Abstract
Purpose
The present study identified predictors of intrahepatic progression-free survival (ihPFS) after ultrasound (US)-guided percutaneous microwave ablation (MWA) for colorectal liver metastases (CRLMs) and developed a nomogram to predict ihPFS.
Methods
From January 2013 to December 2018, a total of 314 patients (224 men and 90 women; mean age, 57.1 ± 11.8 years; range, 23–86 years) with 645 CRLMs (mean diameter, 16.6 ± 6.5 mm; range, 6.0–45.0 mm) treated with US-guided percutaneous MWA were included and analyzed. The average number of CRLMs per patient treated was 2 (range, 1–8). A nomogram to predict ihPFS was developed based on a multivariable Cox model. Validation of the nomogram was performed using the concordance index (C-index), calibration curves, and decision curve analyses.
Results
The 1-, 2-, and 3-year cumulative ihPFS rates were 59.0%, 38.9%, and 30.8%, respectively. Maximal CRLM size, number of CRLMs, ablative margin, primary tumor lymph node status, and chemotherapy were five independent prognostic factors for ihPFS. The C-index of the nomogram was 0.702 (CI: 0.681–0.723). A risk classification system that perfectly classified the patients into three risk groups was constructed. The median ihPFS of patients in the low-, intermediate-, and high-risk groups was 36.3 months (95% CI: 21.4–51.1), 13.4 months (95% CI: 12.1–14.6), and 3.8 months (95% CI: 2.3–5.3), respectively.
Conclusion
The nomogram and risk classification system will facilitate the personalized assessment of ihPFS for patients receiving US-guided percutaneous MWA for CRLMs.
Introduction
Liver metastases occur in approximately 50% of colorectal cancer (CRC) patients and account for at least two-thirds of CRC-related deaths [Citation1]. Surgical resection is the most effective treatment for colorectal liver metastases (CRLMs), but less than 30% of patients with CRLMs are suitable for surgical resection at the time of diagnosis [Citation1]. A total of 40–70% of patients develop new metastases after surgery, and most of these patients cannot undergo repeat resection [Citation2]. Therefore, various local ablative approaches, such as radiofrequency ablation (RFA) and microwave ablation (MWA), are used to treat CRLMs [Citation3]. Ablation may be used alone or with surgery to treat CRLMs as long as all visible tumors are eradicated [Citation4]. The addition of thermal ablation alone or with surgery to systemic chemotherapy results in significant prolongation of progression-free survival (PFS, 16.8 months versus 9.9 months) and overall survival (OS, 45.6 months versus 40.5 months) compared to patients treated with chemotherapy alone [Citation5,Citation6]. MWA offers the theoretical advantages of a larger ablation zone, a shorter ablation time, and less susceptibility to the “heat sink” effect compared to RFA [Citation7,Citation8]. Research showed that complete ablation was achieved for >90% of patients undergoing MWA, and local tumor progression (LTP) occurred in only 2.7–9.0% of lesions [Citation7,Citation9]. Unfortunately, nearly half of the patients experienced recurrence at remote hepatic sites [Citation9].
Metastatic disease has a major prognostic impact on CRC patients. Intrahepatic disease progression, including LTP and intrahepatic recurrence (iHR), remains a major problem, especially when early recurrence is associated with worsened OS [Citation10]. Knowledge of prognostic factors becomes essential for follow-up and therapeutic adjuvant strategies. The prognostic risk factors for patients are heterogeneous because of a variety of clinical and tumor-specific factors [Citation9,Citation11]. Individual patient decision-making requires better tools for assessing prognosis to provide patients with appropriate treatment options [Citation12]. A nomogram is a statistical tool that generates a simple graphical representation of a complex statistical model. Nomograms help clinicians graphically determine individualized, patient-specific survival estimates rapidly without performing any complex calculations [Citation11]. Compared to traditional scoring systems, the advantages of prognostic nomograms include the ability to accurately account for continuous variables and take the specific value of each factor into consideration to provide the probability of a particular outcome [Citation13].
The current nomograms in most studies are used to predict disease-free survival and OS after surgical resection of CRLMs [Citation10–15], but few nomograms are used to predict intrahepatic progression-free survival (ihPFS) after MWA for CRLMs. The present study summarized experience with the ultrasound (US)-guided percutaneous MWA of CRLMs based on a relatively large sample size at a single center. The purposes of this study were to identify prognostic risk factors for ihPFS after MWA for CRLMs and develop a nomogram to predict ihPFS. The current study created a nomogram to predict ihPFS instead of OS, which may be influenced by postoperative chemotherapy and treatment patterns of recurrent tumors [Citation10].
