Abstract
Purpose
To evaluate the safety and efficacy of indocyanine green fluorescence imaging for real-time guidance of laparoscopic thermal ablation in patients with liver cancer.
Materials and methods
A total of 27 patients with 40 liver lesions underwent fluorescence-assisted laparoscopic ablation between January 2020 to March 2023. The sensitivity of indocyanine green (ICG)-fluorescence imaging, technique effectiveness rate and complications of fluorescence-assisted laparoscopic thermal ablation were evaluated.
Results
In total, 33 out of the 40 lesions were identified by ICG-fluorescence imaging technique, with the sensitivity of 82.5%. The sensitivity of ICG-fluorescence imaging of tumor detection in liver surface of parenchyma was significantly higher than that in the deeply located hepatic parenchyma (96.8% vs 33.3%, p = 0.002). ICG-fluorescence imaging procedures detected 4 lesions that cannot be seen on intraoperative ultrasound. It provides clear demarcation lines on the hepatic surface. Technical success is achieved if the necrotic zone had at least a 5 mm ablative margin around the outer edge of the ICG-fluorescence image. Technical success of fluorescence laparoscopic radiofrequency ablation (FLRFA) and fluorescence laparoscopic microwave ablation (FLMWA) was 100% (27/27). Technical effectiveness is defined by the complete necrotic lesions of the local tumor tissue during follow-up. According to the CT/MRI one month after FLRFA or FLMWA, the technical efficacy rate was 92.5% (37/40) and local tumor progression occurred in 7.5% (3/40) of the enrolled lesions. During the follow-up period, no major complications were observed.
Conclusion
ICG-fluorescence imaging guided laparoscopic thermal ablation was feasible, safe and effective.
1. Introduction
Liver cancer remains a global health challenge and its incidence is growing around the world [Citation1]. Hepatocellular carcinoma (HCC) accounts for 80%-90% of primary liver cancers [Citation2]. In addition, due to its unique anatomical and physiological characteristics, extrahepatic tumors such as gastric cancer, breast cancer, and pancreatic cancer are prone to metastasize to the liver, especially colorectal cancer [Citation3]. Metastatic liver malignancies are 18–40 times more common than primary liver malignancies [Citation4].
In clinical practice, curative therapeutic modalities include surgical resection, liver transplantation and ablation techniques like radiofrequency ablation (RFA) [Citation5]. RFA is recommend as the first-line treatment for single tumor smaller than 2 cm [Citation6]. Besides, microwave ablation (MWA) has been proved to be a safe and effective procedure in the treatment of liver cancers. However, more and more studies showed better recurrence free survival in surgical resection group in both Randomized Controlled Trials (RCTs) and Norm-Referenced Tests (NRTs). The cause of local tumor recurrence can be explained as an incomplete primary tumor control ability [Citation7]. The objective of thermal ablation of liver cancer is to completely coagulate the tumor, leaving no live malignant tissue. The importance of partial ablation during thermal ablation is a significant yet unsolved problem. After a single session of thermal ablation, incomplete ablation is common (3%-52%) [Citation8]. The wide variation of incomplete ablation rate might be partly due to the different tumor sizes in the studies. There are several causes of insufficient thermal ablation, including both procedural and biological characteristics of the tumor. Several researchers have proposed that the location and morphology of tumors are strongly connected to treatment success [Citation9, Citation10]. For instance, due to the heat-sink effect, thermal ablation for tumors near to major arteries may frequently result in incomplete ablation [Citation11]. Moreover, tumor size larger than 3 cm is an influential factor leading to patrial ablation [Citation12]. In addition to the insufficient ablation margin, the difficulties in identifying occult malignant lesions account for the incomplete tumor ablation [Citation13, Citation14].
Fluorescence imaging is recognized as a novel modality that is capable of tumor imaging. It is monitored by sensors and projected onto the display to provide real-time guidance for tumor imaging [Citation15]. Clinical approved dye indocyanine green (ICG), a nontoxic fluorescent dye was used for Near-Infrared Region II (NIR-II) fluorescence imaging. After being administered, ICG is immediately bound to plasma albumin after intravenous administration and then taken up by liver parenchymal cells. Normal liver tissue can excrete ICG through the biliary system after ingesting it, but tumor tissues lack a normal biliary system, resulting in a significantly longer residence time of ICG in tumor tissue. As a result, when exposed to NIR II after a period of metabolism, the tumor tissue will generate green fluorescence, revealing the morphological outline of the tumor [Citation16]. The fluorescence imaging system is used to visualize the real-time fluorescence emitted by the ICG. Surgeons can switch between white light and NIR modes during the procedure. These techniques achieved highly sensitive of liver cancer detection by visualization of the disordered biliary excretion of ICG that present in liver cancers and noncancerous liver tissues [Citation17]. Therefore, we hypothesized that indocyanine green fluorescence imaging (IFI)-guided thermal ablation can be performed effectively in hepatic malignancies that are invisible or poorly visualized by traditional preoperative examination.
