Abstract
Purpose
High-intensity focused ultrasound (HIFU) represents an emerging noninvasive modality for tumor treatment. While biological responses and immunological change associated with incomplete ablation have not been thoroughly investigated. This study aims to evaluate the damage effect of HIFU incomplete ablation via establishing animal model and further explore its possible mechanism to inhibit tumor growth.
Methods
The rabbit VX2 breast cancer model was established and received HIFU treatment with complete ablation (100% tumor volume) and incomplete ablation (about 80% tumor volume) under real-time B-ultrasound monitoring. Histopathological alterations, dynamics of tumor cell apoptosis and proliferation, expression levels of VEGF, MMP-9, IL-2R, TGF-β1, HSP-70, IL-6, IL-8, and INF-γ, and the presence of circulating tumor cells (CTCs) were evaluated post-HIFU incomplete ablation.
Results
For HIFU 80% ablation group, there was an 85.85% reduction in tumor volume 21 days post-intervention. A marked increase in tumor cell apoptosis and a concomitant decrease in proliferation were observed. Notably, distant tumor metastasis rates, CTC counts, and expression levels of VEGF, MMP-9, IL-2R, TGF-β1, IL-6, and IL-8 were significantly reduced. In contrast, INF-γ and HSP-70 expressions were notably elevated, aligning with findings from the 100% ablation group.
Conclusions
HIFU incomplete ablation, with an 80% tumor ablation rate, induces substantial tumor damage, augments tumor cell apoptosis, and triggers an anti-tumor immune response, curtailing metastasis. These insights may underpin further investigations into the therapeutic implications of HIFU incomplete ablation.
1. Introduction
Breast cancer is a highly malignant cancer with the highest incidence and mortality rate among women globally [Citation1]. In 2020, there were approximately 2,261,419 diagnosed cases of invasive breast cancer in women, resulting in approximately 684,996 deaths, thereby ranking it as the leading cause of death from malignant cancer in women [Citation1,Citation2]. Currently, traditional surgical interventions remain the primary treatment modality for breast cancer. However, procedures such as radical mastectomy, modified radical mastectomy, and breast conservation are invasive and can result in various complications, thereby diminishing patients’ quality of life [Citation4–6]. As treatment paradigms shift and technology advances, minimally invasive and noninvasive treatment modalities have emerged as appealing alternatives for breast cancer, offering reduced trauma and lower risks of complications [Citation7,Citation8].
High-Intensity Focused Ultrasound (HIFU), often termed a ‘bloodless surgical knife’, is emerging as a promising noninvasive and non-radiative transcutaneous treatment modality for solid tumor therapy [Citation9–11]. Theoretically, HIFU focuses the high-frequency pressure ultrasound waves generated outside the body on in vivo targeted lesions. Ultrasonic energy can penetrate tissue and deposit energy to raise the temperature of the focused area to 60 ∼ 95 °C within a few seconds thereby inducing localized protein denaturation and coagulative necrosis of cancer cells [Citation12,Citation13]. Compared with traditional modified radical mastectomy, HIFU treatment is increasingly adopted in breast cancer management due to its noninvasive, real-time efficacy evaluation, conformal treatment and other characteristics [Citation14,Citation15]. Generally, HIFU treatment of breast cancer usually adopts ultra-wide ablation strictly to achieve more thorough treatment. In fact, when the tumor has no capsule, unclear boundary and infiltration and adhesion with surrounding normal tissues, or the tumor tissue volume is too large and the sound channel skin surface is broken, it is difficult to completely ablate the tumor tissue clearly. Given the limitations of safety and sound channel conditions, there exists a potential for incomplete ablation in HIFU therapy [Citation16,Citation17]. Therefore, it is crucial to investigate the impact of incomplete ablation on both the ablation area and the residual tissues in the surrounding region, as well as its implications for tumor prognosis and recurrence.
Furthermore, beyond the immediate coagulation necrosis of tumor cells caused by HIFU through direct hyperthermia and mechanical shearing, previous studies have confirmed that the local ablative techniques could cause the damaged tumor cells to release endogenous danger signals, enhance tumor infiltration of macrophages, and lead to HIFU-induced anti-tumor immunity. However, it is still unknown whether the anti-tumor immune response induced by incomplete ablation is exactly the same as that of complete ablation. It is worth exploring how the ablation junction and residual tumors stimulate the changes of host immune response.
Current experimental research on breast cancer treatment delves into the biological behavior and drug efficacy through animal models. While the mouse breast transplantation tumor model is commonly used for drug development and mechanisms, its small size makes it unsuitable for surgical or ablative treatments. In contrast, the VX2 tumor is a rabbit papilloma caused by Shope virus infection, characterized by squamous cell carcinoma. With abundant blood flow and rapid growth, it provides a convenient and efficient rabbit model for cancer studies. The simple carcinogenesis method leads to a high tumor formation rate, making the VX2 tumor model extensively utilized in breast cancer research. In this study, we established HIFU tumor ablation models with incomplete coverage in VX2 breast cancer rabbits. We then compared the efficacy of tumor inhibition, prognosis, and systemic anti-tumor immune response changes associated with complete and incomplete HIFU ablation treatments. The in-depth analysis of the prognosis of HIFU incomplete ablation may help to provide improved focused ultrasound treatment strategies, especially to provide incomplete ablation scheme guidance for tumors that cannot be completed ablation treatment at one time.
