connected. Dog patient was intubated and mechanically ventilated to regulate the evacuation of CO2. Effective neuromuscular blockage was required to prevent counter breathing. Anesthesia was maintained with isoflurane (1.75–3%) in O2 (1–3 L/min). Each treatment was composed of three phases: (1) heating phase (at a rate of 1 °C increase per 30 min); (2) plateau phase at a body temperature of 41.5 °C; and (3) cooling phase. Vital parameters were monitored during the entire procedure, in function of the need for deep anesthesia with good muscle relaxation, cardiovascular support with adequate fluid and vasopressor adjustment, and ventilatory compensation of metabolic changes. Blood sampling was performed once during the heating phase, and every 2 h after stabilization (plateau phase − 41.5 °C), at 24 h, 48 h and 14 days after WBTT. These blood samples were analyzed in house for routine hematological and biochemical parameters, cardiac troponin I and electrolytes. Additionally, coagulation times (activated partial thromboplastin time (aPTT) and prothrombin time (PT)) were determined. After the treatment, animals were hospitalized for 2 more days to continue fluid therapy, and to allow clinical monitoring and blood sampling.
Quality of life
Quality of Life (QoL) was an important parameter in this study. Therefore, the dog owner was asked to complete a QoL questionnaire at different timepoints during the study. The questions checked for the dog’s physical status (appetite, sleep, activity, pain), emotional behavior (interaction with the owner, changes in behavior, happiness) as well as for the owner’s anxiety in relation to the dog’s symptoms, disease and treatment.
Adverse events
Adverse Events (AEs) have been graded according to the Veterinary Cooperative Oncology Group-Common terminology criteria for adverse events following chemotherapy or biological antineoplastic therapy in dogs and cats (VCOG-CTCAE v1.1) [Citation29].
Results
Case selection
A total of six dog patients have been recruited in this study, see .
Device performance
While allowing evaluation of the tolerability of WBTT, the present study has also enabled the validation of the accuracy of the HyperTherm G3.5 performance. The maximum liver, rectal and tumor temperatures during WBTT of each patient are depicted in , in which for each dog, one representative WBTT session has been selected. Generally, the patient body temperature was between 36 and 38 °C at the start of the heating phase, and it took between 2 and 4 h to reach the set-point of 41.5 °C. Major factors affecting the animal heating process were the size of the dog and amount of fat tissue as well as a thick furry coat. During the plateau-phase (41.5 °C), there is a fluctuation of the animal body temperature around the designed set-point. This is a result of multiple technical factors including the accuracy of the sensors, tuning of the device parameters and the body mass of the patient. The data collected during the initial WBTT runs, and the switch to a different sensing technology, allowed to fine tune the system, resulting in an improved temperature regulation accuracy (, as compared to ).
Figure 2. Comparison of the maximal liver, rectal and tumor temperatures monitored during whole-body thermal treatment. (A) dog n°1, WBTT1 (there was no monitoring of the rectal temperature with ElmediX sensors during this case, only with a standard hospital probe); (B) dog n°2, WBTT2; (C) dog n°3, WBTT1; (D) dog n°4, WBTT1; (E) dog n°5, WBTT1; (F) dog n°6, WBTT3. During plateau-phase, there is a fluctuation of temperature around the set-point (41.5 °C). Over time, the temperature accuracy improved (A and B as compared to F). By adding extra heating patches on superficial tumors located far from the core, treatment temperature at 41.5 °C was reached (F).
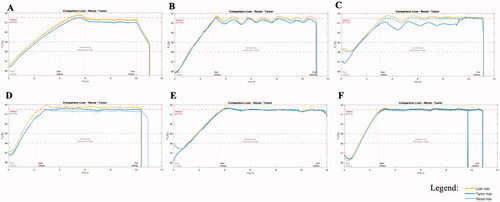
The addition of a local heating patch and a thermally insulating layer onto the tumor allowed reaching target treatment temperature even in superficial tumors located on the extremities. The effects of this solution on tumor temperature can be appreciated comparing (osteosarcoma located on the distal tibia) and (anal sac carcinoma). The temperature was proven to be uniform across liver, rectal and tumor measurements. The uniformity of temperature, intended as maximal variation across the temperature recorded in the different organs, improved over the course of the study from an initial value of ± 0.15 °C in the first dog, to ± 0.05 °C in the last treatment of the last dog.