In 2008, Aoki et al. first reported the use of fluorescence imaging for segmental localization during liver surgery [Citation18]. Positive staining can be achieved by gradually injecting ICG into the portal vein branches of the tumor-bearing segment. After standard Glisson dissection, ICG is injected intravenously for negative staining, and the segmental pedicle is clamped or ligated to prevent blood from entering the tumor-bearing segment. Negative staining is easier to perform than positive staining and is mainly suitable for resection of left segments (II and III) or lower segments (IVb, V, VI), which are easy to reach the roots of segmental portal vein branches [Citation19, Citation20]. In liver resection, fluorescence imaging identified 25 of 27 HCC lesions, which amounts to a 92.6% success rate [Citation21]. It has also been shown to detect liver metastases from pancreatic cancer [Citation22] and melanoma [Citation23].
In the present study, we aimed to investigate the safety and efficacy of ICG fluorescence imaging for real-time guidance of laparoscopic thermal ablation in patients with liver cancer.
2. Materials and methods
2.1. Study population and lesions
This study was approved by the Ethics Committee of Zhejiang University School of Medicine and was performed according to the guidelines of the Declaration of Helsinki. From January 2020 to March 2023, patients who underwent fluorescence laparoscopic ablation in the surgical department of the 2nd and 4th Affiliated Hospital of Zhejiang University were consecutively included in this retrospective study. The diagnosis of HCC was based on the typical imaging appearance of contrast enhanced computed tomography (CECT) or magnetic resonance imaging (CEMRI) combined with clinical findings according to AASLD [Citation24, Citation25]. The diagnostic test of CRLM was followed by consensus recommendations of the National Comprehensive Cancer Network (NCCN) and the European Society for Medical Oncology (ESMO) [Citation26]. And the patients of ICC were histologically or cytological documented.
The inclusion criteria were as follows: tumor size ≤5 cm in diameter, or fewer than three nodules all ≤3cm, without extrahepatic metastasis [Citation27]; Child-Pugh A or B; the absence of intractable ascites and uncorrectable coagulopathy; prothrombin activity above 40% and a platelet count of more than 500,000/L [Citation28]; RFA or MWA with curative intent regardless of prior liver-directed treatment.
The exclusion criteria were as follows: presence of vascular invasion and extrahepatic metastases; severe clotting impairment; sever cardiopulmonary dysfunction or uncontrolled liver disease decompensation [Citation29].
The results of the patients were retrospectively reviewed.
2.2. Procedure
In this study, ablation was performed using the KANG YOU microwave ablation system (KY-2000) with a 14-gauge probe (#KY-2450B-2), featuring 7 mm active tips and the cool-tip radiofrequency ablation system (Covidien, Boulder, Colombia, CO) with #21-863471 probes (17-gauge), featuring 5 mm active tips, utilized water cooling. The OptoMedic (OPTO-CAM2100) or the Mindray fluorescence imaging system consisted of the camera (H-R1-82RE-4M) and endoscope (G-01030A) were used to scan the lesions and guide probes for thermal ablation procedures. The system comprises a high-end full-definition camera system connected to a ICG telescope. The system’s light source provides both visible and NIR excitation light (805 nm). The telescope is equipped with a specialized beam combiner prism and two sets of Complementary Metal-Oxide-Semiconductor (CMOS) sensors to achieve the effect of segmentation or combination. The surgeon can use a switch to change from white light to NIR. In bright fluorescence mode, the near-infrared signal was overlaid in green onto the white light image. The fluorescence imaging systems showed comparable performance in terms of imaging principles, modes (including brightfield and fluorescence modes), and image clarity. Contrast-enhanced CT or MRI, conducted prior to FLRFA or FLMWA, were used to assess the distance between the lesions and the liver surface of parenchyma and to assess the size of lesions for planned treatments. In this study, a sequential approach was adopted to target lesions using ultrasound (US) and fluorescence imaging. Deep-seated tumor’s part was first targeted using percutaneous US guidance, followed by superficial lesions guided by fluorescence imaging. The intent of this two-step process was to comprehensively treat both deep and superficial tumors. Specifically, US was utilized primarily to identify and ablate tumors embedded in deep tissues. Subsequently, fluorescence imaging was employed to guide the ablation of superficial lesions. All lesions were covered with gauze to prevent heat conduction from damaging surrounding organs. The overarching goal was to create a 0.5–1 cm ablation margin to minimize local tumor progression.
