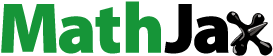
Abstract
Background
The novel separable clustered electrode system with two adjustable active tips (ICAEs) and a fine multipurpose needle (MPN) for in situ temperature monitoring and adjuvant agent injection was developed and validated in an animal study. The purpose of this study was to evaluate the technical efficacy and complication of the novel electrode system for patients who have small HCC.
Methods
In this prospective, single-center clinical trial, ten participants with 14 small (≤ 2 cm, BCLC 0-A) HCCs referred for RFA were enrolled. A novel electrode system consisting of two ICAEs and one MPN with a thermometer and side holes was used for RFA. The RF energy was delivered using a multichannel RF system combining bipolar and switching monopolar modes. Technical success, efficacy, and complications were evaluated on immediate and one-month follow-up CT.
Results
Technical success was achieved in 92.9% (13/14) of tumors. One participant withdrew consent after RFA, and technical efficacy was achieved in 91.7% (11/12) of tumors. None showed thermal injury to nontarget organs. All patients were discharged the day after RFA without major complications. The active electrode lengths were adjusted in 60% (6/10) of patients during the procedure to tailor the ablation zone (83.3%, n = 5) or treat two tumors with different sizes (16.7%, n = 1). MPN was capable of continuous temperature monitoring during all ablations (100%, 14/14).
Conclusions
RFA using a novel electrode system showed acceptable technical efficacy and safety in patients with small HCCs. Further comparative studies are needed for the investigation of the system’s potential benefits compared to conventional electrodes.
Introduction
Radiofrequency ablation (RFA) shows comparable overall survival to hepatic resection in patients with small hepatocellular carcinoma (HCC) less than 3 cm in diameter. Therefore, percutaneous ablation is widely recommended as one of the best treatment options for early- or very-early-stage HCC (Barcelona Clinic Liver Cancer stage 0 or A) according to major international guidelines, including the Korean Liver Cancer Association, National Cancer Center practice guideline v 2022 [Citation1–5]. Compared to surgery, RFA provides several advantages like shorter recovery time and fewer complications but is known to be inferior in local tumor control [Citation1,Citation6,Citation7]. Tumor location, tumor size, and the distance between the target tumor and large blood vessels are independent prognostic factors for local tumor control after RFA [Citation8,Citation9]. Besides, creating a sufficient ablative margin around the index tumor is critical for lowering local tumor progression [Citation10–12]. As RF electrodes usually can create an ablation sphere with a diameter range of 3 to 4 cm, the highest ablation rate could be achieved for HCCs with a diameter ≤ 3 cm [Citation13,Citation14].
Recently, various advances in RF systems and RF electrodes have been made to achieve more effective ablation based on improving energy distribution and depth of energy deposition [Citation15–18]. Moreover, no-touch techniques, anatomical ablation techniques, and combination therapies with other locoregional treatments have been developed for better outcomes [Citation18–23]. On the other hand, there has always been a tradeoff between large ablation zone and complication rates. Although the creation of a large ablation zone is advantageous for improving local tumor control, growing concerns have been raised about unnecessary thermal injuries related to the creation of larger ablation zones [Citation24]. The subcapsular location of the tumor is a risk factor for collateral thermal injury [Citation24]. Given the same tumor volume, the irregular shape of the tumor requires a larger volume of the ablation zone. Therefore, for treating irregularly shaped tumors in subcapsular locations abutting the bowels, gallbladder, heart, or diaphragm, operators should be more careful to avoid unnecessary thermal injury to adjacent vital organs.
To create tailored ablation zones according to nonspherical tumor shapes and to avoid unnecessary thermal damage, we surmised that separable adjustable electrodes, including a tissue temperature monitoring needle, might be valuable. Additionally, there is some evidence showing that a combination of adjuvant agent injections, such as saline or ethanol, during RFA may benefit treatment efficacy [Citation15,Citation23]. Therefore, we developed a novel separable clustered RF electrode system capable of temperature monitoring and adjuvant agent injection. The electrode system has recently demonstrated the ability to create tailored ablation zones with reduced nontarget thermal injury in animal studies [Citation25]. However, the feasibility of this method in human patients has not yet been established. This study aimed to evaluate the clinical feasibility of RFA using a novel separable clustered RF electrode system capable of active tip length adjustment, intraprocedural temperature monitoring, and adjuvant agent injection for treating HCC.
Materials and methods
This prospective, single-center, preliminary clinical trial was approved by the Institutional Review Board of Seoul National University Hospital (IRB No. 2112-092-1285). All participants signed written informed consent for enrollment in the study.