Materials and methods
Patient selection
We performed a retrospective review of a prospectively collected database of patients who underwent US-guided percutaneous MWA for CRLMs at a single center between January 2013 and December 2018. Written informed consent was obtained from each patient before ablation. This research study was performed retrospectively from data obtained for clinical purposes. This retrospective study was approved by our institutional ethics committee (the Ethics committee approved protocol number is 2021ZSLYEC-383). The following inclusion criteria were used: (1) patients with CRC confirmed by a pathology study; (2) patients with liver-limited metastases and no major vascular incursion confirmed by contrast-enhanced computed tomography (CECT), contrast-enhanced magnetic resonance imaging (CEMRI) or positron emission tomography-computed tomography (PET-CT) (the interval between CECT, CEMRI or PET-CT before MWA and MWA was within one month); and (3) number of CRLMs ≤9 and size of CRLMs ≤5 cm. The following exclusion criteria were used: (1) Child-Pugh class C or severe coagulation disorder (platelet count <50 × 103/µl, prothrombin time >20 s); (2) poor performance status (ECOG PS >2); (3) no surgical removal of the primary tumor (n = 11); (4) inability to detect the lesions on US or contrast-enhanced ultrasound (CEUS) (n = 38); (5) inability to completely ablate the lesions because of proximity to major biliary structures or adherence to the gastrointestinal tract (n = 11); (6) inability for the patient to tolerate the pain during the procedure and termination of the treatment (n = 3); and (7) insufficient follow-up time (survival status = “intrahepatic progression-free survival” & survival time < 6 months; n = 25).
MWA procedure
A 2450-MHz microwave ablation system was used (KY2000; Nanjing Kangyou Biological Energy Co., Ltd., Nanjing, China) and included a microwave generator with a power output of 1–100 W, a flexible coaxial cable, and a cooled-shaft antenna (KY-2450-b; Nanjing Kangyou Biological Energy Co., Ltd., Nanjing, China). The dual channel antenna was 18 cm long and 1.9 mm in diameter (15 G), through which cold saline was circulated by a peristaltic pump to continuously cool the shaft. Pre-operative sedation was performed via an intramuscular injection of 50 mg of Dolantin (Chaohui; Shanghai Pharmaceuticals, Shanghai, China) 30 min before ablation, and another 50 mg of Dolantin was given on demand during ablation. Lidocaine (2%, Chaohui; Shanghai Pharmaceuticals, Shanghai, China) was used for local anesthesia before puncture. The antenna was inserted into the lesion under US guidance (LOGIQ E9; GE Healthcare, Milwaukee, WI). All ablations were performed according to the manufacturer’s protocol with the aim of creating an ablation zone that covered the lesion plus a safety margin of at least 5 mm. The number of antennas, ablative power, and effective ablative duration were designed based on the tumor volume and desired ablation zone. The MWA procedure used in our center is described as follows. For lesions 2.0 cm or less in diameter, a single antenna and a single insertion were used. For lesions 2.0–3.0 cm, two antennas and two insertions were used, and two antennas and multiple insertions were used for lesions > 3.0 cm. The distance between the two antennas was 0.5–1.0 cm. The energy output was adjusted between 40 and 60 W over 5–10 min to ensure that the ablation zone covered the lesion. The needle track was ablated at the end of the procedure to prevent bleeding and tumor seeding. CEUS was performed immediately following the procedure to determine whether the tumor was treated according to the protocol and was covered completely by the ablation zone. Because the immediate assessment after ablation would be affected by the inflamed hyperemia reaction around the ablation zone, CEUS was performed again the day after ablation to further clarify the “technical success” and the presence of adverse events. If the ablation zone was considered insufficient compared to the pre-MWA tumor size and/or margin, one or more additional sessions were performed immediately. The same radiologist (L. G. J., with 18 years of experience in liver ablation) performed all procedures.
Follow-up
CEUS, CECT (Aquilion One, Toshiba Medical System, Tokyo, Japan) or CEMRI (Optima MR360, GE Healthcare, Milwaukee, WI) were performed at one month post-MWA. CEUS was performed every 3 months thereafter, and CECT or CEMRI was performed every 3 months for half a year and every 6 months thereafter for follow-up.
We used the standardization of terminology and reporting criteria of image-guided tumor ablation that was recently updated by Ahmed et al. [Citation16]. “Technical success” was defined as the tumor being treated according to the protocol and covered completely by the ablation zone, which was assessed 30 min or one day post-MWA using CEUS. Complete ablation was achieved when the entire ablated area exhibited no enhancement on follow-up CECT or CEMRI at one month post-ablation. LTP was defined as the appearance of a hyper-enhanced nodule with washout located at the marginal area of the ablated zone after the tumor was documented as having achieved complete ablation. All other new contrast-enhancing focal lesions at other intrahepatic sites were defined as iHR [Citation16]. Intrahepatic disease progression included LTP and iHR. For the patients who developed intrahepatic disease progression, further ablation was offered. Surgical resection was performed for lesions that could not be completely ablated. For patients with intrahepatic disease progression who could not receive ablation or resection because of the tumor location or other reasons, systemic chemotherapy was given when possible. IhPFS was calculated from the date of MWA to the date of intrahepatic disease progression or the last date of follow-up (for patients who did not suffer intrahepatic disease progression). OS was defined as the time between the initial MWA procedure and the patient’s death from any cause or the most recent follow-up. Adverse events (AEs) were reported using a proposal by the Society of Interventional Radiology Standards of Practice Committee for complications and were classified into five levels according to severity [Citation17]. AEs were classified as follows: (1) mild AE: no therapy or nominal therapy; (2) moderate AE: moderate escalation of care that required substantial treatment (e.g., intervention under conscious sedation or blood product administration) and extremely prolonged outpatient observation; (3) severe AE: marked escalation of care with hospital admission or prolongation of existing hospital admission for >24 h, inpatient transfer from regular floor/telemetry to intensive care unit, or complex intervention performed requiring general anesthesia; (4) life-threatening or disabling event: cardiopulmonary arrest, shock, organ failure, unanticipated dialysis, paralysis, or loss of limb or organ; and (5) patient death [Citation17].