2.3. ICG-fluorescence imaging monitoring and evaluation
2.3.1. ICG-fluorescence imaging
Preoperatively, a dose of 0.5 mg/kg ICG (H20055881) was administered intravenously 2 days before the produces. The in vivo ICG-fluorescence imaging was performed to identify hepatic lesions and evaluate treatment effectiveness.
2.3.2. Evaluation of treatment response
Technical success was assessed by ICG-fluorescence imaging after the procedures immediately. Technical success achieved if necrotic zone covered the edge of ICG-fluorescence imaging with at least a 5 mm ablative margin [Citation30]. The necrotic zone was assessed using CECT or CEMRI. Specifically, the necrotic zone typically manifested as a round or oval area that demonstrated a defect in contrast enhancement at CECT or CEMRI imaging. During the arterial phase of imaging, no enhancement was observed in the ablated tumor lesions, indicating necrosis.
2.4. Follow-up
Adverse events were evaluated using the National Cancer Institute Common Terminology Criteria for Adverse Events Version 4.0 [Citation31]. The technical effectiveness of FLRFA and FLMWA was assessed using CECT or CEMRI one month after thermal ablation procedure. Technical effectiveness refers to complete necrotic lesions of the local tumor tissue. Local tumor progression (LTP) were defined as the appearance of tumor at the edge of the ablation zone during the one-month follow-up period [Citation29].
2.5. Statistical analysis
Categorical variables are described as absolute numbers and percentages. Continuous variables are summarized as mean ± standard deviation. We used univariate logistic regression to assess whether variables influence the observation of ICG-fluorescence. The variables include one continuous variable (The distance from the tumor to the liver surface) and three binary variables (the presence or absence of cirrhosis, whether the tumor is located on the surface of the liver, and whether the distance from the tumor to the liver surface is greater than 0.8 cm or not). Logistic Regression was used to analyze the date. A p-value less than 0.05 was significant. Date was compiled using Microsoft Excel (Microsoft Crop., Redmond, WA, USA) and analyzed using SPSS statistics 27.0(IBM Corp., Armonk, NY, USA).
3. Results
3.1. Patient characteristics
A total of 27 patients with 40 lesions were included in this study ( and Citation2). In total, the mean age of the 27 patients (23 males and 4 females) was 66.6 ± 9.2 (range: 52–85 years). Baseline characteristics of the study population are shown in . 40 lesions were detected using contrast enhanced CT or MRI before procedures. The mean tumor diameter was 1.65 ± 0.58 cm. Among them, 17 patients (25 lesions) had primary liver cancers, and 10 patients (15 lesions) had liver metastases with all from colorectal cancer. Background liver disease included chronic viral hepatitis in 13 patients and cirrhosis in 13 patients. The variables of cirrhosis have no apparent effect on ICG signal intensity in liver tumors (p = 0.281). Detailed information on each patient is shown in .
Table 1. Detailed patient information.
3.2. ICG-fluorescence imaging procedures
ICG-Fluorescence imaging procedures were successfully performed in 27 patients. Tumor characteristics and the observation under ICG-Fluorescence imaging were summarized in . Among all patients, 12 patients underwent FLRFA. Regarding the generation of images like those in , we followed a specific procedure. First, the camera lens was white-balanced to ensure the accurate representation of colors on the monitor. Subsequently, the imaging mode was adjusted to highlight the bright green fluorescence. In , an image taken under white light was depicted. When the tissue was exposed to specific NIR wavelengths (805 nm), it emitted fluorescence, which was then received and processed by the imaging device. The resulting green fluorescence signal was then superimposed on the white light image to create the composite images seen in . ICG-fluorescence imaging technique identified 33 of the total 40 lesions that were preoperative diagnosed of primary or metastatic liver tumors by CEMRI or CECT, demonstrating sensitivity of 82.5% (). The mean diameter of 33 identifiable tumors was 1.63 ± 0.57 cm. The mean diameter of 7 lesions that were not identified by ICG- fluorescence imaging was 1.71 ± 0.67 cm. Notably, the diameter of tumor had no effect on the observation of ICG-fluorescence (p = 0.735). Among the 40 lesions, 28 lesions were in the liver surface or in the cut surface of parenchyma. ICG-Fluorescence imaging procedures provided clear demarcation lines on the hepatic surface, aiding surgeons in accurately identifying and targeting tumor lesions by distinguishing and visualizing the boundary between tumor and non-tumor tissue. The sensitivity of ICG-Fluorescence imaging for detecting tumor in liver surface or in the cut surface of parenchyma was significantly higher than that in the deeply located hepatic parenchyma (96.4% vs 50.0%, p = 0.005). 40 lesions were further subdivided into α < 0.8 cm group and α ≥ 0.8 cm group according to the distance between the lesion and the liver surface or the cut surface of parenchyma. There was significant difference in sensitivity of ICG-Fluorescence imaging between the α < 0.8 cm group and α ≥ 0.8 cm group (96.8% vs 33.3%, p = 0.002). In one patient with local recurrence 7-month after RFA, ICG helped to detect the tumor around the ablation area (). Besides, ICG-Fluorescence imaging procedures found 8 lesions that cannot be seen on intraoperative ultrasound. Surprisingly, a combination of intraoperative ultrasound and ICG- fluorescence imaging technique detected all the lesions.