Clinical safety parameters
Hematological changes
During the WBTT procedure, there was a progressive drop in the 3 main cellular components of the blood: red blood cells (RBC), white blood cells (WBC) and platelets (). RBC and platelet values recovered within 24–48 h. For WBC, there was a transient rise noticeable, peaking 24 h after thermal treatment, before slowly decreasing back to normal values.
Biochemical changes
The heatmap depicted in presents an overview of all monitored blood parameters during the study. Per WBTT session (ranging from one day before to 14 days after WBTT) and per animal, all parameters maximally deviating from their normal range were selected. These aberrant parameters were then graded according to the same guidelines also adopted for grading the adverse events (VCOG-CTCAE v1.1) [Citation29].
Figure 3. Heatmap depicting all biochemical, electrolyte and coagulation parameters measured during whole-body thermal treatment (WBTT) and their seriousness. Per WBTT session (ranging from the day before to 14 days after the respective session) and per animal, all parameters maximally deviating from their normal ranges were selected. The seriousness of the deviation was than graded according to the VCOG-CTCAE v1.1 guideline. As shown, most parameters remained within their reference ranges. Grade 3 and 4 disturbances occur in selected parameters (liver (AST), skeletal muscle (creatine kinase), cardiac (Troponin I) and coagulation (aPTT and D-dimers)) though not systematically in each patient or during each WBTT session.
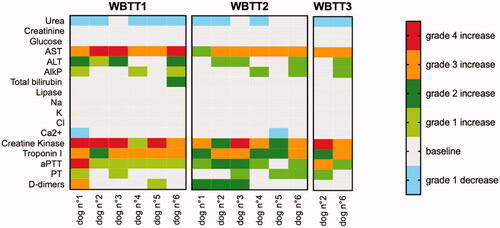
Overall, most blood parameters remained within their normal ranges during WBTT sessions. Slight decreases in urea and ionized calcium were noted in 4 dogs. Observed changes in other blood parameters reflect the impact on 4 organs or systems. First, there was a noticeable effect on the liver, as illustrated by an increase in the enzyme alanine aminotransferase (ALT, ). Simultaneously, there was a mild increase of alkaline phosphatase (ALP) in 6 out of 14 treatments and a stronger increase in the enzyme aspartate transaminase (AST) in all the 14 treatments. The latter can represent stress on skeletal muscles and the heart. This second observation is supported by the increase of creatine kinase (CK) levels in all 14 treatments. Third, there was an increase in cTnI. Last, there was also mild prolongation of blood clotting times. For prothrombin time (PT) this happened in 6 of the 14 sessions, whereas for activated partial thromboplastin time (aPTT) during 12 of the 14 sessions. In one case however, there was an important increase in aPTT, combined with spontaneous bleeding at the catheter site and from the nose. All changes in blood parameters were clinically non-significant and transient. They peaked at 24–48 h after WBTT and were found to be back within their normal ranges at the first follow-up visit at 14 days (no blood sampling after 48 h). It is very likely that the values normalized much faster, as it was observed in the first dog patient. This was the only animal that had a control visit 6 days after WBTT, finding that all parameters were already restored at that time ( and ).
Figure 4. Effect of whole-body thermal treatment (WBTT) on selected hematological parameters. Red blood cells (A), white blood cells (B) and platelets (C) were determined the day before treatment, every two hours during the treatment plateau-phase, and then 24 h, 48 h, and 14 days after the treatment. For all parameters, a temporary drop is seen during the WBTT session. This is a dilution effect of the blood, caused by the required intense fluid therapy (10 ml/kg/h). All parameters recover quickly after the end of the procedure. Only the values of first WBTT session are shown; the same trend was seen in the other treatment sessions.
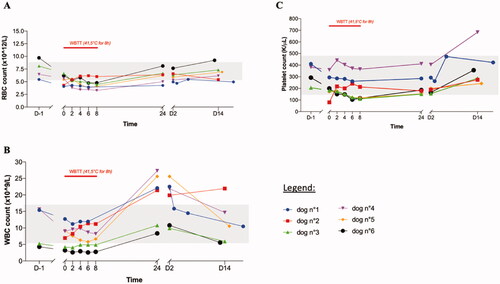
Figure 5. Effect of whole-body thermal treatment (WBTT) on selected biochemical parameters. Alanine aminotransferase (ALT (A)), aspartate aminotransferase (AST (B)), creatine kinase (CK (C)) and cardiac Troponin I (cTnI (D)) were determined the day before treatment, every two hours during the treatment plateau-phase, and then 24 h, 48 h, and 14 days after the treatment. These results confirm a heat-induced stress of WBTT on liver, skeletal muscle and heart which is mild, clinically well tolerated by the patient and transient in nature.