The assessment of ablation margins used in this study was originally described by Wang et al. [Citation18], and the same method was used in other studies [Citation19–21]. Ablation margins were measured on a picture archiving and communication system (Ruike PACS; Ruike Healthcare, Shanghai, China) using pre- and one-month post-ablation CECT scans and anatomical landmarks. Anatomical landmarks around the tumor were chosen, and the distances from the edge of the tumor and ablation defect to the landmarks were measured. For each landmark, the pre-ablation distance was subtracted from the post-ablation distance to render the margin at that site. The smallest value was considered the minimal margin. The margin size was classified as <5 mm, 5–10 mm, and >10 mm [Citation19]. Two radiologists (S. Q. and R. C., with 4 and 6 years of experience in liver radiographic imaging interpretation, respectively) performed these assessments in consensus (independently obtained, then reconciled), and a radiologist with 9 years of experience in liver radiographic imaging interpretation (Y. C.) resolved any differences in the evaluations between the other radiologists. None of the radiologists were familiar with the treatment and other imaging studies.
Clinicopathological characteristics of patients
All eligible patients underwent curative or palliative surgery. Palliative surgery (non-R0 resection) was performed for patients whose tumors could not be completely resected to reduce tumor burden and relieve symptoms. The pathology of primary tumors from surgical resection was assessed as a predictor of ihPFS. Immunohistochemical staining for altered proteins (MLH1, MSH2, MSH6, and PMS2) was used to screen patients and tumors for microsatellite instability (MSI) or deficiency in mismatch repair (MMR). The negative expression of one or more proteins was defined as deficient MMR (dMMR) or microsatellite instability-high (MSI-H). Otherwise, it was defined as proficient MMR (pMMR), microsatellite instability-low (MSI-L) or microsatellite stable (MSS) [Citation22]. NRAS, KRAS and BRAF gene status was determined from DNA extracted from the primary tumor. A routine polymerase chain reaction-based primer extension assay was performed to screen for mutations in KRAS exons 2, 3, and 4, NRAS exons 2, 3, and 4 and BRAF V600E mutation in patients treated in the most recent years of the study period [Citation23,Citation24]. Mutations in the various codons of KRAS and NRAS were reported and analyzed together as RAS mutations [Citation24].
Based on the general international classification method, synchronous liver metastases referred to liver metastases that were discovered at the time or before primary CRC and definitively diagnosed, and metachronous liver metastases referred to liver metastases that were discovered after radical excision of the primary CRC [Citation25].
Patients with advanced CRC, CRLMs larger than 3 cm, and >5 CRLMs received chemotherapy before MWA to reduce tumor burden. The chemotherapy regimen was oxaliplatin-based (n = 98), including XELOX and mFOLFOX6; a FOLFIRI regimen (n = 22); and FOLFOXIRI (n = 30). Bevacizumab (n = 15) and cetuximab (n = 8) were additionally administered to 23 patients. The number of chemotherapy cycles ranged from 2 to 12. The response to pre-operative chemotherapy was evaluated by CECT/MRI after every four cycles of treatment according to the Response Evaluation Criteria in Solid Tumors (RECIST) [Citation26]. During chemotherapy, the need for ablation was reconsidered at a multidisciplinary meeting each time a response to chemotherapy was observed. The interval between chemotherapy and MWA was 1–14 days. Patients with a high clinical risk score for tumor recurrence (score >2) received chemotherapy after MWA to reduce recurrence. Eleven patients did not undergo chemotherapy after MWA because they were unwilling or their physical status was unsuitable for chemotherapy administration.
Statistical analysis
Continuous variables with a normal distribution are expressed as the mean value ± standard deviation (SD). Otherwise, the median (range) was used. Categorical variables are expressed as the number and percentage. Survival curves for OS and ihPFS were calculated using the Kaplan–Meier method. Prognostic predictors were identified using univariable and multivariable analyses and Cox proportional hazards regression models. All variables associated with LTP, iHR and ihPFS with p < 0.05 in the univariable analyses were subsequently entered into a Cox multivariable proportional hazards model to assess their independent influence. Receiver operating characteristic (ROC) curves and area under the curve (AUC) analyses were used to identify optimal cutoff values for continuous variables. Factors that were included in the final model were used to construct the nomogram and risk classification system. Validation of the nomogram was performed using the concordance index (C-index), calibration curves, and decision curve analysis (DCA). The C-index was used to reflect the predictive accuracy and discrimination ability of each factor and the nomogram. Calibration curves (100 bootstrap resamples) were generated to test the calibration of the nomogram, and DCA was performed to assess the clinical usefulness of the nomogram. The total points calculated by the nomogram were categorized into three risk groups to predict ihPFS following the cutoff points detected by the X-tile analysis. Kaplan–Meier curves and the log-rank test were used to illustrate and compare the ihPFS of patients in the different risk groups.