Study participants
From May 2022 to June 2022, we recruited study participants who met the following eligibility criteria: 1) age 20 to 85 years, 2) Child-Pugh class A or B7, and 3) small HCC (≤2 cm) on computed tomography (CT) or magnetic resonance imaging (MRI) examination within 60 days leading to referral for percutaneous RFA. The exclusion criteria were as follows: 1) tumor number >3 or tumor size >2 cm, 2) diffuse type HCC, 3) presence of macrovascular invasion or extrahepatic metastasis, 4) HCC abutting a large (≥5 mm) vessel or bile duct with <5 mm proximity, 5) bleeding tendency (platelet count <50,000/mm3 or prothrombin time international normalized ratio [PT INR] prolongation >50%). HCC was diagnosed based on the Korean guidelines for HCC management [Citation19]. Our criteria for the feasibility of a novel electrode system were achieving successful RF energy transmission and temperature monitoring in over 95% of cases. The formula for sample size calculation for a single proportion was used to estimate the minimum required sample size. The expected minimum sample size was calculated by commercially available statistical software (MedCalc Statistical Software Version 20.217, MedCalc Software, bv, Ostend, Belgium) as 7, with type I and II error levels of 0.05 and 0.20. Considering dropouts during the study, we finally planned to enroll 10 participants. The patient enrollment was terminated after reaching the study population target (N = 10).
Preprocedural considerations
The baseline information of patients and tumors were recorded by one radiologist (S.W.K. with 7 years of experience) after a thorough review of the electronic medical records of study participants and preprocedural imaging studies (CT or MRI). The patient’s age, sex, risk factors for HCC (e.g., chronic hepatitis B, alcoholic liver cirrhosis), previous locoregional or surgical treatment history (e.g., transarterial chemoembolization, RFA), laboratory test data including liver function tests (serum aspartate aminotransferase, alanine aminotransferase, albumin, total bilirubin), platelet counts, PT INR, and serum alpha-fetoprotein levels were recorded. Tumor size, location, and depth were recorded and evaluated based on preprocedural imaging. Tumor depth was categorized as either subcapsular (tumor extends to the parenchyma within 10 mm beneath the hepatic capsule) or deep (remaining tumors). In subcapsular tumors, the nearest adjacent nontarget organs (e.g., gallbladder, colon, stomach) were recorded.
RFA equipment
A specially designed separable clustered electrode (Octopus MP electrode; STARmed, Goyang, Korea) including two 17-gauge internally cooled electrodes with adjustable active tips at 5-mm intervals (2.0, 2.5, and 3.0 cm) (ICAE) and one 19-gauge multipurpose needle (MPN) were used for all RFA procedures. The shaft lengths of both ICAE and MPN are 15 cm. The MPN has four side holes (⌀, 0.3 mm) and a thermometer, enabling adjuvant agent (such as saline or ethanol) injection and intraprocedural temperature monitoring (). Although the MPN cannot deliver RF energy, ‘hot withdrawal’ for tract ablation is possible.
Figure 1. Octopus MP electrode system.
A. Two 17-gauge internally cooled electrodes with adjustable active tip lengths at 5-mm intervals (ICAE) (electrodes with longer needle shafts) and one 19-gauge multipurpose needle (MPN) capable of temperature monitoring and adjuvant agent injection (needle with shorter shaft). B. Adjustment of the active tip length in an ICAE. C. The MPN tip with four 0.3-mm-sized side holes (arrowheads a and b) and a thermometer (arrowhead c). Below is the schematic diagram of cross sections at each corresponding arrowhead level.
T: thermometer.
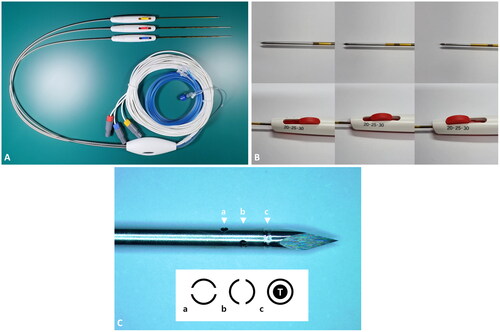
The multichannel RF system was used to deliver RF energy in bipolar or switching monopolar mode using the two ICAEs. A peristaltic pump (VIVA Pump; STARmed) was used for the infusion of an adjuvant agent at a constant rate through the MPN side holes. Technical parameters such as ablation time, electrode temperature, power output, electrical impedance, currents applied, and total energy deposition were monitored continuously and recorded using monitoring software (VIVA Monitor Software V 1.0; STARmed).
RFA procedure
One radiologist (J.M.L. with 22 years of experience in percutaneous RFA) performed all RFA procedures with assistance from one radiology fellow or resident. All procedures were done on an inpatient basis under conscious sedation. Preprocedural CT or MRI studies were reviewed before each procedure. Real-time US-CT/MR fusion imaging was used for the localization of the target lesion. US visibility and technical feasibility of each tumor were graded by an operator on a four-point scale [Citation26].