2. Materials and methods
2.1. Experimental animals and VX2 breast cancer model construction
Healthy female New Zealand rabbits, 3 ∼ 4 months old, weighing 2.0 ∼ 2.5 kg, were purchased from the Experimental Animal Center of Chongqing Medical University. Tumor inoculations were performed using the VX2 tumor strain (Institute of Ultrasound Medicine, Chongqing Medical University). Briefly, the tumor tissue with good growth and no necrosis was taken out from tumor-bearing rabbit and cut into 1mm3 tumor pieces, and then embedded in the second pair of mammary glands on the left side of the rabbit. The tumor volume was measured after surgery, and the formula for calculating the tumor volume was: V = (long diameter × short diameter2)/2. After 11 ∼ 14 days, the VX2 breast cancer model was established successfully. Our study was approved by the Animal Ethics Committee of Chongqing Medical University.
2.2. HIFU ablation treatment
The VX2 breast cancer rabbits were randomly divided into 3 groups: the complete group received 100% tumor volume ablation (100% group, n = 25), while the incomplete group received 80% (±5%) tumor volume ablation (80% group, n = 25), the control group received HIFU sham sonication (rabbits were put on the HIFU machine without the focused ultrasound generated, n = 20).The tumor localization and real-time monitoring of ablation range during the HIFU treatment were performed using the HIFU treatment system (JC200, developed by Chongqing Haifu Medical Technology Co., Ltd.) and B-ultrasound [Citation18,Citation19]. The ultrasound power was set at 150 W/cm2, with a scanning speed of 3 mm/s and a frequency of 1.03 MHz. A diameter of 158 mm transducer was utilized to ablate the target tumor tissue in a sequential manner, following the ‘point-line-plane-volume’ approach: utilizing the target area center as the focal point, whereby under ultrasound guidance, focal displacement was orchestrated to effect linear ablation. Subsequent expansion led to the establishment of planar ablation, culminating in the ultimate achievement of comprehensive three-dimensional intratumoral volumetric ablation of the entire target neoplastic tissue. The tumor area and ablation area were consistently delineated by the same individual. Subsequently, the long axis and short axis of the ablation area were measured using the HifuJupiterF software integrated in the HIFU treatment system, allowing for an approximate calculation of the ablation area’s volume. The tumor ablation rate for each group was calculated using the formula: tumor ablation rate = (approximate volume of hyperechoic target area after sonication/approximate volume of tumor before sonication). Based on the different ablation ranges, corresponding treatment plans were devised. The total ultrasound dose per cubic centimeter was the same for each tumor model, and only the extent of the ablated tumor differed. The weight and tumor size of the rabbits were recorded prior to treatment and on the 0, 3rd, 5th, 7th, 11th, 14th and 21st day after HIFU treatment, and the tumor inhibition rate was calculated by the formula: TIR =(tumor volume before treatment - tumor volume after treatment)/tumor volume before treatment × 100%.
2.3. Observation of tumor damage area by TTC staining
One day after ablation, two rabbits from each group were randomly selected and euthanized. The tumors were carefully excised, washed with PBS, and longitudinally sectioned through the middle along the direction of HIFU treatment. A portion of the tumor tissue was immersed in 2% 2, 3, 5 -Triphenyl -2H-tetrazolium chloride (TTC, Biofroxx GMBH) solution, and incubated in darkness at 37 °C for 30-40 min. The albuginea on the periphery of the tumor tissue was gently removed using a blade, revealing the white coagulated necrotic area in the center, which indicated the extent of ablation. The dimensions (length, width, and height) of the coagulation necrosis area were measured, and the actual ablation volume was calculated using the appropriate formula to determine if the tumor ablation range met the experimental requirements.
2.4. Observation of histopathological structural changes by H&E staining
The tumors were harvested on the 1st day and 21st day after HIFU ablation and fixed in 4% paraformaldehyde for 24 h and then dehydrated, transparent, waxed, embedded and sliced. The sections were stained with hematoxylin and eosin to observe the pathological structure changes in the center and periphery of the tumor after incomplete ablation.
2.5. Tumor apoptotic cells dynamic detection
The tumor apoptotic cells were detected using a terminal deoxynucleotidyl transferase dUTP nick-end labeling (TUNEL) assay on the 1st, 3rd, 7th, 14th, and 21st day. The boundary area between the ablation site and the surrounding tissue were taken and fixed with 4% paraformaldehyde to prepare tissue sections. The section was digested with proteinase K and incubated with a TUNEL detection solution according to the manufac turer’s instructions using a one-step TUNEL cell apoptosis detection kit (C1088, Beyotime, Shanghai, China). Then, the tumor nucleus were stained by DAPI dye. The distribution of apoptotic cells was observed under a fluorescence microscope (Blue sky C1086) and the apoptotic cells were marked with bright green fluorescence. Five areas of positive expression in random high-power (×100) fields were selected from each section, and the fluorescence intensity of green fluorescence was quantitatively analyzed by Image J software (National Institute of Health, USA), then the Tumor cell apoptisis rate was calculated by the formula: Tumor cell apoptisis rate = green fluorescence intensity/blue fluorescence intensity.
2.6. Analysis of cell proliferation and angiogenesis factor by immunohistochemical
The expression of proliferating cell nuclear antigen (PCNA) and CD31 angiogenesis factor was detected by immunohistochemical. Briefly, tumor tissues were collected on the 1st, 3rd, 7th, 14th, and 21st day after HIFU ablation, and fixed in 4% paraformaldehyde to prepare tissue sections. Sections were routinely dewaxed, incubated at room temperature with 3% H2O2 and 5% BSA blocking solution. Then antibody working solution of PCNA(AF1303, Beyotime Biological, Shanghai, China) and CD31 (AF1642, Beyotime Biological, Shanghai, China) were added dropwise and incubated. The brownish-yellow or tan-colored nuclei granules in the visual field were considered as the positive expression. Five visual fields were randomly selected from each group, and the average optical density (AOD) was analyzed by Image J software.