Statistical analyses were performed using SPSS 22.0 software (SPSS, Inc., Chicago, IL), MedCalc v18.2.1 (MedCalc Software, Mokdongseo-ro, Seoul, Korea) and R software version 4.1.0 (R Foundation for Statistical Computing, Vienna, Austria). A value of p < 0.05 was considered statistically significant.
Results
A total of 314 patients (224 men and 90 women; mean age, 57.1 ± 11.8 years; range, 23-86 years) were included and analyzed (). The clinicopathological characteristics of the patients are shown in . RAS and BRAF gene status were available in our records for 175/314 (55.7%) patients. A total of 645 lesions were treated (mean diameter, 16.6 ± 6.5 mm; range, 6.0–45.0 mm). The average number of CRLMs per patient treated was 2 (range, 1–8). The median follow-up period was 23.5 months (range, 6.0–74.2 months).
Table 1. Characteristics of the patients.
Outcome and complications after MWA treatment
Technical success and complete ablation were achieved in all patients included in this study. LTP was observed in 34/314 (10.8%) patients and 37/645 (5.7%) lesions at a median time of 8.0 months. IHR occurred in 162/314 patients (51.6%) at a median time of 17.1 months. LTP and iHR co-occurred in 17 (17/34, 50.0%) patients, iHR followed by LTP occurred in four (4/34, 11.8%) patients, and the reverse pattern was observed in five (5/34, 14.7%) patients. Eight (8/34, 23.5%) patients developed LTP alone during follow-up. We jointly investigated LTP and iHR as intrahepatic disease progression because the number of patients with LTP alone was relatively small, and most LTP coexisted with iHR in this study. The 1-, 2-, and 3-year cumulative ihPFS rates were 59.0%, 38.9%, and 30.8%, respectively (). The median ihPFS time after MWA was 14.1 months. Further ablation was offered for 98 patients who developed intrahepatic disease progression, and the median ihPFS time after re-ablation was 8.4 months. Surgical resection was performed for three patients. Sixty-two patients received chemotherapy alone because of extrahepatic metastasis (n = 59) or refusal of surgery and ablation (n = 3). Seven patients refused surgery, ablation, and chemotherapy due to personal economic reasons or physical factors. The 1-, 2-, and 3-year OS rates after MWA were 99.3%, 88.7%, and 74.4%, respectively (). The incidence of AEs was 5.7% (18/314), including mild AEs in 3.5% (11/314) of patients (biloma n = 4, asymptomatic circumhepatic effusion n = 3, slightly dilated intrahepatic bile duct n = 3, and thrombus in the sagittal portal vein n = 1), moderate AEs in 1.0% (3/314) of patients (symptomatic pleural effusion n = 2 and symptomatic circumhepatic effusion n = 1), severe AEs in 1.0% (3/314) of patients (liver abscess n = 3) and life-threatening or disabling events in 0.3% (1/314) of patients (gastrointestinal perforation n = 1). There were no deaths related to ablation.
Univariable and multivariable analyses
Four factors significantly (p < 0.05) influenced LTP in the univariate analysis (Supplementary Table 1): primary tumor location, maximal size of CRLM, CRLMs near vessels, and ablative margin. Two independent prognostic factors for LTP were identified in the multivariable analysis (p < 0.05) (Supplementary Table 2): maximal size of CRLM and ablative margin. The LTP rates for margin sizes of <5 mm, 5–10 mm and >10 mm were 29.4% (25 of 85), 4.6% (9 of 194) and 0, respectively (p < 0.001). Seven factors significantly (p < 0.05) influenced iHR in the univariate analysis (Supplementary Table 3): primary tumor lymph node status, history of liver metastasis resection pre-MWA, number of CRLMs, maximal size of CRLM, unilobar or bilobar CRLM, timing of CRLM and chemotherapy. Four independent prognostic factors for iHR were identified in the multivariable analysis (p < 0.05) (Supplementary Table 4): maximal size of CRLM, number of CRLMs, primary tumor lymph node status and chemotherapy. Seven factors significantly (p < 0.05) influenced ihPFS in the univariate analysis (): primary tumor lymph node status, history of liver metastasis resection pre-MWA, number of CRLMs, maximal size of CRLM, unilobar or bilobar CRLM, ablative margin and chemotherapy. Five independent prognostic factors for ihPFS were identified in the multivariable analysis (p < 0.05) (): maximal size of CRLM, number of CRLMs, ablative margin, primary tumor lymph node status and chemotherapy. The maximal size of CRLMs and number of CRLMs were entered into ROC-AUC analysis to determine the optimal cutoff value. The cutoffs with the best combined sensitivity and specificity were 16 mm for the size of CRLMs (AUC: 0.612; p < 0.001) and 2 for the number of CRLMs (AUC: 0.610; p < 0.001).