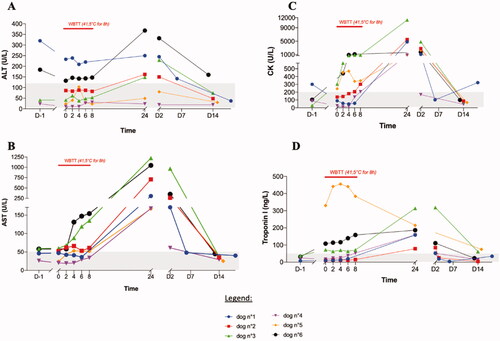
Hemodynamic parameters (blood pressure (BP), heart rate (HR), data not shown) were subject to physiological changes (heating of the body) and/or pharmacological intervention (anesthetics, chemotherapy injection for dogs n°4 and n°5). To maintain homeostasis these parameters were corrected and kept within normal ranges with deepening anesthesia, ventilatory correction, fluid therapy and vasopressors.
Adverse events and QoL
A total of 44 adverse events (AEs) have been reported. An overview of the 10 Serious Adverse Events (SAEs) considered to be treatment-related is presented in . All cardiovascular events were considered as anesthesia- and treatment-related, which means they were transient, and recovered promptly after awakening of the patients. Unfortunately, dog n°3 died at the start of the third WBTT session. Though the liver sensor was placed under ultrasound guidance by an EBVS European Specialist in veterinary Diagnostic Imaging while patient is under anesthesia, a traumatic lesion originated with severe liver hemorrhage and subsequent death of the animal, despite of an emergency surgical intervention. Postmortem pathological analysis revealed underlying chronic hepatitis, representing a risk factor for bleeding. Other AEs (grade 1 and 2, data not shown) which were probably, or possibly, related to the treatment included: skin disorders, among which dermatitis, erythema, and alopecia, occurring in some dogs. These could be treatment-induced as they appeared on contact points like the back of the head and the back of the animals. Lastly, general signs seen in the patients when waking up were dysphoria, joint pain, tachypnea, and disorientation. Regarding the dog’s QoL, this parameter tended to decline at inclusion compared to 6 months before the start of the study. However, after the first WBTT, the score improved, and it improved further and stabilized during the study.
Table 2. Overview of Serious Adverse Events (SAEs) registered during the study, their gradation according to VCOG-CTCAE v1.1 and the relation to the WBTT procedure.
Discussion
This veterinary phase I study represented an additional step to support the development of an accurate and safe WBTT using ElmediX’ HyperTherm medical device. After having documented the tolerability of the treatment procedure in female Aachen minipigs [Citation27], the primary goal of this study was to investigate the feasibility of long duration WBTT (41.5 °C, up to 8 h) in combination with other cytotoxic treatments while monitoring tumor response and overall survival in dogs with naturally occurring cancer. A total of 6 dog patients diagnosed with advanced and/or metastasized cancer were included in the study. All patients had normal cardiovascular, respiratory, hepatic, renal and coagulation function. Regarding the technical performance of the HyperTherm-mediated WBTT, this study allowed the validation of the therapeutic treatment procedure, and the accurate control of long-term WBTT (41.5 °C for 6 h) for all patients, and all WBTT sessions.
The close monitoring of clinical parameters and AE’s during this study, points at several aspects to consider when planning further clinical development. The first observation was a transient decrease in hematocrit, leukocytes, platelets and RBC, as a result of hemodilution because of perioperative intensive intravenous fluid therapy (10 ml/kg/h). The prompt recovery of all these parameters back to their basal values within 24 h after WBTT supports this finding. As an exception, the number of neutrophils peaked and practically doubled 48 h after WBTT, compared to the values before start of treatment. The same effect was previously described [Citation16], and postulated to be linked to neutrophil demargination from the circulating pool after catecholamine release, induced by thermal treatment [Citation30]. No plasma cortisol levels were determined during this study, so this hypothesis could not be confirmed.