2.7. Detection of multiple cytokines expression by ELISA
The blood samples were collected from rabbit auricular veins on the 0, 3rd, 7th, 14th, and 28th day after HIFU ablation and then freeze centrifuged at 3600 rpm for 15 min. The supernatant was separated and used for the quantifcation of the multiple cytokines IL-2R, TGF-β1,vascular endothelial growth factor (VEGF) and Matrix Metalloprotein (MMP-9), HSP-70, IL-6, IL-8, and INF-γ using the corresponding rabbit ELISA kits (JingMei BioTech, Jiangsu, China) in accordance with the manufacturer’s instructions. The reaction product was measured at the absorbance at 450 nm to detect various cytokines concentration using microplate reader (Megu Molecular Instrument co., Ltd, Shanghai, China).
2.8. Observation of distant metastasis of tumor
On the 28th day after HIFU ablation, the lungs and axillary lymph nodes of tumor bearing rabbits in each group were anatomically sampled. The microscopic manifestations after H&E staining of tissue sections and macroscopic manifestations of tissue appearance were used to compare the distant metastasis of tumors in each group. The rate of tumor metastasis was calculated by the formula: tumor metastasis rate (100%) = number of animals showing metastasis/total number of animals × 100%.
2.9. Analysis of circulating tumor cells
The peripheral blood samples were collected from rabbits on the 0, 14th, and 28th day after HIFU ablation. The samples were pretreated by performing red blood cell lysis or centrifugation to remove interference from red blood cells and other cells. The processed samples were introduced into the Isolation by Size of Epithelial Tumor (ISET) filter device (Jingke Chemical Technology Co., Ltd, Shanghai, China). Blood traversed through an 8 μm polycarbonate membrane (Millipore Corporation, USA) under the gravity. Due to the small pore size of the filtration membrane, smaller normal blood cells were filtered out, while larger tumor cells were retained on the filter membrane, enabling the enrichment of tumor cells. The cells on the membrane were fixed using a cell fixative solution, followed by Papanicolaou staining. Subsequently, the cells were subjected to Papanicolaou staining and hematoxylin staining, and then differentiated using 1% hydrochloric acid and ethanol. The cells were dehydrated with ethanol at different concentrations for 2 min and subsequently stained with orange G6 dye. Finally, the cells were observed and counted using a light microscope. Circulating tumor cells often exhibit distinct morphological characteristics, such as irregular cell shape, large nuclear to plasma ratio, and metachromatic nuclei.
2.10. Statistical analysis
All statistical analyses were performed by GraphPad Prism 8.2.1 (GraphPad Software Inc., San Diego, CA, USA), and all continuous measurement data were expressed as the mean standard deviation (mean ± SD). Two-way ANOVA (group × time) was used for comparison between the two groups. *p < 0.05 was considered statistically significant, and **p < 0.001 was considered statistically significant.
3. Results
3.1. The comparison of ablation rates after HIFU ablation
To validate the successful establishment of the HIFU partial ablation model, B-mode ultrasound images were collected before and after the ablation procedure (). The tumor ablation rates for each group were determined by measuring the approximate volume of the hyperechoic region. The ablation rates were determined to be 80.2 ± 0.21% (80% ablation group) and 100.3 ± 0.17% (100% ablation group) (). To further confirm if the ablation in different regions by HIFU conforms to expectations, complete tumor tissues were excised immediately after HIFU ablation. The volume of coagulation necrosis was then measured using TTC staining: coagulation necrotic tissue resulting from ablation appears white, enabling clear differentiation from normal tissue (). The measured tumor ablation rates for the 80% and 100% ablation group were found to be 80.4 ± 0.17% and 100.5 ± 0.15% respectively (), which is consistent with the results obtained from the B-mode ultrasound measurements. These results confirm the successful establishment of the HIFU partial ablation model.
Figure 1. (A) B-mode ultrasonography images of VX2 breast tumor areas in each group before and immediately after HIFU ablation. After ablation, the sonographic images of each group showed obvious enhancement of tumor echo; (B) Quantitative calculation of approximate tumor volume before and after ablation by B-ultrasonography; (C) Observation of the extent of coagulation necrosis in each group. (D) Quantitative calculation of tumor volume in two group by TTC staining (n = 5, *p < 0.05, **p < 0.001).
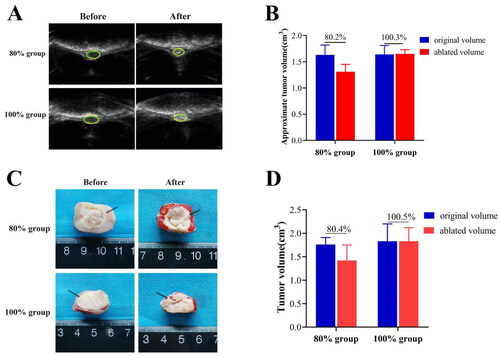
3.2. Histopathological changes of tumor area and peritumor after HIFU incomplete ablation
The histopathological structural changes of tumor area and peritumor tissue on the 1st and 21st day after HIFU ablation were observed. On 1st day after ablation, tumor coagulation necrosis occurred in the ablation area, and the tissues around the injured area in the 80% group exhibited congestion, evident by a purple-red congestive band (, thin black arrow shown). H&E staining observation showed that the tumor cells in the ablation target area of the two ablation groups were massively dissolved, including nuclear fragmentation and enlargement of the intercellular space. Especially, the tumor cells at the junction around the ablation area showed cell shrinkage, decreased cytoplasm, and loose arrangement (, thick black arrow shown). However, the red hyperemia zone completely disappeared at 21st day after ablation, the tumor cells were massively ruptured (, thin black arrow shown), necrotic and dissolved, the cell outline disappeared, fibroblast proliferation and new capillaries appeared around, and the necrotic part of the ablation target area was gradually absorbed (, thick black arrow shown).