Figure 1. ICG- fluorescence imaging of the patient with liver cancer (Case 22). (A) Tumor images without ICG-fluorescence imaging (B) Tumor images with ICG-fluorescence imaging (C) Inserting the needle to ablate the tumor at the edge with ICG green fluorescence. (D) Tumor images with ICG-fluorescence imaging after MWA.
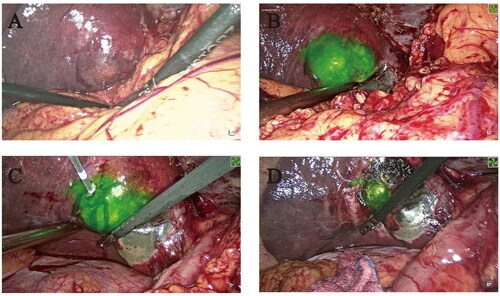
Figure 2. The tumor recurred at the same site as the initial thermal ablation site (Case 27). (A) The enhanced magnetic resonance imaging of tumor (B) Tumor imaging without ICG fluorescence imaging (C) Tumor imaging with ICG fluorescence imaging (D) Residual fluorescence was found in tumors after FLRFA.
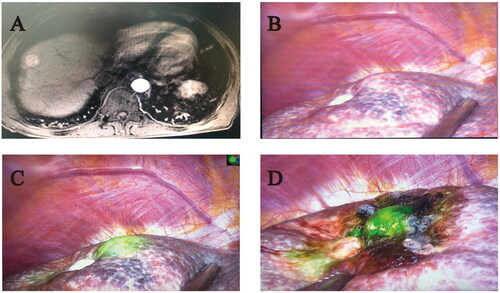
Table 2. Detailed tumors information.
3.3. Follow-up
40 lesions were all successfully ablated. TS (40/40, 100%) were observed in all treated tumors. CECT or CEMRI were conducted one month after FLRFA or FLMWA. summarizes the characteristics of ablated lesions. Among 40 liver lesions, 37 lesions had no recurrence/metastases, yielding a technical efficacy rate of 92.5% (37/40). LTP occurred in 7.5% (3/40) of the enrolled lesions. Patients with the residual tumor and insufficient tumor-free margins received re-ablation or further medical treatment.
Intraoperative fluorescent imaging demonstrated the residual tumor on the surface of the remnant liver, requiring an additional RFA or MWA produces (). The ICG-Fluorescent imaging of a portion of lesions were still visible after FLRFA or FLMWA ().
Figure 3. ICG fluorescence remained after ultrasound-guided ablation (Case 26). (A) Tumor imaging with ICG fluorescence imaging after ultrasound-guided percutaneous ablation (B) Inserting the needle to ablate the tumor at the edge with ICG green fluorescence. (C) Tumor fluorescence imaging after complete ablation (D) Residual fluorescence was found in tumors after ultrasound-guided microwave ablation. (E) FLWMA were performed to ablate tumor with the residue green fluorescence. (F) The green fluorescence was still presented in the tumor after FLMWA.
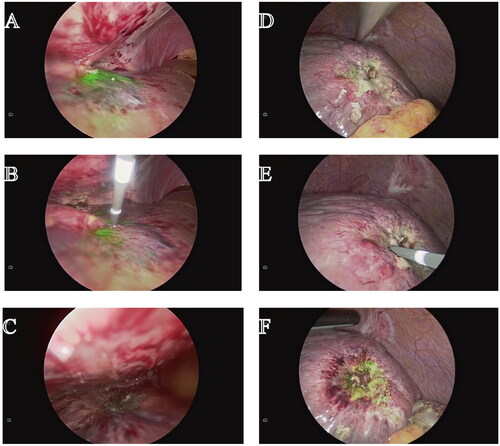
3.4. Perioperative complications
None of the patients experienced death due to FLRFA or FLMWA treatment. The average length of hospital stay was 8.2 ± 3.9 day. There were no serious grade 3,4 AEs such as intrahepatic hemorrhage, biliary fistula, intestinal perforation, bile duct stricture, cholecystitis, diaphragm damage, skin burn or severe abdominal pain after treatment. Almost all cases had fever ranging from 37 to 39.2 °C at the 1st-2nd day. After symptomatic treatment, the body temperature returned to normal. Additionally, all patients exhibited varying degrees of liver function damage, with 20 out of 27 classified as Child-Pugh A postoperatively and 7 as Child-Pugh B. Among the 7 patients classified as Child-Pugh B, 2 were already classified as Child-Pugh B preoperatively, while 5 transitioned from Child-Pugh A to Child-Pugh B. In each case, liver function damage occurred within the first 24 h after the procedure, did not necessitate intensive care, and remission occurred within 5–12 days.