For all tumors, no-touch RFA was initially attempted. Under US-CT/MR fusion guidance, the two ICAEs were inserted into the peritumoral area of the index tumor (usually 3–5 mm from the margin of the tumor) with an interelectrode distance of 2–3 cm, depending on the tumor size [Citation22]. However, if no safe route for no-touch RFA was identifiable, we converted the procedure to conventional tumor puncture RFA (21.4%, 3/14). The operator adjusted the length of the active tip (2.0–3.0 cm) and mode of energy delivery (bipolar or switching monopolar) according to the tumor size, location, and desired ablation zone geometry. The operator determined whether or not to infuse artificial ascites regarding tumor visibility, the presence or absence of a safe route for electrode insertion, and the risk of nontarget thermal injury [Citation27]. After the decision to infuse artificial ascites, a 6-Fr angiosheath was inserted through the abdominal wall into the peritoneal cavity under local anesthesia, and 5% dextrose in water solution at room temperature was infused through the angiosheath [Citation27]. The operator monitored echogenic bubbles during the procedure and terminated ablation after achieving a desirable ablative margin around the index tumor (generally 5–10 mm around the tumor margin).
MPN Utility
For every procedure, the tip of MPN was placed into the tissue between the margin of the tumor and the external margin of the expected ablation zone to monitor treatment temperature adequacy. Special care was taken to avoid direct puncture of the tumor for oncologic safety. In some cases, the MPN was used for the following two additional purposes: 1) when a more expansive ablation zone was needed at a specific location, MPN was inserted into the location and subsequent saline infusions were performed at a rate of 0.5–1.0 cc/s (adjuvant injection [AI]), and 2) when a tumor was in a subcapsular location (<10 mm from the hepatic capsule) and a nontarget organ was in contact with the capsule, the MPN was inserted into the subcapsular parenchyma for continuous temperature monitoring (subcapsular parenchyma temperature monitoring, SPTM). To avoid nontarget thermal injury, ablation was terminated when the tissue temperature measured from near the capsule abutting adjacent organs reached a threshold (60 °C). illustrates the schematics of MPN insertion strategies.
Figure 2. Schematic diagram of the multipurpose needle (MPN) strategy.
For the usual cases, the MPN is inserted into the tissue between margin of the tumor and the external margin of ablation zone in order to monitor adequacy of treatment temperature ①. For adjuvant injection (AI), the MPN is inserted into the parenchyma near the tumor margin where the operator plans to create a larger ablation zone ②. For subcapsular parenchyma temperature monitoring (SPTM), the MPN is inserted into the subcapsular parenchyma for continuous temperature monitoring to avoid thermal injury in nontarget organs ③. For all MPN insertions, special care was taken not to directly puncture the tumor for oncological safety purposes.
E: electrode.
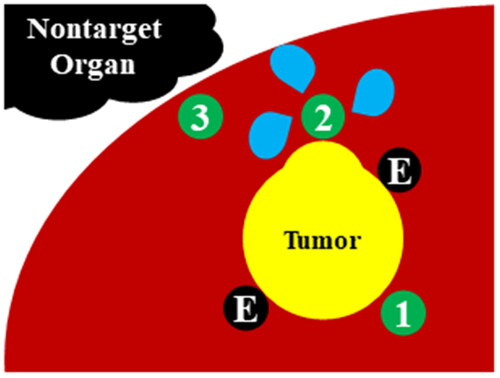
Evaluation of the procedure, follow-up, and outcome
Immediately after RFA completion, liver dynamic CT was performed to assess ablation size, possible complications, and technical success based on the reporting criteria suggested by the International Working Group on Image-guided Tumor Ablation [Citation28,Citation29]. Technical success of RFA was defined as complete coverage of the target tumor with sufficient ablation zone (> 3 mm) on immediate follow-up CT [Citation28,Citation29]. Any nodular enhancement at the ablation margin was considered to be a residual unabated tumor (RUT), and patients with RUT underwent additional ablation on the same day [Citation30]. The volume of the ablation zone was calculated using the longitudinal diameter along the electrode axis (Dl), the short diameter perpendicular to the electrode axis (Ds), and the vertical diameter (Dv) with the following formula: In addition, we also evaluated the presence of any sign of thermal injury to the adjacent organs near the ablation zone.
A contrast-enhanced CT or MRI was performed one month after RFA to detect local tumor progression, intrahepatic distant recurrence, or extrahepatic metastasis. Technical efficacy was defined as complete coverage of the target tumor by the ablation zone at one-month follow-up imaging [Citation28]. Any complications following tumor ablation procedures were classified as major or minor complications based on the Society of Interventional Radiology clinical practice guidelines [Citation28,Citation31].