Figure 2. The appearance of tumor tissue damage and the Observation of H&E staining after HIFU ablation in different areas at 1st day (A) and 21st days (B) (the thin black arrow in the tumor tissue refers to the purplish red hyperemic zone surrounding the ablation zone, while the thick arrow in H&E refers to the appearance of tumor ablation junction area, scale bar = 200 μm).
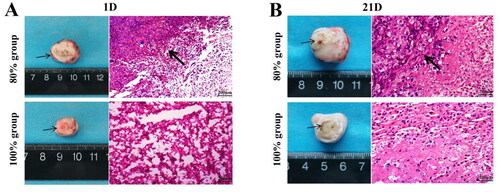
3.3. Detection of tumor inhibition ability after HIFU incomplete ablation
The short-term prognostic outcome in tumor-bearing rabbits post-HIFU ablation were assessed by analyzing tumor volume and body weight changes 21 days post-ablation. The tumor volume in the control group continued to increase with time, while the tumor inhibition rate at 21st day was 85.63 ± 0.25% in 80% group and 91.04 ± 17% in 100% group respectively, which was no significant difference (p > 0.05) (). In addition, the skin of the control group had different degrees of ulceration and crusting, while most of the tumors in the two ablation groups had been absorbed, and only a slight bulge was seen on the breast surface (). Moreover, the weight of the control group tumor bearing rabbit has significantly reduced, while the 80% and 100% ablation groups have not fluctuated after HIFU ablation ().
Figure 3. Changes of tumor volume and body weight of tumor bearing rabbits in different group within 21 days. (A) The curve diagram of tumor volume in each group of tumor bearing rabbits; (B) Calculation of tumor inhibition rate in each group at 21 days after HIFU ablation; (C)The tumor appearance observation of each group of tumor bearing rabbits; (D) The curve graph of the change of body weight of each group 21 days after HIFU ablation (n = 5, *p < 0.05, **p < 0.001, scale bar =10mm).
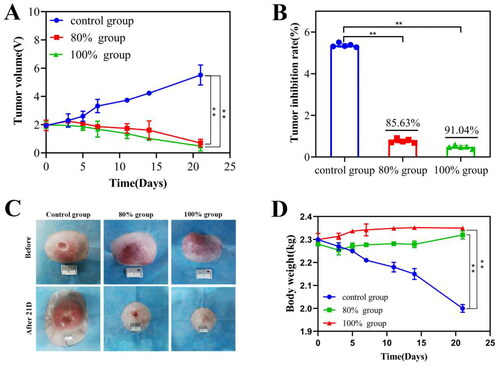
3.4. Tumor cell apoptosis dynamic change after HIFU incomplete ablation
Dynamic changes in tumor cell apoptosis at various time points post-HIFU ablation were observed using TUNEL staining, as depicted in . The results indicated that the positive expression of apoptotic cells (green fluorescence) was significantly increased in the 80% ablation group, and the prominent apoptotic areas primarily manifested in the junctional area and its surrounding regions (). Furthermore, a noteworthy surge in apoptosis was observed during the 3-7 day period after ablation (). However, the target area was almost coagulated necrotic tissue and only a small amount of residual tumor tissue was found to express apoptotic cells in the 100% ablation group, and there was a significant difference between 80% groups (65.26 ± 0.02%) and 100% group (21.76 ± 0.04%) to 21 days after HIFU ablation (p<0.001).
Figure 4. Tumor cell apoptosis was observed by TUNEL staining in each group after HIFU ablation in different range. (A) Observation of TUNEL fluorescence staining under confocal laser microscopy; (B) Fluorescence intensity analysis of TUNEL expression in each group at different time points after HIFU ablation (n = 5, *p < 0.05, **p < 0.001, scale bar = 100 μm).
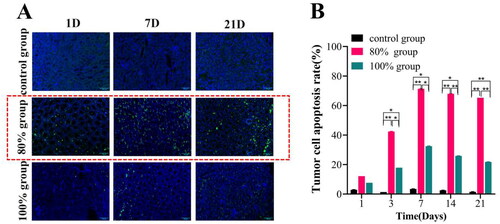
3.5. Tumor cell proliferation and expression of angiogenic factors after HIFU incomplete ablation
In order to explore the effects of incomplete HIFU ablation on tumor cell proliferation and tumor angiogenesis, we detected the expression of PCNA and CD31 at different time by immunohistochemical staining. A large area of brown-yellow nuclei (PCNA-positive cells) can be seen under the field of vision in control group, but almost no positive proliferating cells expressed in the 100% ablation group due to thoroughly coagulation necrosis and only a few proliferating cells in the junction area in 80% ablation group, but the expression of proliferating cells at the junction in 80% ablation group was obvious reduced at the 21st day after HIFU ablation (). Up to 21 days after HIFU ablation, there was still a significant statistical difference between the two groups (p < 0.05). However, both groups showed significantly lower tumor metastasis rates compared to the control group (p < 0.001) ().