4. Discussion
To our best knowledge, this is the first investigation on the safety and efficacy of indocyanine green fluorescence imaging for real-time guidance of laparoscopic thermal ablation in patients with liver cancers. The current study showed 96.8% sensitivity in detecting subscapular tumors within 8 mm of the liver surface of the liver parenchyma. And logistic regression analysis determined that tumor size, liver disease conditions and functions were not significantly associated with sensitivity. In the present study, the technical success rate of FLRFA and FLMWA was 100% and the technical efficacy rate was 92.5%. In addition, no serious complication was observed in the follow-up periods.
Although thermal ablation significantly improves the survival outcomes of patients with liver cancer, it remains an extremely challenging procedure with a high rate of local recurrence. It has been reported that when RFA was used as the first-line treatment for early-stage liver cancer, the two-year cumulative recurrence rate was 31.1% and the two-year cumulative mortality rate was 28.9% [Citation32]. MWA is theoretically superior to RFA. However, in a recent phase 2 RCT, the primary end point did not differ from RFA, and the local tumor progression after MWA was 2 years [Citation33]. Several adverse prognostic factors for recurrence have been identified, such as tumor size, positive ablation margin and ascites [Citation34, Citation35]. In traditional surgery, intraoperative ultrasound is widely used for thermal ablation navigation [Citation36]. However, the difficulty is exacerbated when ultrasonography targeted tumor shrinkage due to thick subcutaneous fat, severely debilitated fatty liver, or liver atrophy, resulting in incomplete thermal ablation. Incomplete thermal ablation of the target tumor could sufficiently promote residual tumor cells to induce accelerated growth of the distant tumor and hinder the efficacy of anti-PD-1 therapy [Citation37, Citation38]. The ablation can also be performed under CT or MRI guidance [Citation39]. Like fusion imaging, contrast-enhanced ultrasound, CT and MRI improve the performance of RFA in tumor localization and targeting. Nevertheless, they are relatively expensive, time-consuming and not available for all patients.
Indocyanine green fluorescence imaging based on the principle that ICG emits green fluorescence with a peak wavelength of 835 nm when illuminated with near-infrared light [Citation40]. These wavelengths are important, because they enable penetration of tissues at a 5–10 mm thickness. Previous study reported that fluorescence imaging identified 273 of 276 HCC lesions, demonstrating sensitivity of 99% [Citation41]. In this study, ICG-fluorescence imaging technique identified 33 of the total 40 lesions and the sensitivity was 82.5%. We infer that the difference might be related to the procedure of ICG-Fluorescence imaging. They sliced surgical specimens into 1 cm sections and then the cut surfaces of lesions were investigated using the ICG-fluorescence imaging. Therefore, the deep location lesions would be directly exposed to near-infrared light. Furthermore, the present study determined that ICG-Fluorescence imaging used in liver surface or in the cut surface of parenchyma (within 8 mm) was significantly higher than that in the deeply located hepatic parenchyma (96.4% vs 50.0%, p = 0.005). The distance between the lesion and the liver surface is an important factor affecting the sensitivity of ICG-fluorescence imaging. Tumor size and the cirrhosis of liver have no significant effect on it (p = 0.735, p = 0.281). Previous studies have reported that tumor detection rate using ultrasound in laparoscopic surgery or laparotomy increased by 30% compared with preoperative imaging [Citation42, Citation43]. For patients with local recurrence after ablation, ICG helps to find tumors around the ablation zone. In this study, a combination of intraoperative ultrasound and ICG- fluorescence imaging technique detected all the lesions. False positives can occur with ICG-fluorescence imaging due to factors such as cirrhotic liver nodules, hepatic cysts, and hyperplastic bile ducts that are capable of ICG uptake. To address this concern, a comprehensive reassessment of radiological data could be conducted to determine if the identified suspicious lesions might have been overlooked in preoperative imaging. If the location and size of the suspicious nodules closely align with those of tumors identified preoperatively, direct ablation could be performed. For instances where suspicious nodules are confirmed not to have been detected in preoperative imaging, an intraoperative percutaneous biopsy could be conducted. This additional step would ensure a thorough evaluation and provide valuable insights for guiding further treatment decisions.