Table 2. Univariable analyses of factors associated with intrahepatic disease progression.
Table 3. Multivariable analyses of factors associated with intrahepatic disease progression.
Prognostic nomogram
Based on the results of the Cox proportional hazards regression model, nomograms were constructed using all of the prognostic factors for iHR (Supplementary Figure 1) and ihPFS (), respectively. The C-index of the nomogram for predicting iHR was 0.670 (CI: 0.649–0.691). The C-index of the nomogram for predicting ihPFS was 0.702 (CI: 0.681–0.723), which reflected the good discrimination ability of the model. The nomogram for predicting LTP were not made separately because the number of patients with LTP was relatively small and there were fewer influencing factors screened out.
Figure 3. Prognostic nomogram for predicting the ihPFS of patients with CRLMs undergoing MWA. To use the nomogram, draw a line straight upward to the points axis at the same vertical position for each variable. Sum the points achieved for each predictor and locate this sum on the total points axis. Draw a line straight down to the ihPFS axis to find the patient’s probability of 1-, 2-, and 3-year ihPFS at the same vertical position. According to the total score, the risk group to which the patient belongs may be obtained. ihPFS: intrahepatic progression-free survival; CRLMs: colorectal liver metastases: MWA: microwave ablation; N: primary tumor lymph node status.
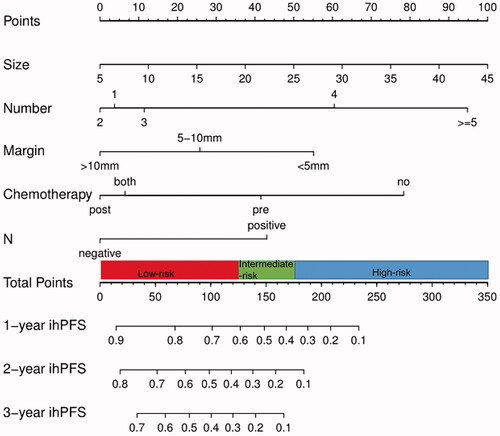
The performance of the nomogram for predicting ihPFS was measured using ROC curves, and the AUC values for the 1-, 2-, and 3-year ihPFS in the model from the observed data were 0.695, 0.764, and 0.782, respectively. Calibration curves were plotted [] and showed good agreement between the predicted survival estimates assessed by the nomogram and the direct observations at 1, 2, or 3 years after MWA. DCA exhibited great positive net benefits in the predictive model for most of the threshold probabilities at different time points, which indicated the favorable potential clinical effect of the predictive model [].
Figure 4. Calibration curves and decision curves of the nomogram. (a–c) Calibration curves showing the probability of 1-, 2-, and 3-year ihPFS between the nomogram prediction and the actual observation. Perfect prediction corresponds to a slope of 1 (diagonal 45-degree gray line). (d–f) Decision curves of the nomogram predicting ihPFS. The x-axis represents the threshold probabilities, and the y-axis measures the net benefit calculated by adding the true positives and subtracting the false positives. The horizontal line along the x-axis assumes that intrahepatic disease progression occurred in no patients, whereas the solid gray line assumes that all patients will have intrahepatic disease progression at a specific threshold probability. The dashed line represents the net benefit of using the nomogram. ihPFS: intrahepatic progression free survival.
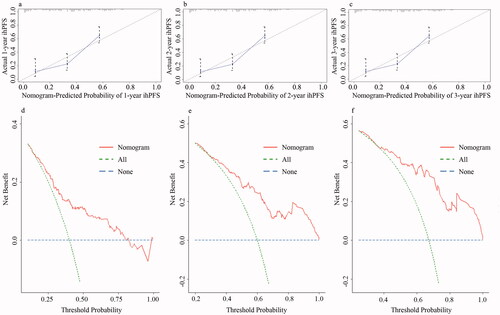
The total points calculated by the nomogram were categorized into three risk groups to predict ihPFS: low-risk (<126), intermediate-risk (126–176), and high-risk (>176) (). Kaplan–Meier curves showed that ihPFS in the different groups was accurately differentiated by the risk classification system (). The median ihPFS of patients in the low-, intermediate-, and high-risk groups was 36.3 months (95% CI: 21.4–51.1), 13.4 months (95% CI: 12.1–14.6), and 3.8 months (95% CI: 2.3–5.3), respectively.