Figure 5. The immunohistochemical analysis of PCNA and CD31 in each group after HIFU ablation in different range. Representative images of PCNA(A) and CD31(C) after 21 day of HIFU ablation in each group. Semi-quantitative analysis of PCNA(B) and CD31(D) positive expression at different time after ablation via AOD value (n = 5, *p < 0.05, **p < 0.001, scale bar = 100 μm).
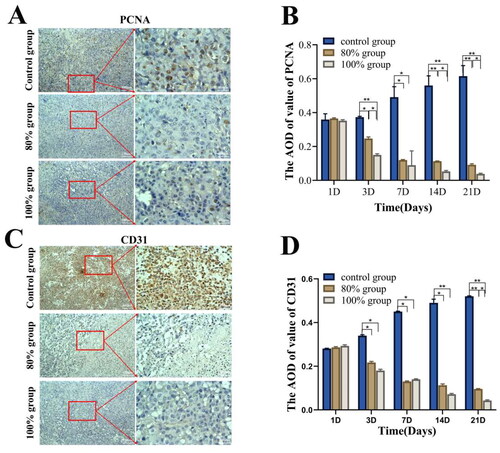
The results of CD31 immunohistochemical analysis are shown in . Compared to the continuous increase in strong positive staining in the control group, the 100% ablation group showed almost no expression of positive cells after ablation, while the 80% ablation group exhibited a significant decrease in brownish-yellow positive staining over time. On the 21st day after ablation, the AOD value of CD31 was different between the two ablation groups (p < 0.05), but when compared with the control group, the CD31 expression was significantly decreased in both ablation groups (p < 0.001).
3.6. Quantifcation of multiple immune factor expression after HIFU incomplete ablation
To assess the impact of incomplete HIFU ablation on tumor immunity, ELISA was employed to measure the expression of various immune factors under different HIFU ablation ranges, as shown in . The results showed that the expression levels of TGF-β1, IL-2R, IL-6, and IL-8 in the control group continued to increase over time, while the expression levels of TGF-β1 and IL-2R gradually decreased over time in the 80% ablation group and the 100% ablation group (). At 28 days post-ablation, its expression level was significantly lower than that in the control group (p < 0.05). However, the expression levels of IL-6, IL-8 and HSP-70 in the two ablation groups increased first and then decreased (), reached the peak on the 3rd day, and decreased significantly on the 7th day. There was no significant difference between the two ablation groups (p > 0.05). In addition, the expression of INF-γ in 80% ablation group and 100% ablation group increased with time (), and the difference was statistically significant compared with the control group at 28 days (p < 0.001), but there was no significant difference between 80% ablation group and 100% ablation group (p > 0.05). The changes in the expression levels of cytokines at different time points may be related to the tissue damage and inflammatory response induced by HIFU ablation.
3.7. Observation of distant metastasis after HIFU incomplete ablation
Macroscopic observation of tumor metastasis in various organs of the tumor-bearing rabbits from each group, 28 days post-HIFU ablation, are presented in and . In the control group, tumors progressively metastasized to distant sites over time, reaching a metastasis rate of 100% by 28 days. The most common sites of metastasis were the axillary lymph nodes and lungs. In contrast, the size of metastatic lesions was significantly smaller in 80% ablation group and 100% ablation group than in the control group (), and the rate of tumor metastasis was significantly reduced to 40% and 20% in the 80% ablation group and 100% ablation group, respectively () (p<0.05). The H&E staining analysis revealed a lower number of tumor cells with metastasis in the lung and axillary lymph nodes of group 80% ablation group (), suggesting its effectiveness in inhibiting distant metastasis.
Figure 7. Observation of distant metastasis of tumors in each group after 28 days of HIFU ablation. (A) Macro-observation of distant metastasis of tumor after HIFU ablation. (B) Quantitative analysis of tumor metastasis rate after in different group. (C) Observation of distant metastasis of tumor by H&E staining (scale bar = 200 μm). the tumor metastasis in the two treatment groups was lower than that in the control group (p < 0.05). (n = 5, *p < 0.05, **p < 0.001).
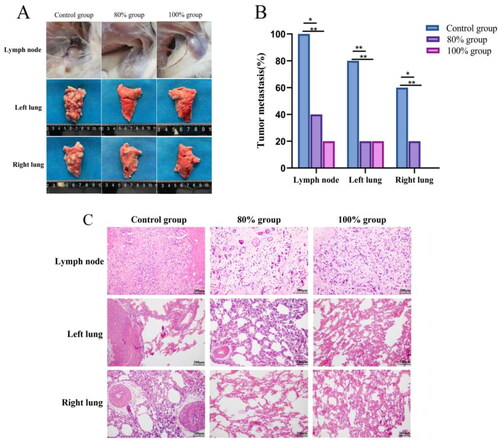
Table 1. Statistics on distant metastasis of tumors in each group at 28th day after HIFU ablation.
3.8. Observation of CTCs and expression of tumor metastasis-related factors
Peripheral blood samples from each group of tumor bearing rabbits were collected at various time points post-HIFU ablation. The CTCs in the peripheral blood of each group were enriched and identified using the ISET method, as illustrated in . The results shown that more CTCs could be enriched in the peripheral blood of the control group. In the 100% ablation group, CTCs were undetectable in the peripheral blood on the 14th day post-HIFU ablation. In the 80% ablation group, a few CTCs were observed on the 14th day, but none were detected by the 28th day (). In addition, the expression levels of tumor metastasis-related factors of MMP and VEGF in the control group continued to increase with time. While it continued to decrease after HIFU ablation in 80% and 100% group, and there was no significant difference between the two groups ().