The success of temperature monitoring was defined as the successful acquisition of continuous temperature data without interruption. The SPTM was considered successful if the temperature monitoring was successful and if evidence of nontarget organ injury was absent in immediate postprocedural CT. The AI was considered successful if sustained, significant (>10 °C) temperature decrease was detected by the MPN during the infusion of saline.
Results
Ten participants (male, N = 9; mean age, 65.1 ± 11.1 years) with 14 tumors (mean ± standard deviation of maximum tumor diameter, 1.24 ± 0.51 cm) were enrolled. Four patients had two tumors. Among 14 ablations, three procedures were converted from no-touch RFA to tumor puncture RFA due to lack of safe route. The baseline characteristics of participants, tumors, and ablation procedures are summarized in .
Table 1. Baseline characteristics of patients, tumors, and ablation procedures.
Technical success, technical efficacy, and complications
Overall technical success was achieved in 92.9% (13/14) of tumors according to immediate postprocedural CT. In the retrospective review of postprocedural images, one RUT in a patient was not detected at the time of immediate postprocedural CT. Thus, no additional ablation was done for the RUT during admission. All participants were discharged the day after the RFA. Three participants had minor complications: abdominal pain without fever (N = 2) and post-ablation syndrome with abdominal pain and fever (N = 1). All symptoms subsided within three days after the procedure.
One patient withdrew consent to study participation at the time of discharge, so follow-up data that occurred after discharge were excluded from the analysis. The remaining 9 participants with 12 tumors underwent follow-up CT after one month. Technical efficacy was achieved in 91.7% (11/12) of tumors. One viable tumor on one-month follow-up imaging, which is the above mentioned missed RUT, did not show significant change in the follow-up image. summarizes the outcomes of our study.
Table 2. Treatment outcomes.
Utility of ICAE and MPN
The active electrode lengths were adjusted in 60% (6/10) of participants during the procedure to either tailor them to the ablation zone (83.3%, 5/6) or treat two tumors of different sizes (maximum diameters, 1.4 cm and 0.5 cm) (16.7%, 1/6). The maximum diameters of the former five tumors requiring tailored ablation zones were 1.6 cm, 1.4 cm, 1.5 cm, 0.5 cm, and 1.9 cm. The longitudinal diameter of ablation zone (Dl), short diameter of ablation zone (Ds), vertical diameter of ablation zone (Dv) and volume of ablation zone (Vabl) for the five aforementioned index tumors were 5.0 cm-2.7 cm-3.3 cm-23.3 cm3, 7.2 cm-4.4 cm-5.2 cm-86.2 cm3, 4.4 cm-3.8 cm-4.3 cm-37.6 cm3, 5.4 cm-4.1 cm-2.2 cm-25.5 cm3, and 5.8 cm-3.8 cm-3.9 cm-45.0 cm3, respectively.
The MPN was capable of continuous temperature monitoring during all ablations (100.0%, 14/14). All cases in which SPTM and AI were attempted were successful (2/2 and 2/2, respectively). Artificial ascites were used in both cases of SPTM. No nontarget injury was observed in cases with SPTM in both immediate and one-month postprocedural imaging. In AI cases, a tip temperature decrease was observed during infusion, but impedance change was not noticeable (). summarizes the utility of ICAEs and the MPN in our study population. A successful ablation case using SPTM and a failed case with RUT are shown in , respectively.
Figure 3. Changes in current, impedance, and temperature before, during, and after adjuvant saline infusion in two cases with adjuvant injection (AI).
In both cases, the temperature (monitored by the MPN) dropped sharply at the beginning of the saline infusion. However, the saline infusion did not affect the impedance and current (monitored by the ICAEs) meaningfully in both cases.
MPN: multipurpose needle; ICAE: internally cooled electrode with adjustable active tip length.
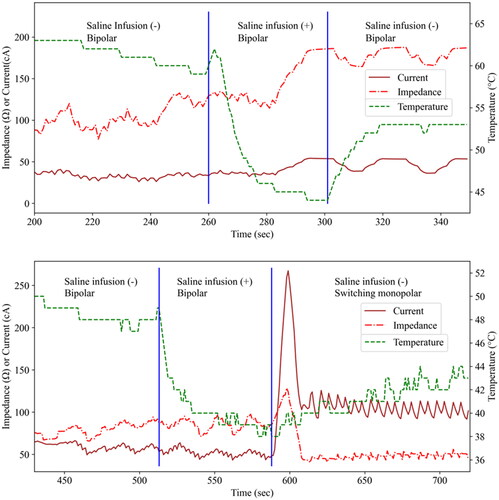
Figure 4. Successful ablation of a subcapsular tumor with nearby hepatic flexure of the colon in a 57-year-old male patient.