Discussion
The progression of liver metastases predominantly determines mortality in CRC [Citation27]. During the last decade, thermal ablation was proposed as an alternative to surgical resection for CRLM patients with limited hepatic involvement [Citation3]. Prior studies described factors affecting LTP and the clinical risk score modified for the RFA of CRLMs [Citation20,Citation28,Citation29]. Independent risk factors for LTP included tumor size ≥ 2 cm, subcapsular tumor location and minimal ablative margin ≤ 5 mm [Citation28]. A modified clinical risk score including five criteria (node-positive primary, disease-free interval from primary to metastases > 12 months, number of hepatic tumors > 1, largest CRLM > 3 cm, and carcinoembryonic antigen (CEA) level > 30 ng/ml) was a patient stratification and prognostic tool after RFA for CRLMs [Citation20]. MWA is a relatively new thermal ablative modality that is used to provide local control of liver tumors. However, few predictive models are available for predicting intrahepatic disease progression (including LTP and iHR) after MWA for CRLMs. The present study developed a prognostic nomogram to predict ihPFS in patients with CRLMs after MWA. A risk classification that could satisfactorily separate the patients into three distinct prognostic groups and supplement the nomogram was also developed in the present study. The proposed nomogram is simple and useful for determining the individual likelihood of ihPFS after MWA in patients with CRLMs. The nomogram will help increase physicians’ understanding of the efficacy of ablation, facilitate effective physician-patient communication, and provide individualized predictions to clinicians and patients in the treatment decision-making process.
The number and size of CRLMs reflect the tumor burden and limitations in controlling CRLMs [Citation30]. The number of CRLMs, especially numbers >2, was identified as an independent prognostic factor for predicting ihPFS after MWA in the present study. Increasing numbers of tumors may represent an unfavorable tumor biology of micro-intrahepatic metastases [Citation10]. Moreover, MWA prolonged ihPFS for the treatment of small CRLMs compared to CRLMs larger than 16 mm (median ihPFS: 21.6 months versus 12.3 months, p < 0.001) in the present study. It is generally believed that a single-antenna approach achieves the complete ablation of tumors <2 cm and ensures sufficient ablation margins [Citation9]. Larger lesions may be treated by repositioning the antennae or the use of multiple antennae simultaneously to create larger zones of coagulative necrosis [Citation9]. However, it is often difficult to ensure sufficient ablation margins in all directions under the guidance of US alone. Adequate safety margins are a critical determinant of oncological outcomes after ablation [Citation2]. The consensus recommends that a minimum ablation margin of 1 cm is required in all directions or to the edge of the liver to maximize the likelihood of complete ablation [Citation2]. Some studies suggest that a minimal margin >5 mm is a critical factor for local tumor control [Citation18,Citation20,Citation21,Citation28]. Consistent with this finding, our study revealed that ablative margin ≥5 mm was a significant predictor of ihPFS for MWA. There was no significant difference in LTP (4.7% versus 0, p = 0.361) or ihPFS (median ihPFS: 17.1 months versus 34.5 months, p = 0.305) when comparing ablative margins between 5–10 mm and >10 mm. A larger ablative margin means better local tumor control, but it may be associated with a higher risk of complications. One study indicated no LTP for tumors ablated with margins >10 mm, but it indicated a relatively higher risk for patients with prior exposure to arterial chemotherapy, bevacizumab and preexisting biliary dilatation [Citation29]. Therefore, every effort should be made to create ablative margins at least larger than 5 mm around the tumor. Further improvement of local tumor control may be achieved with ablation zone biopsy negative for residual tumor when the ablative margin is within 5–10 mm [Citation21]. The need to assess the ablation zone and margins within the procedure is critical. Several studies describe the use of 3D software programs to assess the ablation zone and margins [Citation31,Citation32]. The ablation margin is related to tumor size and location. When the tumor is near important organs, such as the diaphragm and the gastrointestinal tract, it is difficult to ensure adequate ablation margins due to the unclear lesion display under the guidance of US and the fear of damaging adjacent structures [Citation2]. The use of fusion devices may be considered in these cases to help improve the monitoring of procedures and determine the appropriateness of the ablation margin [Citation33]. These patients should be advised about the possible need for repeat treatment and the necessity of appropriate follow-up to detect and treat early tumor regrowth and control the liver tumor load [Citation2].
The involvement of regional lymph node metastasis from CRC was a significant prognostic factor for outcomes in patients undergoing hepatic resection in a previous study [Citation34]. IhPFS after MWA was also positively associated with node negativity of the primary tumor in the present study. Adjuvant chemotherapy improves PFS [Citation2,Citation35,Citation36], and our study reported that patients without adjuvant chemotherapy exhibited substantially worse ihPFS (median ihPFS: 9.6 months versus 20.7 months, p < 0.001). Therefore, systematic chemotherapy after MWA should be routinely performed. Chemotherapy before ablation is often advocated in patients with advanced stages, heavy tumor burdens, and potential lesions that cannot be shown by imaging [Citation2]. Effective pre-RFA systemic therapy favorably affects recurrence patterns and long-term survival [Citation35]. However, some tumors may be difficult to visualize after chemotherapy because of the development of chemotherapy-induced liver injury and/or tumor shrinkage. Tumors that have disappeared on imaging remain detectable in pathology specimens, and recurrence is very likely on follow-up imaging as the tumors enlarge [Citation37].