Figure 8. Expression detection of tumor distant metastasis-related after HIFU incomplete ablation. (A)Schematic diagram of enrichment of CTCs by TSET device; (B)Detection of circulating tumor cells in each group at different time points after HIFU ablation (scale bar = 200 μm); (C)Expression level of MMP factor; (D) Expression level of VEGF factor (n = 5, *p < 0.05, **p < 0.001).
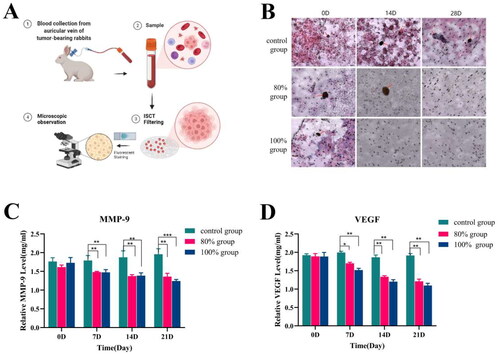
Discussion
With the continuous expansion of clinical indications for HIFU [Citation20,Citation21], the incomplete ablative therapy may be a complementary approach to HIFU ablative therapy, and it is very important to clarify the biological effects and outcomes of tumor after incomplete ablative therapy. The previous studies on HIFU treatment almost have focused on improving thermal ablation efficiency through more precise control of targeting and monitoring of lesion formation, while largely ignoring the various biological responses that HIFU may induce [Citation22,Citation23]. In our study, 80% volume of rabbit VX2 breast tumor was ablated by HIFU under B-ultrasound monitoring, and the local biological changes, metastasis and prognosis of the tumor after ablation were observed. The experimental results demonstrated that HIFU ablation at 80% incompleteness could effectively induce tumor damage and suppressed the proliferation and metastasis of tumor cells, comparable to complete ablation. This outcome led to improved prognostic outcomes. This may be related to the biological effects induced by incomplete ablation of HIFU [Citation24,Citation25].
Apoptosis is an autonomous and orderly death phenomenon of cells under the activation, expression and regulation of a series of genes [Citation26]. Some studies [Citation27,Citation28] have reported that HIFU ablation can cause apoptosis in the surrounding tissue of the ablation target due to thermal effects, which was also confirmed in our study. In the 100% ablation group, only a small amount of positive expression of apoptosis was seen at the junction because the tumor was completely covered. However, in the incomplete ablation group, the residual area surrounding the tumor showed significant apoptosis 14 days after ablation and gradually decreased after 21 days (). In addition, the immunohistochemical examination of PCNA and CD31 staining showed that tumor cell proliferation and tumor blood vessels were also significantly inhibited, which demonstrated that HIFU effectively incomplete ablation can promote residual tumor apoptosis, inhibit cell proliferation and angiogenesis, and thus achieve anti-tumor growth and metastasis (). However, in real-world scenarios, tumor cells might also disseminate to organs that have not been identified or evaluated. The utilization of luminescent or fluorescent labeling techniques may potentially enhance our ability to accurately detect metastatic foci in previously unrecognized locations. This aspect will indeed constitute an area for further incorporation and advancement in our forthcoming research endeavors.
In addition to the thermal effects of HIFU treatment, recent studies have also demonstrated it possesses the potential to elicit the host’s own anti-tumor immune response [Citation29,Citation30]. The process of HIFU ablation induces mechanical disruption and thermal effects on tissues, leading to tumor cell death and release of cellular components. Among these components, DAMP factors are released into the extracellular space or bloodstream, serving as danger signals that directly or indirectly stimulate the initiation of adaptive immune responses by the immune system [Citation31,Citation32]. In this study, we observed a significant upregulation of factors such as INF-γ and HSP-70 in the expression levels after HIFU ablation in both ablation groups. These DAMP factors are considered signaling molecules that enable the immune system to recognize tumor cells, interacting with specific receptors on immune cells such as toll-like receptors, thereby activating immune responses.
Furthermore, DAMPs can also activate inflammatory responses, where inflammatory cells release cytokines or chemokines that further stimulate immune responses. Cytokines such as IL-6, IL-8, and HSP-70 play important roles in the immune and inflammatory responses in the body [Citation33]. IL-6, as a pro-inflammatory cytokine, can be transiently upregulated in response to the inflammatory reaction triggered by HIFU ablation, thereby activating immune cells and enhancing anti-tumor immune responses. IL-8 is a chemokine involved in inflammation and immune cell recruitment, and it can be stimulated by HIFU treatment, leading to the recruitment of immune cells to the tumor site and potentially enhancing immune-mediated tumor destruction. HSP-70 comprises a group of heat-sensitive proteins that form immune complexes with peptide antigens derived from other cells. These proteins stimulate the body’s T lymphocyte system and play an important role in tumor immunity [Citation34].
The inflammatory response induced by HIFU is most pronounced in the first three days after ablation, and our results also show a significant increase in the expression levels of these factors on the third day after HIFU ablation. However, as time progresses, this inflammatory response gradually subsides, and the expression levels of these factors visibly decrease from the 3rd day to the 7th day. On the 7th day, the inflammatory response diminishes, and the expression of these factors begins to gradually decrease over time. It is worth noting that in addition to their involvement in the inflammatory response, IL-6, IL-8, and IL-2R also function as important tumor-associated cytokines that participate in promoting tumor cell proliferation, invasion, metastasis, and angiogenesis. Prolonged high expression levels of IL-6, IL-8, and IL-2R are often associated with tumor malignancy and poor prognosis. The results of this study also demonstrate a significant decrease in the expression of these cytokines in the incomplete ablation group approximately one month after HIFU treatment, confirming the significant inhibitory effect of HIFU incomplete ablation therapy on tumor proliferation.