Second, reports in the literature indicate diffuse intravascular coagulation (DIC) induced by thermal treatment, as also observed in human patients suffering from heat stroke [Citation31]. DIC is a consumptive coagulopathy defined by the presence of thrombocytopenia, prolonged clotting times, fibrin degradation products (FDG) in plasma, decreased fibrinogen concentration, red blood cell fragments in circulation and/or spontaneous bleeding [Citation32]. In our study, one WBTT session was conservatively stopped because of prolonged clotting times (aPTT 2.5-fold higher than upper limit, PT 1.8-fold higher than upper limit), fibrine degradation products (3.4-fold upper limit) accompanied with spontaneous bleeding. However, fibrinogen concentration (3.3 g/L; reference: 1.5–4 g/L) and platelets (261 K/L; reference: 148–484 K/L) were within normal range at time of ending (6 h of plateau-phase). This coagulopathy was transient and displayed some, but not all the characteristics of DIC, and a prompt recovery without interventional treatment was observed. This event occurred during the first WBTT of the first patient and has not been observed in any of the following treatments of the same patient, or from the other dogs. It was postulated to be linked to a short but significant overshoot in the liver temperature at 6 h of treatment.
Another observation made in human heat stroke patients is the damage to organs, namely the liver, kidneys, muscles, heart, central nervous system, and the lungs [Citation31]. Our study results confirmed the heat-induced stress on some of these organs, though clinically well tolerated by the patients and transient in nature. Regarding the liver, we observed a maximum of 3.5-fold increase in ALT values. AST values increased more significantly, even to 24-fold in one patient. The latter could represent damage to myocytes and/or cardiac muscle. CK levels peaked to 30-fold increase, which can indicate cardiac stress and/or damage to skeletal muscle, possibly because of the long fixation in dorsoventral position during WBTT sessions. However, potassium levels always remained within normal ranges, proving that the strong rise in CK-levels is not linked to severe rhabdomyolysis, as was seen in other studies [Citation33]. During our study there was an elevation of cTnI, up to maximum of 15 times the upper limit, but these values recovered to normal ranges within 14 days after treatment. CTnI is one of the most sensitive and specific cardiac biomarkers in dogs and indicated myocardial stress [Citation34], however in humans it can also be released after long duration physical exercise even in the absence of myocardial injury [Citation35]. Short strenuous exercise did not raise cTnI in normal dogs [Citation36]. Electrocardiograms during anesthesia showed no abnormalities and additionally, 3 of the 6 dogs were evaluated by echocardiography 14 days after having received their second WBTT. All the evaluations showed normal heart function without any abnormalities. The body responds to increased temperatures during thermal treatments by increasing heart rate, increasing respiratory ventilation and with vasodilatation to lose the excess heat and CO2. This represents a stress condition that is maintained over a long period of time, which makes it comparable to strenuous exercise. The absence of any other measurable cardiac abnormalities and the fast drop of cTnI supports the idea that its increase was exercise-induced. No AEs were observed when combining long-term WBTT with a reduced dosage of RT or CT. Especially in the case of CT combination, with patients receiving a dosage of 240 or 250 mg/m2 carboplatin during the plateau phase of WBTT, no renal toxicity was observed. The number of AE and SAE diminished clearly between the patients as the experience with WBTT increased with no SAE in the last two patients.
Although the current study was not designed, nor powered, to investigate clinical efficacy of the proposed treatment modality, some preliminary findings were noticed. First, the clinical response was overall good for each dog with a reduction in pain, good general condition, and improved QoL compared to study inclusion time. Regarding post treatment survival, strict endpoint determination is complex and influenced by many parameters, not in the least by the owners who have the final decision for euthanasia. As explained earlier, dog n°3 died during the start of the third treatment. The five other patients were euthanized because of progression of disease with worsening of symptoms and a subsequent strong decay in QoL (dog n°1: facial deformity and fistula forming, dog n°2: paraplegia, dog n°4 and n°5: severe pain at tumor site and for dog n°6: paraneoplastic progressive polyneuropathy with secondary mega-esophagus). Three dogs stand out regarding overall survival, given the low dose of standard of care therapy (either CT or RT) they received, they did reach a survival time on the upper limit or exceeding of what was expected with a full dose of SOC therapy.