In treating liver cancer, the efficacy of ICG-guided ablation therapy still needs to be further studied. Therefore, we retrospectively analyzed FLMWA and FLRFA for the treatment of both primary and metastatic liver tumors in our centers. The technical success rate was 100% and the technical effectiveness in this study was 92.5%, which is similar to previous studies [Citation44]. There were no serious grade 3, 4 AEs during FLRFA and FLMWA treatment. All the patients with side-effects recovered soon after procedures. We considered that the procedure offers greater flexibility as electrodes could be inserted at optimal angles and the liver could be moved when needed. This flexibility allowed for precise targeting of the tumor and minimizes the risk of injuring adjacent structures, such as large vessels, extrahepatic organs, or the liver capsule.
To increase the technical safety and efficacy of thermal ablation, ICG-Fluorescence imaging has shown improvement via several ways. 1) Clearly defined the boundary of the tumors; 2) To identify occult tumors and small satellite nodules not detected by preoperative imaging examination after performing a whole-liver fluorescence imaging examination prior to ablation therapy.
There were also some limitations in our study. First, it was a retrospective study with a small sample, short-term follow-up and lack of controls. Because fluorescent laparoscopy is not widely used in clinic, it is difficult to conduct large-scale randomized controlled studies and we were unable to establish the most significant boundary through rigorous mathematical modeling and statistically determine whether a depth of 0.8 cm provided the most significant difference in sensitivity. Secondly, it should be noted that while ICG fluorescence imaging identified some additional lesions, these were not subjected to confirmatory pathological examination and these suspicious nodules were not included in the analysis. However, this retrospective study may provide a hypothesis for future studies on the role of FLRFA and FLMWA in tumor ablation technology. Future multicenter studies could be conducted to assess their advantages and disadvantages. The effectiveness of fluorescent laparoscopy on different types of liver cancer remains unknown.
In conclusion, ICG-fluorescence-guided laparoscopic thermal ablation is a safe and curative method for tumor treatment. ICG-Fluorescence imaging helps to localize subscapular tumors on the liver surface or within 8 mm of the parenchymal section and to obtain a minimal coagulation margin during thermal ablation. In additional, it shows great technical effectiveness in treating liver cancer.
Disclosure statement
There are no relevant conflicts of interest related to the submitted work.
Data availability statement
Raw date was generated in the Department of Surgery at the Second Affiliated Hospital of Zhejiang University and the Fourth Affiliated Hospital of Zhejiang University. Derived date supporting the findings of this study are available from the corresponding author Zhe Tang on request.
Additional information
Funding
References
- Llovet JM, Kelley RK, Villanueva A, et al. Hepatocellular carcinoma. Nat Rev Dis Primers. 2021;7(1):6. doi:10.1038/s41572-020-00240-3.
- Li X, Ramadori P, Pfister D, et al. The immunological and metabolic landscape in primary and metastatic liver cancer. Nat Rev Cancer. 2021;21(9):541–557. doi:10.1038/s41568-021-00383-9.
- Steeg PS. Tumor metastasis: mechanistic insights and clinical challenges. Nat Med. 2006;12(8):895–904. doi:10.1038/nm1469.
- Milette S, Sicklick JK, Lowy AM, et al. Molecular pathways: targeting the microenvironment of liver metastases. Clin Cancer Res. 2017;23(21):6390–6399. doi:10.1158/1078-0432.CCR-15-1636.
- De Toni EN, Schlesinger-Raab A, Fuchs M, et al. Age independent survival benefit for patients with hepatocellular carcinoma (HCC) without metastases at diagnosis: a population-based study. Gut. 2020;69(1):168–176. doi:10.1136/gutjnl-2018-318193.
- Llovet JM, De Baere T, Kulik L, et al. Locoregional therapies in the era of molecular and immune treatments for hepatocellular carcinoma. Nat Rev Gastroenterol Hepatol. 2021;18(5):293–313. doi:10.1038/s41575-020-00395-0.
- Shin SW, Ahn KS, Kim SW, et al. Liver resection versus local ablation therapies for hepatocellular carcinoma within the milan criteria: a systematic review and meta-analysis. Ann Surg. 2021;273(4):656–666. doi:10.1097/SLA.0000000000004350.
- Lam VW, Ng KK, Chok KS, et al. Incomplete ablation after radiofrequency ablation of hepatocellular carcinoma: analysis of risk factors and prognostic factors. Ann Surg Oncol. 2008;15(3):782–790. doi:10.1245/s10434-007-9733-9.
- Teratani T, Yoshida H, Shiina S, et al. Radiofrequency ablation for hepatocellular carcinoma in so-called high-risk locations. Hepatology. 2006;43(5):1101–1108. doi:10.1002/hep.21164.