On the one hand, the bubbles generated by the cavitation effect of HIFU undergo continuous growth and rupture in the liquid medium when exposed to ultrasound, giving rise to intense physical reactions, including the generation of shock waves and microair flows. These physical effects can cause mechanical damage and thermal changes in tissues, leading to necrosis and coagulation necrosis of surrounding cells. On the other hand, from the 3rd day after ablation, the thermal effect induced by ablation began to act on the surrounding tumor cells, the tumor cells ruptured, the antigens in the cytoplasm and nucleus were fully exposed and released, and the release of immunomodulatory factors changed the tumor cell’s immunogenicity, enhancing the body’s immune response to tumors. The combined action of this biological effect stimulates the potential of the body’s anti-tumor immune response. To sum up, the mechanisms of tumor outcome after ablation under the two ablation methods are different: (1) The full ablation primarily relies on the thermal effect to induce coagulation necrosis in all tumor cells. Over time, the necrotic tumor tissue undergoes progressive changes, ultimately leading to complete absorption of the tumor within a timeframe of 28 to 35 days; (2) The incomplete ablation can not only cause coagulation necrosis through thermal ablation, but also induce persistent apoptosis of tumor cells outside the ablation target area through the cavitation effect, thereby achieving the purpose of inhibiting the growth of residual tumors.
Conclusion
In summary, we performed incomplete ablation of HIFU on the rabbit VX2 breast cancer model by HIFU ablating 80% tumors volume, and observed the damage effect and prognosis of the tumor after incomplete HIFU ablation. Our study demonstrated that HIFU incomplete ablation with an ablation rate of 80% could markedly impair tumors and suppress metastasis, which may be related to enhance residual tumor cell apoptosis and stimulate anti-tumor immunity. HIFU incomplete ablation can provide a new treatment idea for patients who cannot tolerate complete ablation or do not have the conditions for HIFU complete ablation, and provide a reference for the basic research of HIFU incomplete ablation of tumors.
Authors’ contributions
Xiaoling Feng, Jianhu Li and Hongjian Liao conducted molecular and animal experiments. Min Yang, Zhifei Zhang completed the sequencing data analysis and participated in data. Xiaoling Feng completed the manuscript writing. Qi Wang and Yonghong Du conceived and designed the study, and revised the manuscript.
Supplemental Material
Download PDF (290.5 KB)Acknowledgments
Thanks to the State Key Laboratory of Ultrasound in Medicine and Engineering, College of Biomedical Engineering for providing the experimental platform. Thanks to the members of this research group, advisors and mentors for their help. Thanks to the support of the Chongqing Graduate Research Innovation Project Fund (No. CYS20213).
Disclosure statement
No potential conflict of interest was reported by the author(s).
Additional information
Funding
References
- Azamjah N, Soltan-Zadeh Y, Zayeri F. Global trend of breast cancer mortality rate: a 25-Year study. Asian Pac J Cancer Prev. 2019;20(7):2015–2020. doi: 10.31557/APJCP.2019.20.7.2015.
- Sung H, Ferlay J, Siegel RL, et al. Global cancer statistics 2020: GLOBOCAN estimates of incidence and mortality worldwide for 36 cancers in 185 countries. Cancer J Clin. 2021;71(3):209–249.
- Siegel RL, Miller KD, Fuchs HE, et al. Cancer statistics, 2021. CA Cancer J Clin. 2021;71(1):7–33. doi: 10.3322/caac.21654.
- Waks AG, Winer EP. Breast cancer treatment: a review. J Am Med Associat. 2019;321(3):288–300. doi: 10.1001/jama.2018.19323.
- Yin L, Duan JJ, Bian XW, et al. Triple-negative breast cancer molecular subtyping and treatment progress. Breast Cancer Res. 2020;22(1):61. doi: 10.1186/s13058-020-01296-5.
- Miccoli M. Recent advances in the treatment of breast cancer. Anticancer Agents Med Chem. 2022;22(4):747–747. doi: 10.2174/187152062204220217144704.
- Lovelace DL, Mcdaniel LR, Golden D. Long-Term effects of breast cancer surgery, treatment, and survivor care. J Midwifery Womens Health. 2019;64(6):713–724. doi: 10.1111/jmwh.13012.
- Bale R, Putzer D, Schullian P. Local treatment of breast cancer liver metastasis. Cancers (Basel). 2019;11(9):1341. doi: 10.3390/cancers11091341.
- Maloney E, Hwang JH. Emerging HIFU applications in cancer therapy. Int J Hyperthermia. 2015;31(3):302–309. doi: 10.3109/02656736.2014.969789.
- Marinova M, Rauch M, Mucke M, et al. High-intensity focused ultrasound (HIFU) for pancreatic carcinoma: evaluation of feasibility, reduction of tumour volume and pain intensity. Eur Radiol. 2016;26(11):4047–4056. doi: 10.1007/s00330-016-4239-0.
- Peek M, Wu F. High-intensity focused ultrasound in the treatment of breast tumours. Ecancermedicalscience. 2018;12:794. doi: 10.3332/ecancer.2018.794.
- Marinova M, Wilhelm-Buchstab T, Strunk H. Advanced pancreatic cancer: high-Intensity focused ultrasound (HIFU) and other local ablative therapies. Rofo. 2019;191(3):216–227. doi: 10.1055/a-0820-5564.
- Ter Haar G. HIFU tissue ablation: concept and devices. Adv Exp Med Biol. 2016;880:3–20.