A 1.3-cm subcapsular tumor with hepatobiliary phase defect (arrowhead, upper left) and mild T2 hyperintensity (arrowhead, upper right) is close to the hepatic flexure of the colon. With US-MR fusion guidance, two ICAEs were inserted into the peritumoral parenchyma in a parallel manner with an interelectrode distance of 2.0 cm (middle left). An MPN was inserted into the subcapsular parenchyma (arrows, middle right) for SPTM. Note that the hepatic flexure of the colon lies close to the peritumoral liver capsule even after artificial ascites infusion (asterisk, middle right). In the immediate (lower row) and one-month follow-up CT (not shown), the index tumor was surrounded by an ablation zone with sufficient margin (arrowhead, lower left). Nontarget injuries were not observed in the colon (asterisk, lower right) and other nearby structures.
ICAE: internally cooled electrode with adjustable active tip length; MPN: multipurpose needle; SPTM: subcapsular parenchyma temperature monitoring; CT: computed tomography.
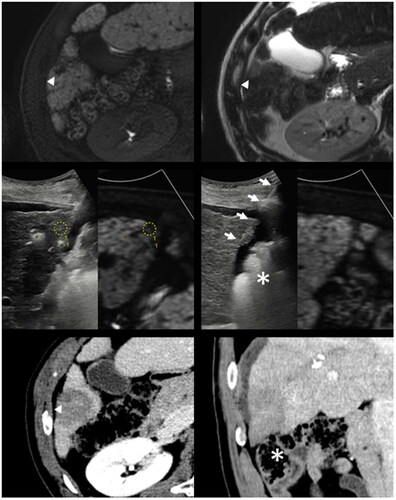
Figure 5. Technical Failure with a residual unablated tumor in a 69-year-old male patient.
A 0.4-cm-sized arterially enhancing nodule (arrowhead, upper left) with portal phase washout (arrowhead, upper right) was not visualized on B-mode ultrasound. RFA was performed under US-CT fusion guidance (arrow and arrowhead, middle left). In the immediate follow-up CT, nodular arterial phase enhancement (arrowhead, middle right) with portal phase washout (arrowhead, lower left) was noted but was mistakenly interpreted as a part of a treatment-related hyperemic rim. In the one-month follow-up CT, residual unablated tumor tissue is clearly delineated (arrowhead, lower right).
RFA: radiofrequency ablation; CT: computed tomography.
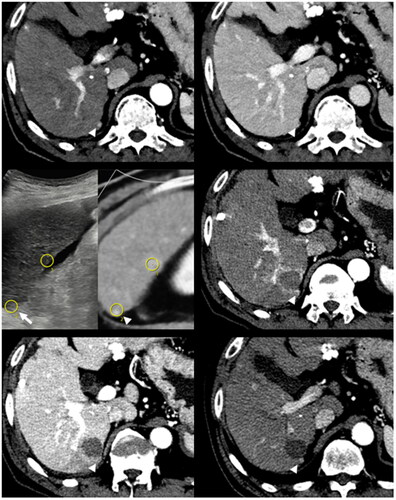
Table 3. Utility of the novel adjustable electrodes and multipurpose needles.
Discussion
For this clinical trial in patients with HCC, we evaluated the clinical feasibility of RFA using novel separable clustered electrodes composed of two electrodes with adjustable active tips and a fine electrode for in situ temperature monitoring and adjuvant agent injection. Overall technical success was achieved in 92.9% of tumors, and technical efficacy was achieved in 91.7% of tumors. All patients were discharged the day after the RFA without significant complications, and no patient showed thermal injury to adjacent organs near the ablation zone on immediate follow-up CT. With a novel electrode system, the active tip lengths of the two ICAEs were adjusted in 60.0% of patients during the procedure for tailoring the ablation zone or treating two tumors of different sizes. The MPN was capable of continuous temperature monitoring during all ablations. Based on our study results, the novel electrode system showed acceptable clinical feasibility for percutaneous RFA of small HCCs (<2 cm), especially in subcapsular locations. Furthermore, this system is expected to help avoid unnecessary thermal injury in patients with HCCs abutting the diaphragm, bowel, or gallbladder.
The novel electrodes were invented to tailor the ablation zone according to various tumor shapes and sizes and to decrease unnecessary thermal damage to adjacent organs, especially for peripherally located tumors, ultimately increasing flexibility and safety. Among the features of the novel electrodes, the adjustable active tips of the two ICAEs increased the operator’s flexibility to perform RFA. The active electrode lengths were adjusted in 60.0% of patients during the procedure either for tailoring the ablation zone (83.3%) or for treating two tumors of different sizes (16.7%). This feature was helpful for treating oval or irregularly shaped tumors having different diameters on each side and for treating subcapsular tumors surrounded by different thicknesses of liver parenchyma [Citation32]. Furthermore, it can be used for a multi-step gradual increment of the active tip length. Previous studies demonstrated that multi-step gradual increment of active tip length may provide higher efficiency of RF energy delivery by avoiding rapid increases in impedance and help to avoid intratumoral pressure elevation during the ablation procedure [Citation33]. On pathology, even small HCCs <2 cm show microvascular invasion in more than 30% of cases [Citation34]. Considering that intratumoral pressure elevation may have a detrimental effect on promoting intrahepatic metastases, especially in HCCs with microvascular invasion, the adjustability of active tip length may help decrease potential local tumor progression and intrahepatic distant recurrence in patients with small HCCs, especially, when used in combination with no-touch ablation techniques [Citation22,Citation35–38].