- Lu DS, Raman SS, Limanond P, et al. Influence of large peritumoral vessels on outcome of radiofrequency ablation of liver tumors. J Vasc Interv Radiol. 2003;14(10):1267–1274. doi:10.1097/01.rvi.0000092666.72261.6b.
- Zorbas G, Samaras T. A study of the sink effect by blood vessels in radiofrequency ablation. Comput Biol Med. 2015;57:182–186. doi:10.1016/j.compbiomed.2014.12.014.
- Kim JW, Shin SS, Heo SH, et al. Ultrasound-Guided percutaneous radiofrequency ablation of liver tumors: how We do it safely and completely. Korean J Radiol. 2015;16(6):1226–1239. doi:10.3348/kjr.2015.16.6.1226.
- Lee S, Kang TW, Song KD, et al. Effect of microvascular invasion risk on early recurrence of hepatocellular carcinoma after surgery and radiofrequency ablation. Ann Surg. 2021;273(3):564–571. doi:10.1097/SLA.0000000000003268.
- Jiang Q, Zeng Y, Xu Y, et al. Ultrasound molecular imaging as a potential non-invasive diagnosis to detect the margin of hepatocarcinoma via CSF-1R targeting. Front Bioeng Biotechnol. 2020;8:783. doi:10.3389/fbioe.2020.00783.
- Lauwerends LJ, van Driel P, Baatenburg de Jong RJ, et al. Real-time fluorescence imaging in intraoperative decision making for cancer surgery. Lancet Oncol. 2021;22(5):e186–e195. doi:10.1016/S1470-2045(20)30600-8.
- Fan Z, Zong J, Lau WY, et al. Indocyanine green and its nanosynthetic particles for the diagnosis and treatment of hepatocellular carcinoma. Am J Transl Res. 2020;12(6):2344–2352.
- Ishizawa T, Fukushima N, Shibahara J, et al. Real-time identification of liver cancers by using indocyanine green fluorescent imaging. Cancer. 2009;115(11):2491–2504. doi:10.1002/cncr.24291.
- Aoki T, Yasuda D, Shimizu Y, et al. Image-guided liver mapping using fluorescence navigation system with indocyanine green for anatomical hepatic resection. World J Surg. 2008;32(8):1763–1767. doi:10.1007/s00268-008-9620-y.
- Ishizawa T, Zuker NB, Kokudo N, et al. Positive and negative staining of hepatic segments by use of fluorescent imaging techniques during laparoscopic hepatectomy. Arch Surg. 2012;147(4):393–394. doi:10.1001/archsurg.2012.59.
- Wang X, Teh CSC, Ishizawa T, et al. Consensus guidelines for the use of fluorescence imaging in hepatobiliary surgery. Ann Surg. 2021;274(1):97–106. doi:10.1097/SLA.0000000000004718.
- Alfano MS, Molfino S, Benedicenti S, et al. Intraoperative ICG-based imaging of liver neoplasms: a simple yet powerful tool. Preliminary results. Surg Endosc. 2019;33(1):126–134. doi:10.1007/s00464-018-6282-1.
- Yokoyama N, Otani T, Hashidate H, et al. Real-time detection of hepatic micrometastases from pancreatic cancer by intraoperative fluorescence imaging: preliminary results of a prospective study. Cancer. 2012;118(11):2813–2819. doi:10.1002/cncr.26594.
- Tummers QR, Verbeek FP, Prevoo HA, et al. First experience on laparoscopic near-infrared fluorescence imaging of hepatic uveal melanoma metastases using indocyanine green. Surg Innov. 2015;22(1):20–25. doi:10.1177/1553350614535857.
- Kokudo N, Takemura N, Hasegawa K, et al. Clinical practice guidelines for hepatocellular carcinoma: the Japan society of hepatology 2017 (4th JSH-HCC guidelines) 2019 update. Hepatol Res. 2019;49(10):1109–1113. doi:10.1111/hepr.13411.
- Singal AG, Llovet JM, Yarchoan M, et al. AASLD practice guidance on prevention, diagnosis, and treatment of hepatocellular carcinoma. Hepatology. 2023;78(6):1922–1965. doi:10.1097/HEP.0000000000000466.
- Siriwardena AK, Serrablo A, Fretland AA, et al. Abdominal radiology, C.; interventional radiology society of Europe consensus on colorectal cancer with synchronous liver, m., multisocietal european consensus on the terminology, diagnosis, and management of patients with synchronous colorectal cancer and liver metastases: an E-AHPBA consensus in partnership with ESSO, ESCP, ESGAR, and CIRSE. Br J Surg. 2023;110(9):1161–1170. doi:10.1093/bjs/znad124.
- Hocquelet A, Aubé C, Rode A, et al. Comparison of no-touch multi-bipolar vs. monopolar radiofrequency ablation for small HCC. J Hepatol. 2017;66(1):67–74. doi:10.1016/j.jhep.2016.07.010.