Recent studies showed that RAS mutations, BRAF mutations and MSS CRC may be associated with worse prognoses [Citation22–24]. However, RAS gene status, BRAF gene status, and MMR were not associated with ihPFS in the present study, which may be due to the small number of patients. There is a minor discrepancy in biomarkers between the primary tumor and metastatic sites (concordance rates are 93.7% for KRAS and 99.4% for BRAF), and there is diversity in mutant alleles and variability in gene copy number, which may also contribute to the heterogeneity of clinical outcomes in cancer patients [Citation29].
The present study has several limitations. First, the possibility of bias inherent in retrospective studies could not be eliminated. Second, our observations were limited to a single-center experience. Third, a validation study using an external cohort is needed to confirm the usefulness of this nomogram. There was a lack of histopathological evaluation of the ablation zone and margins for viable tumor cells after ablation. Despite these limitations, our work provides valuable information for clinical practitioners and serves as a hypothesis-generating dataset for further clinical research.
In conclusion, this study provides outcome data indicating the potential of 3-year ihPFS for patients receiving US-guided percutaneous MWA for CRLM. The proposed nomogram was specifically designed for patients with liver-only metastases undergoing US-guided percutaneous MWA and may help precisely estimate their prognosis after ablation. Further studies are needed to validate the predictive ability of this nomogram.
Supplemental Material
Download PDF (315.3 KB)Acknowledgments
This study did not receive any funding, and the authors have no conflicts of interest to disclose.
Disclosure statement
No potential conflict of interest was reported by the author(s).
References
- Chakedis J, Squires MH, Beal EW, et al. Update on current problems in colorectal liver metastasis. Curr Probl Surg. 2017;54(11):554–602.
- Gillams A, Goldberg N, Ahmed M, et al. Thermal ablation of colorectal liver metastases: a position paper by an international panel of ablation experts, the Interventional Oncology Sans Frontières Meeting 2013. Eur Radiol. 2015;25(12):3438–3454.
- Van Cutsem E, Cervantes A, Adam R, et al. ESMO consensus guidelines for the management of patients with metastatic colorectal cancer. Ann Oncol. 2016;27(8):1386–1422.
- Benson AB, Venook AP, Al-Hawary MM, et al. Colon cancer, version 2.2021, NCCN clinical practice guidelines in oncology. J Natl Compr Canc Netw. 2021;19(3):329–359.
- Ruers T, Punt C, Van Coevorden F, Arbeitsgruppe Lebermetastasen und—tumoren in der Chirurgischen Arbeitsgemeinschaft Onkologie (ALM-CAO) and the National Cancer Research Institute Colorectal Clinical Study Group (NCRI CCSG), et al. Radiofrequency ablation combined with systemic treatment versus systemic treatment alone in patients with non-resectable colorectal liver metastases: a randomized EORTC intergroup phase II study (EORTC 40004). Ann Oncol. 2012;23(10):2619–2626.
- Ruers T, Van Coevorden F, Punt CJ, et al. Local treatment of unresectable colorectal liver metastases: results of a randomized phase II trial. J Natl Cancer Inst. 2017;109:djx015.
- Facciorusso A, Di Maso M, Muscatiello N. Microwave ablation versus radiofrequency ablation for the treatment of hepatocellular carcinoma: a systematic review and meta-analysis. Int J Hyperthermia. 2016;32(3):339–344.
- Glassberg MB, Ghosh S, Clymer JW, et al. Microwave ablation compared with radiofrequency ablation for treatment of hepatocellular carcinoma and liver metastases: a systematic review and meta-analysis. Onco Targets Ther. 2019;12:6407–6438.
- Groeschl RT, Wong RK, Quebbeman EJ, et al. Recurrence after microwave ablation of liver malignancies: a single institution experience. HPB (Oxford). 2013;15(5):365–371.
- Liu W, Wang K, Han Y, et al. Nomogram predicted disease free survival for colorectal liver metastasis patients with preoperative chemotherapy followed by hepatic resection. Eur J Surg Oncol. 2019;45(11):2070–2077.
- Higuchi A, Aoyama T, Kazama K, et al. Beppu's nomogram score is an independent prognostic factor for colorectal liver metastasis receiving perioperative chemotherapy and/or targeted therapy. In Vivo. 2019;33(4):1301–1306.
- Sjoquist KM, Renfro LA, Simes RJ, The Fondation Aide et Recherche en Cancerologie Digestive Group (ARCAD), et al. Personalizing survival predictions in advanced colorectal cancer: the ARCAD nomogram project. J Natl Cancer Inst. 2018;110(6):638–648.
- Reddy SK, Kattan MW, Yu C, et al. Evaluation of peri-operative chemotherapy using a prognostic nomogram for survival after resection of colorectal liver metastases. HPB (Oxford). 2009;11(7):592–599.
- Kattan MW, Gonen M, Jarnagin WR, et al. A nomogram for predicting disease-specific survival after hepatic resection for metastatic colorectal cancer. Ann Surg. 2008;247(2):282–287.