The MPN of the novel electrode system provided two advantages: 1) to monitor tissue temperature directly from the area at high risk of thermal injury and 2) to provide multiple holes for saline or ethanol injection. The MPN was capable of continuous temperature monitoring during all ablations. Nontarget injuries were not observed in cases with SPTM in both immediate and one-month postprocedural imaging, although confounding effects of successful artificial ascites injections should be considered with respect to the true protective effect of SPTM. Complications resulting from RFA include those related to imaging-guided electrode placement such as bleeding, infection, tract seeding, and nontarget thermal damage, such as of the biliary system or bowel [Citation39]. Several techniques have been proposed to prevent nontarget thermal damage to the gallbladder, diaphragm, or bowel, including artificial ascites, hydro dissection, and pneumoperitoneum [Citation18,Citation27,Citation39]. However, when severe adhesion is present in patients who had previous surgical treatments, direct tissue temperature measurements during the ablation procedure may provide additional benefits by avoiding nontarget thermal damage. Another indication of MPN is the injection of an adjuvant agent (such as saline or ethanol), and therefore, novel electrodes could be used for ethanol-assisted RFA. In this study, two cases in which AIs had been attempted were successful and showed tissue temperature drops following saline injection. Interestingly, the impedance was not decreased during the saline injection. This might be explained by the distribution of saline being mainly around the MPN rather than the other electrodes, which may fail to lower impedance. Because of the non-comparative design of the study, the positive effect of saline injection, such as increased ablation zone volume or improved patient outcome could not be evaluated. Further prospective comparative studies with larger numbers of patients are warranted to evaluate the clinical impact of the MPN of the novel electrode system.
Our study has several limitations. First, the small sample size and short follow-up duration after RFA are major limitations of our study. However, the primary goal of this study was to evaluate the clinical feasibility of RFA using a novel electrode system. Second, due to the non-comparative study design, we were unable to demonstrate improvement in the clinical outcomes of the new electrode system regarding oncologic safety and complications. Thus, a new comparative study is warranted. Third, as our study was performed by a single operator having considerable experience in RFA in a tertiary academic hospital, this might act as a bias to overestimate the technical feasibility. Although objective principles for judging the termination of the RFA procedure were implemented based on tissue temperature monitoring and virtual tumor markers of the fusion imaging system, some subjectivity was involved as the operator made the final decision. Additionally, the frequent use of artificial ascites (85.6%, 12/14) may have been a significant confounding factor in estimating the usefulness of MPNs in preventing nontarget thermal injury.
In conclusion, RFA using multipurpose separable clustered electrodes demonstrated clinical feasibility by providing acceptable technical efficacy and safety in patients with small HCCs. Further comparative studies with a larger population ideally designed as a multicenter study are warranted to determine the clinical benefit of this new electrode system.
Disclosure statement
No potential conflict of interest was reported by the author(s).
Data availability statement
The data that support the findings of this study are available on request from the corresponding author, Jeong Min Lee. The data are not publicly available due to the privacy of research participants.
Additional information
Funding
References
- Xu Q, Kobayashi S, Ye X, et al. Comparison of hepatic resection and radiofrequency ablation for small hepatocellular carcinoma: a meta-analysis of 16,103 patients. Sci Rep. 2014;4:7252. doi: 10.1038/srep07252.
- Galle PR, Forner A, Llovet JM, et al. European association for the study of the liver. EASL clinical practice guidelines: management of hepatocellular carcinoma. J Hepatol. 2018;69(1):182–236.
- Korean Liver Cancer Association (KLCA), National Cancer Center (NCC). Korean liver cancer association–national cancer center Korea practice guidelines for the management of hepatocellular carcinoma. Korean J Radiol. 2018;20:1042–1113.
- Reig M, Forner A, Rimola J, et al. BCLC strategy for prognosis prediction and treatment recommendation: the 2022 update. J Hepatol. 2022;76(3):681–693. doi: 10.1016/j.jhep.2021.11.018.
- Takayama T, Hasegawa K, Izumi N, et al. Surgery versus radiofrequency ablation for small hepatocellular carcinoma: a randomized controlled trial (SURF trial). Liver Cancer. 2022;11(3):209–218. doi: 10.1159/000521665.