- Ahn SJ, Lee JM, Lee DH, et al. Real-time US-CT/MR fusion imaging for percutaneous radiofrequency ablation of hepatocellular carcinoma. J Hepatol. 2017;66(2):347–354. doi:10.1016/j.jhep.2016.09.003.
- Xu M, Xu D, Deng Z, et al. Long-term outcomes of endoscopic ultrasound-guided laser ablation for liver tumors in the caudate lobe: 5 years of experience. Scand J Gastroenterol. 2023;58(5):558–564. doi:10.1080/00365521.2022.2148833.
- Meng YF, Jiang BB, Yan K, et al. Evaluation of the safety and efficacy of ultrasound-guided percutaneous radiofrequency ablation for hepatocellular carcinoma and liver metastases adjacent to the gallbladder. International Journal of Hyperthermia. 2023;40(1):2182748. doi:10.1080/02656736.2023.2182748.
- Fuchs CS, Doi T, Jang RW, et al. Safety and efficacy of pembrolizumab monotherapy in patients with previously treated advanced gastric and gastroesophageal junction cancer: phase 2 clinical KEYNOTE-059 trial. JAMA Oncol. 2018;4(5):e180013. doi:10.1001/jamaoncol.2018.0013.
- Kim N, Cheng J, Jung I, et al. Stereotactic body radiation therapy vs. radiofrequency ablation in asian patients with hepatocellular carcinoma. J Hepatol. 2020;73(1):121–129. doi:10.1016/j.jhep.2020.03.005.
- Vietti Violi N, Duran R, Guiu B, et al. Efficacy of microwave ablation versus radiofrequency ablation for the treatment of hepatocellular carcinoma in patients with chronic liver disease: a randomised controlled phase 2 trial. Lancet Gastroenterol Hepatol. 2018;3(5):317–325. doi:10.1016/S2468-1253(18)30029-3.
- Widmann G, Bodner G, Bale R. Tumour ablation: technical aspects. Cancer Imaging. 2009;9 Spec No A(Special issue A):S63–S7. doi:10.1102/1470-7330.2009.9026.
- Mulier S, Ni Y, Jamart J, et al. Local recurrence after hepatic radiofrequency coagulation: multivariate meta-analysis and review of contributing factors. Ann Surg. 2005;242(2):158–171. doi:10.1097/01.sla.0000171032.99149.fe.
- Xu EJ, Lv SM, Li K, et al. Immediate evaluation and guidance of liver cancer thermal ablation by three-dimensional ultrasound/contrast-enhanced ultrasound fusion imaging. Int J Hyperthermia. 2018;34(6):870–876. doi:10.1080/02656736.2017.1373306.
- Shi L, Wang J, Ding N, et al. Inflammation induced by incomplete radiofrequency ablation accelerates tumor progression and hinders PD-1 immunotherapy. Nat Commun. 2019;10(1):5421. doi:10.1038/s41467-019-13204-3.
- Markezana A, Goldberg SN, Kumar G, et al. Incomplete thermal ablation of tumors promotes increased tumorigenesis. Int J Hyperthermia. 2021;38(1):263–272. doi:10.1080/02656736.2021.1887942.
- Lee MW. Fusion imaging of real-time ultrasonography with CT or MRI for hepatic intervention. Ultrasonography. 2014;33(4):227–239. doi:10.14366/usg.14021.
- Zhou J, Fan X, Wu D, et al. Hot-band absorption of indocyanine green for advanced anti-stokes fluorescence bioimaging. Light Sci Appl. 2021;10(1):182. doi:10.1038/s41377-021-00627-1.
- Ishizawa T, Masuda K, Urano Y, et al. Mechanistic background and clinical applications of indocyanine green fluorescence imaging of hepatocellular carcinoma. Ann Surg Oncol. 2014;21(2):440–448. doi:10.1245/s10434-013-3360-4.
- Chung MH, Wood TF, Tsioulias GJ, et al. Laparoscopic radiofrequency ablation of unresectable hepatic malignancies. A phase 2 trial. Surg Endosc. 2001;15(9):1020–1026. doi:10.1007/s00464-001-0026-2.
- Wood TF, Rose DM, Chung M, et al. Radiofrequency ablation of 231 unresectable hepatic tumors: indications, limitations, and complications. Ann Surg Oncol. 2000;7(8):593–600. doi:10.1007/BF02725339.
- Meng Y, Jiang B, Yan K, et al. Evaluation of the safety and efficacy of ultrasound-guided percutaneous radiofrequency ablation for hepatocellular carcinoma and liver metastases adjacent to the gallbladder. Int J Hyperthermia. 2023;40(1):2182748.