Taken together, our findings show that the HyperTherm-mediated WBTT and procedures can be safely used for the treatment of canine patients with advanced malignancies. The principal gains compared to previously used techniques [Citation19], are 1) the safety that arises from automatization of the procedure, which is based on continuous thermometry and consequently 2) the duration at which we can incubate the patient. As observed during previous and ongoing preclinical work, this duration of incubation at 41.5 °C is a decisive factor in order to obtain the anti-cancer effect of thermal treatment (unpublished data). Our positive preliminary clinical benefit results tend to support a genuine therapeutic potential for long-term WBTT which needs to be confirmed on a larger canine patient population with spontaneous advanced tumors. In a follow-up study (which is currently being initiated), we will investigate in-depth the mode of action (vascular changes, immune response, effect on metastases and DNA damage repair) and clinical efficacy of WBTT in a specific patient population (head and neck tumors and resected hemangiosarcoma). Nevertheless, combined with previously reported safety and tolerability results in minipigs [Citation27], these results greatly contribute to the support of ongoing clinical evaluation of WBTT in advanced cancer human patients.
Disclosure statement
No potential conflict of interest was reported by the authors.
References
- Jha S, Sharma PK, Malviya R. Hyperthermia: role and risk factor for cancer treatment. Achiev Life Sci. 2016;10(2):161–167.
- Kok HP, Cressman ENK, Ceelen W, et al. Heating technology for malignant tumors: a review [internet]. Int J Hyperthermia. 2020;37(1):711–741.
- Jia D, Liu J. Current devices for high-performance whole-body hyperthermia therapy. Expert Rev Med Devices. 2010;7(3):407–423.
- Lee SY, Fiorentini G, Szasz AM, et al. Quo vadis oncological hyperthermia (2020)? frontiers in oncology. Frontiers Media S.A. 2020;10:1690.
- Hildebrandt B, Wust P, Ahlers O, et al. The cellular and molecular basis of hyperthermia. Crit Rev Oncol Hematol. 2002;43(1):33–56. Elsevier;
- Dieing A, Ahlers O, Hildebrandt B, et al. The effect of induced hyperthermia on the immune system. Prog Brain Res. 2007;2007:137–152.
- Sherar M, Liu FF, Pintilie M, et al. Relationship between thermal dose and outcome in thermoradiotherapy treatments for superficial recurrences of breast cancer: data from a phase III trial. Int J Radiat Oncol Biol Phys. 1997;39(2):371–380.
- Jones EL, Oleson JR, Prosnitz LR, et al. Randomized trial of hyperthermia and radiation for superficial tumors. J Clin Oncol. 2005;23(13):3079–3085.
- Baronzio GF, della SR, D’Amico M, et al. Effects of local and whole body hyperthermia on immunity. 2013; [cited 2021 Mar 4]; Available from: https://www.ncbi.nlm.nih.gov/books/NBK6083/.
- Oei AL, Kok HP, Oei SB, et al. Molecular and biological rationale of hyperthermia as radio- and chemosensitizer. Adv Drug Delivery Rev. 2020;163–164:84–97.
- Kerner T, Hildebrandt B, Ahlers O, et al. Anaesthesiological experiences with whole body hyperthermia. Int J Hyperthermia. 2003 [cited 2021 Feb 2;19(1):1–12.
- Kraybill WG, Olenki T, Evans SS, et al. A phase I study of fever-range whole body hyperthermia (FR-WBH) in patients with advanced solid tumours: correlation with mouse models. Int J Hyperthermia. 2002;18(3):253–266.
- Gordon I, Paoloni M, Mazcko C, et al. The comparative oncology trials consortium: using spontaneously occurring cancers in dogs to inform the cancer drug development pathway. PLoS Med. 2009;6(10):e1000161.
- Park JS, Withers SS, Modiano JF, et al. Canine cancer immunotherapy studies: linking mouse and human. j Immunotherapy Cancer. 2016;4(1):7.
- Macy DW, Macy CA, Scott RJ, et al. Physiological studies of whole-body hyperthermia of Dogs1. Cancer Res. 1985;45(6):2769–2773.