- Lee MW, Kang D, Lim HK, et al. Updated 10-year outcomes of percutaneous radiofrequency ablation as first-line therapy for single hepatocellular carcinoma< 3 cm: emphasis on association of local tumor progression and overall survival. Eur Radiol. 2020;30(4):2391–2400. doi: 10.1007/s00330-019-06575-0.
- Lee DH, Kim JW, Lee JM, et al. Laparoscopic liver resection versus percutaneous radiofrequency ablation for small single nodular hepatocellular carcinoma: comparison of treatment outcomes. Liver Cancer. 2021;10(1):25–37. doi: 10.1159/000510909.
- Lee DH, Lee JM, Kang TW, et al. Clinical outcomes of radiofrequency ablation for early hypovascular HCC: a multicenter retrospective study. Radiology. 2018;286(1):338–349. doi: 10.1148/radiol.2017162452.
- Zhou Y, Yang Y, Zhou B, et al. Challenges facing percutaneous ablation in the treatment of hepatocellular carcinoma: extension of ablation criteria. J Hepatocell Carcinoma. 2021;8:625–644. doi: 10.2147/JHC.S298709.
- Kim KW, Lee JM, Klotz E, et al. Safety margin assessment after radiofrequency ablation of the liver using registration of preprocedure and postprocedure CT images. AJR Am J Roentgenol. 2011;196(5):W565–W572. doi: 10.2214/AJR.10.5122.
- Teng W, Liu KW, Lin CC, et al. Insufficient ablative margin determined by early computed tomography may predict the recurrence of hepatocellular carcinoma after radiofrequency ablation. Liver Cancer. 2015;4(1):26–38. doi: 10.1159/000343877.
- Wang X, Sofocleous CT, Erinjeri JP, et al. Margin size is an independent predictor of local tumor progression after ablation of Colon cancer liver metastases. Cardiovasc Intervent Radiol. 2013;36(1):166–175. doi: 10.1007/s00270-012-0377-1.
- Shiina S, Tateishi R, Arano T, et al. Radiofrequency ablation for hepatocellular carcinoma: 10-year outcome and prognostic factors. Am J Gastroenterol. 2012;107(4):569–577; quiz 578. doi: 10.1038/ajg.2011.425.
- Kim YS, Lim HK, Rhim H, et al. Ten-year outcomes of percutaneous radiofrequency ablation as first-line therapy of early hepatocellular carcinoma: analysis of prognostic factors. J Hepatol. 2013;58(1):89–97. doi: 10.1016/j.jhep.2012.09.020.
- Lee JM, Han JK, Kim SH, et al. Wet radio-frequency ablation using multiple electrodes: comparative study of bipolar versus monopolar modes in the bovine liver. Eur J Radiol. 2005;54(3):408–417. doi: 10.1016/j.ejrad.2004.06.004.
- Ahmed M, Brace CL, Lee FT, Jr., et al. Principles of and advances in percutaneous ablation. Radiology. 2011;258(2):351–369. doi: 10.1148/radiol.10081634.
- Lee J, Lee JM, Yoon JH, et al. Percutaneous radiofrequency ablation with multiple electrodes for medium-sized hepatocellular carcinomas. Korean J Radiol. 2012;13(1):34–43. doi: 10.3348/kjr.2012.13.1.34.
- Lee DH, Lee JM. Recent advances in the image-guided tumor ablation of liver malignancies: radiofrequency ablation with multiple electrodes, real-time multimodality fusion imaging, and new energy sources. Korean J Radiol. 2018;19(4):545–559. doi: 10.3348/kjr.2018.19.4.545.
- Hong K, Georgiades C. Radiofrequency ablation: mechanism of action and devices. J Vasc Interv Radiol. 2010;21(8 Suppl):S179–S186. doi: 10.1016/j.jvir.2010.04.008.
- Lu Z, Wen F, Guo Q, et al. Radiofrequency ablation plus chemoembolization versus radiofrequency ablation alone for hepatocellular carcinoma: a meta-analysis of randomized-controlled trials. Eur J Gastroenterol Hepatol. 2013;25(2):187–194. doi: 10.1097/MEG.0b013e32835a0a07.
- Chen QW, Ying HF, Gao S, et al. Radiofrequency ablation plus chemoembolization versus radiofrequency ablation alone for hepatocellular carcinoma: a systematic review and meta-analysis. Clin Res Hepatol Gastroenterol. 2016;40(3):309–314. doi: 10.1016/j.clinre.2015.07.008.
- Lee DH, Lee MW, Kim PN, et al. Outcome of no-touch radiofrequency ablation for small hepatocellular carcinoma: a multicenter clinical trial. Radiology. 2021;301(1):229–236. doi: 10.1148/radiol.2021210309.