- Feril LB, Fernan RL, Tachibana K. High-Intensity focused ultrasound in the treatment of breast cancer. Curr Med Chem. 2021;28(25):5179–5188. doi: 10.2174/0929867327666201111143206.
- Peek MC, Ahmed M, Napoli A, et al. Systematic review of high-intensity focused ultrasound ablation in the treatment of breast cancer. Br J Surg. 2015;102(8):873–882; discussion 882. doi: 10.1002/bjs.9793.
- Yin N, Hu L, Xiao ZB, et al. Factors influencing thermal injury to skin and abdominal wall structures in HIFU ablation of uterine fibroids. Int J Hyperthermia. 2018;34(8):1298–1303. doi: 10.1080/02656736.2018.1433880.
- Zhou Y, Cunitz BW, Dunmire B, et al. Characterization and ex vivo evaluation of an extracorporeal high-intensity focused ultrasound (HIFU) system. J Appl Clin Med Phys. 2021;22(9):345–359. doi: 10.1002/acm2.13074.
- Elhelf I, Albahar H, Shah U, et al. High intensity focused ultrasound: The fundamentals, clinical applications and research trends. Diagn Interv Imaging. 2018;99(6):349–359.
- Mauri G, Sconfienza LM, Pescatori LC, et al. Technical success, technique efficacy and complications of minimally-invasive imaging-guided percutaneous ablation procedures of breast cancer: a systematic review and meta-analysis. Eur Radiol. 2017;27(8):3199–3210. doi: 10.1007/s00330-016-4668-9.
- Palyga I, Palyga R, Mlynarczyk J, et al. The current state and future perspectives of high intensity focused ultrasound (HIFU) ablation for benign thyroid nodules. Gland Surg. 2020;9(2):95–104.
- Scipione R, Anzidei M, Bazzocchi A, et al. HIFU for bone metastases and other musculoskeletal applications. Semin Intervent Radiol. 2018;35(4):261–267. doi: 10.1055/s-0038-1673363.
- Xu D, Zou W, Luo Y, et al. Feasibility between bifidobacteria targeting and changes in the acoustic environment of tumor tissue for synergistic HIFU. Sci Rep. 2020;10(1):7772. doi: 10.1038/s41598-020-64661-6.
- Zhang Y, Yong L, Luo Y, et al. Enhancement of HIFU ablation by sonosensitizer-loading liquid fluorocarbon nanoparticles with pre-targeting in a mouse model. Sci Rep. 2019;9(1):6982. doi: 10.1038/s41598-019-43416-y.
- Sparchez Z, Mocan T, Radu P, et al. Prognostic factors after percutaneous radiofrequency ablation in the treatment of hepatocellular carcinoma. Impact of incomplete ablation on recurrence and overall survival rates. J Gastrointestin Liver Dis. 2018;27(4):399–407. doi: 10.15403/jgld.2014.1121.274.pro.
- Shi L, Wang J, Ding N, et al. Inflammation induced by incomplete radiofrequency ablation accelerates tumor progression and hinders PD-1 immunotherapy. Nat Commun. 2019;10(1):5421. doi: 10.1038/s41467-019-13204-3.
- Ning Z, Zhu Z, Wang H, et al. High-intensity focused ultrasound enhances the effect of bufalin by inducing apoptosis in pancreatic cancer cells. Onco Targets Ther. 2019;12:1161–1170. doi: 10.2147/OTT.S185953.
- Suehiro S, Ohnishi T, Yamashita D, et al. Enhancement of antitumor activity by using 5-ALA-mediated sonodynamic therapy to induce apoptosis in malignant gliomas: significance of high-intensity focused ultrasound on 5-ALA-SDT in a mouse glioma model. J Neurosurg. 2018;129(6):1416–1428. doi: 10.3171/2017.6.JNS162398.
- Wang Y, Zhai D. High intensity focused ultrasound inhibits breast cancer cell proliferation and promotes cell apoptosis via miR-222-3p/p27Kip1 axis. Int J Clin Exp Med. 2020;13:2205–2215.
- Zhu XQ, Lu P, Xu ZL, et al. Alterations in immune response profile of Tumor-Draining lymph nodes after High-Intensity focused ultrasound ablation of breast cancer patients. Cells. 2021;10(12):3346. doi: 10.3390/cells10123346.
- Eranki A, Srinivasan P, Ries M, et al. High-Intensity focused ultrasound (HIFU) triggers immune sensitization of refractory murine neuroblastoma to checkpoint inhibitor therapy. Clin Cancer Res. 2020;26(5):1152–1161. doi: 10.1158/1078-0432.CCR-19-1604.
- Fucikova J, Kepp O, Kasikova L, et al. Detection of immunogenic cell death and its relevance for cancer therapy. Cell Death Dis. 2020;11(11):1013–1020. doi: 10.1038/s41419-020-03221-2.
- Ahmed A, Tait S. Targeting immunogenic cell death in cancer. Mol Oncol. 2020;14(12):2994–3006. doi: 10.1002/1878-0261.12851.
- Kim B, Seo Y, Kwon JH, et al. IL-6 and IL-8, secreted by myofibroblasts in the tumor microenvironment, activate HES1 to expand the cancer stem cell population in early colorectal tumor. Mol Carcinog. 2021;60(3):188–200. doi: 10.1002/mc.23283.
- Oh S, Kim HM, Batsukh S, et al. High-Intensity focused ultrasound induces adipogenesis via control of cilia in Adipose-Derived stem cells in subcutaneous adipose tissue. Int J Mol Sci. 2022;23(16):8866. doi: 10.3390/ijms23168866.