- Imai K, Allard MA, Castro BC, et al. Nomogram for prediction of prognosis in patients with initially unresectable colorectal liver metastases. Br J Surg. 2016;103(5):590–599.
- Ahmed M, Solbiati L, Brace CL, Standard of Practice Committee of the Cardiovascular and Interventional Radiological Society of Europe, et al. Image-guided tumor ablation: standardization of terminology and reporting criteria-a 10-year update. Radiology. 2014;273(1):241–260.
- Khalilzadeh O, Baerlocher MO, Shyn PB, et al. Proposal of a new adverse event classification by the society of interventional radiology standards of practice committee. J Vasc Interv Radiol. 2017;28(10):1432–1437.
- Wang X, Sofocleous CT, Erinjeri JP, et al. Margin size is an independent predictor of local tumor progression after ablation of Colon cancer liver metastases. Cardiovasc Intervent Radiol. 2013;36(1):166–175.
- Shady W, Petre EN, Do KG, et al. Percutaneous microwave versus radiofrequency ablation of colorectal liver metastases: ablation with clear margins (A0) provides the best local tumor control. J Vasc Interv Radiol. 2018;29(2):268–275.
- Shady W, Petre EN, Gonen M, et al. Percutaneous radiofrequency ablation of colorectal cancer liver metastases: factors affecting outcomes – a 10-year experience at a single center. Radiology. 2016;278(2):601–611.
- Sotirchos VS, Petrovic LM, Gonen M, et al. Colorectal cancer liver metastases: biopsy of the ablation zone and margins can be used to predict oncologic outcome. Radiology. 2016;280(3):949–959.
- De' AG, Bottarelli L, Azzoni C, et al. Microsatellite instability in colorectal cancer. Acta Biomed. 2018;89:97–101.
- Gagniere J, Dupre A, Gholami SS, et al. Is hepatectomy justified for BRAF mutant colorectal liver metastases?: a multi-institutional analysis of 1497 patients. Ann Surg. 2020;271(1):147–154.
- Brudvik KW, Jones RP, Giuliante F, et al. RAS mutation clinical risk score to predict survival after resection of colorectal liver metastases. Ann Surg. 2019;269(1):120–126.
- Xu J, Fan J, Qin X, China CRLM Guideline Group, et al. Chinese guidelines for the diagnosis and comprehensive treatment of colorectal liver metastases (version 2018). J Cancer Res Clin Oncol. 2019;145(3):725–736.
- Eisenhauer EA, Therasse P, Bogaerts J, et al. New response evaluation criteria in solid tumours: revised RECIST guideline (version 1.1). Eur J Cancer. 2009;45(2):228–247.
- Fowler KJ, Linehan DC, Menias CO. Colorectal liver metastases: state of the art imaging. Ann Surg Oncol. 2013;20(4):1185–1193.
- Han K, Kim JH, Yang SG, et al. A single-center retrospective analysis of periprocedural variables affecting local tumor progression after radiofrequency ablation of colorectal cancer liver metastases. Radiology. 2021;298(1):212–218.
- Kurilova I, Bendet A, Petre EN, et al. Factors associated with local tumor control and complications after thermal ablation of colorectal cancer liver metastases: a 15-year retrospective cohort study. Clin Colorectal Cancer. 2021;20(2):e82–e95.
- Stang A, Oldhafer KJ, Weilert H, et al. Selection criteria for radiofrequency ablation for colorectal liver metastases in the era of effective systemic therapy: a clinical score based proposal. Bmc Cancer. 2014;14(1):500.
- Kaye EA, Cornelis FH, Petre EN, et al. Volumetric 3D assessment of ablation zones after thermal ablation of colorectal liver metastases to improve prediction of local tumor progression. Eur Radiol. 2019;29(5):2698–2705.
- Laimer G, Jaschke N, Schullian P, et al. Volumetric assessment of the periablational safety margin after thermal ablation of colorectal liver metastases. Eur Radiol. 2021;31(9):6489–6499.
- Lee DH, Lee JM. Recent advances in the image-guided tumor ablation of liver malignancies: radiofrequency ablation with multiple electrodes, real-time multimodality fusion imaging, and new energy sources. Korean J Radiol. 2018;19(4):545–559.
- Suzuki H, Fujii T, Asao T, et al. Extracapsular lymph node involvement is associated with colorectal liver metastases and impact outcome after hepatectomy for colorectal metastases. World J Surg. 2014;38(8):2079–2088.
- Stang A, Donati M, Weilert H, et al. Impact of systemic therapy and recurrence pattern on survival outcome after radiofrequency ablation for colorectal liver metastases. J Cancer. 2016;7(14):1939–1949.
- Brandi G, De Lorenzo S, Nannini M, et al. Adjuvant chemotherapy for resected colorectal cancer metastases: literature review and meta-analysis. World J Gastroenterol. 2016;22(2):519–533.
- Khan K, Wale A, Brown G, et al. Colorectal cancer with liver metastases: neoadjuvant chemotherapy, surgical resection first or palliation alone? World J Gastroenterol. 2014;20(35):12391–12406.