- Lu DE, Cheng SW, Lin YS, et al. Combination of radiofrequency ablation and percutaneous ethanol injection versus radiofrequency ablation alone for hepatocellular carcinoma: a systematic review and meta-analysis. Ann Hepatol. 2022;27(5):100729. doi: 10.1016/j.aohep.2022.100729.
- Mulier S, Mulier P, Ni Y, et al. Complications of radiofrequency coagulation of liver tumours. Br J Surg. 2002;89(10):1206–1222. doi: 10.1046/j.1365-2168.2002.02168.x.
- Park S-J, Lee JM, Kim JH, et al. Comparison of ablation performance between octopus multipurpose electrode versus conventional octopus electrode. Korean J Radiol. 2023;24(2):86–94. doi: 10.3348/kjr.2022.0600.
- Ahn SJ, Lee JM, Lee DH, et al. Real-time US-CT/MR fusion imaging for percutaneous radiofrequency ablation of hepatocellular carcinoma. J Hepatol. 2017;66(2):347–354. doi: 10.1016/j.jhep.2016.09.003.
- Song I, Rhim H, Lim HK, et al. Percutaneous radiofrequency ablation of hepatocellular carcinoma abutting the diaphragm and gastrointestinal tracts with the use of artificial ascites: safety and technical efficacy in 143 patients. Eur Radiol. 2009;19(11):2630–2640. doi: 10.1007/s00330-009-1463-x.
- Ahmed M, Solbiati L, Brace CL, et al. Image-guided tumor ablation: standardization of terminology and reporting criteria—a 10-year update. Radiology. 2014;273(1):241–260. doi: 10.1148/radiol.14132958.
- Yoon JH, Lee JM, Klotz E, et al. Prediction of local tumor progression after radiofrequency ablation (RFA) of hepatocellular carcinoma by assessment of ablative margin using pre-RFA MRI and post-RFA CT registration. Korean J Radiol. 2018;19(6):1053–1065. doi: 10.3348/kjr.2018.19.6.1053.
- Shropshire EL, Chaudhry M, Miller CM, et al. LI-RADS treatment response algorithm: performance and diagnostic accuracy. Radiology. 2019;292(1):226–234. doi: 10.1148/radiol.2019182135.
- Sacks D, McClenny TE, Cardella JF, et al. Society of interventional radiology clinical practice guidelines. J Vasc Interv Radiol. 2003;14(9 Pt 2):S199–S202. doi: 10.1097/01.rvi.0000094584.83406.3e.
- Cha J, Kim YS, Rhim H, et al. Radiofrequency ablation using a new type of internally cooled electrode with an adjustable active tip: an experimental study in ex vivo bovine and in vivo porcine livers. Eur J Radiol. 2011;77(3):516–521. doi: 10.1016/j.ejrad.2009.09.011.
- Kotoh K, Morizono S, Kohjima M, et al. Evaluation of liver parenchymal pressure and portal endothelium damage during radio frequency ablation in an in vivo porcine model. Liver Int. 2005;25(6):1217–1223. doi: 10.1111/j.1478-3231.2005.01167.x.
- Kim KA, Kim MJ, Jeon HM, et al. Prediction of microvascular invasion of hepatocellular carcinoma: usefulness of peritumoral hypointensity seen on gadoxetate disodium‐enhanced hepatobiliary phase images. J Magn Reson Imaging. 2012;35(3):629–634. doi: 10.1002/jmri.22876.
- Hirooka M, Hiraoka A, Ochi H, et al. Prospective cohort trial to confirm the efficacy of no-touch radio frequency ablation. J Gastroenterol Hepatol. 2019;34(3):567–574. doi: 10.1111/jgh.14476.
- Kawamura Y, Ikeda K, Shindoh J, et al. No-touch ablation in hepatocellular carcinoma has the potential to prevent intrasubsegmental recurrence to the same degree as surgical resection. Hepatol Res. 2019;49(2):164–176. doi: 10.1111/hepr.13254.
- Park SJ, Cho EJ, Lee JH, et al. Switching monopolar no-touch radiofrequency ablation using octopus electrodes for small hepatocellular carcinoma: a randomized clinical trial. Liver Cancer. 2021;10(1):72–81. doi: 10.1159/000512338.
- Kim TH, Choi HI, Kim BR, et al. No-touch radiofrequency ablation of VX2 hepatic tumors in vivo in rabbits: a proof of concept study. Korean J Radiol. 2018;19(6):1099–1109. doi: 10.3348/kjr.2018.19.6.1099.
- Rhim H, Dodd GD, 3rd, Chintapalli KN, et al. Radiofrequency thermal ablation of abdominal tumors: lessons learned from complications. Radiographics. 2004;24(1):41–52. doi: 10.1148/rg.241025144.