- Page RL, Meyer RE, Thrall DE, et al. Cardiovascular and metabolic response of tumour-bearing dogs to whole body hyperthermia. Int J Hyperthermia. 1987;3(6):513–525.
- Meyer RE, Page RL, Thrall DE, et al. Determination of continuous atracurium infusion rate in dogs undergoing whole-body hyperthermia. Cancer Res. 1986;46(11):5599–5601.
- Thrall DE, Page RL, Mcleod DA. Use of insulation to reduce extremity temperature nonuniformity during whole body hyperthermia in Dogs1. Cancer Research. 1987;47(22):5880–5882.
- Thrall DE, Page RL, Dewhirst MW, et al. Temperature measurements in normal and tumor tissue of dogs undergoing whole body hyperthermia. Cancer Res. 1986;46(12 Pt 1):6229–6235.
- Page RL, Mcentee MC, Williams PL, et al. Effect of whole body hyperthermia on carboplatin disposition and toxicity in dogs. Int J Hyperthermia. 1994;10(6):807–816.
- Riviere JE, Page RL, Dewhirst M, et al. Effect of hyperthermia on cisplatin pharmacokinetics in normal dogs. Int J Hyperthermia. 1986;2(4):351–358.
- Novotney CA, Page RL, Macy DW, et al. Phase I evaluation of doxorubicin and whole-body hyperthermia in dogs with lymphoma. J Vet Intern Med. 1992;6(4):245–249.
- Thrall DE, Prescott DM, Samulski T. V, et al. Radiation plus local hyperthermia versus radiation plus the combination of local and whole-body hyperthermia in canine sarcomas. Int J Radiat Oncol Biol Phys. 1996;34(5):1087–1096.
- Gillette SM, Dawson CA, Scott RJ, et al. Whole-body hyperthermia combined with hyperfractionated irradiation of the thorax in dog: acute physiological response. Int J Hyperthermia. 1993;9(3):369–382.
- Page RL, Macy DW, Ogilvie GK, et al. Phase III evaluation of doxorubicin and whole-body hyperthermia in dogs with lymphoma. Int J Hyperthermia. 1992;8(2):187–197.
- Hauck ML, Price GS, Ogilvie GK, et al. Phase I evaluation of mitoxantrone alone and combined with whole body hyperthermia in dogs with lymphoma. Int J Hyperthermia. 1996;12(3):309–320.
- Carneiro MW, Brancato L, Wylleman B, et al. Safety evaluation of long-term temperature controlled whole-body thermal treatment in female aachen minipig. Int J Hyperthermia. 2021;38(1):165–175.
- Lavalle GE, de Campos CB, Bertagnolli AC, et al. Canine malignant mammary gland neoplasms with advanced clinical staging treated with carboplatin and cyclooxygenase inhibitors. In Vivo. 2012;26(3):375–379.
- Veterinary cooperative oncology group-common terminology criteria for adverse events (VCOG-CTCAE) following chemotherapy or biological antineoplastic therapy in dogs and cats v1.1. 2011.
- Summers C, Rankin SM, Condliffe AM, et al. Neutrophil kinetics in health and disease [internet]. Trends in Immunology. 2010;31(8):318–324.
- Mozzini C, Xotta G, Garbin U, et al. Non-exertional heatstroke: a case report and review of the literature. Am J Case Rep. 2017;18:1058–1065.
- Diehl KA, Crawford E, Shinko PD, et al. Alterations in hemostasis associated with hyperthermia in a canine model. J. Hematol. 2000;64(4):262–270.
- Lassche G, Frenzel T, Mignot MH, et al. Thermal distribution, physiological effects and toxicities of extracorporeally induced whole-body hyperthermia in a pig model. Physiol Rep. 2020;8(4):366.
- Porter A, Rozanski E, Price LL, et al. Article evaluation of cardiac troponin I in dogs presenting to the emergency room using a point-of-care assay. Can Vet J. 2016;57(6):641–645.
- Baker P, Leckie T, Harrington D, et al. Exercise-induced cardiac troponin elevation: an update on the evidence, mechanism and implications. IJC Heart Vasculature. 2019;22:181–186.
- Wakshlag JJ, Kraus MS, Gelzer AR, et al. The influence of High-Intensity moderate duration exercise on cardiac troponin I and C-Reactive protein in sled dogs. J Vet Intern Med. 2010;24(6):1388–1392.