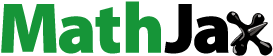
ABSTRACT
The mesomorphism is reported of a discotic complex of gold(III) that is amphiphilic on account of the presence of both hydrocarbon and fluorocarbon chains. The all-hydrocarbon analogue of the complex shows only a columnar hexagonal phase between the melting and clearing points, whereas this new, amphiphilic example shows a nematic phase between a columnar rectangular phase and a columnar hexagonal phase. The nature and formation of the nematic phase are discussed and it is proposed to be a columnar nematic formed as a result of frustration driven by the amphiphilic nature of the complex.
Graphical abstract
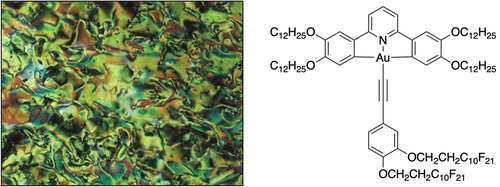
Introduction
As part of ongoing studies of metallomesogens capable of phosphorescence [Citation1–7], we recently reported on the mesomorphism, photophysics and device properties of some square-planar complexes of gold(III) () [Citation8]. These complexes showed exclusively Colh phases where X=OC12H25, whereas when X=H some derivatives showed either a Colr phase or a Colh phase or, in some examples, both. All complexes were phosphorescent and while those with X=H showed low quantum yields for emission (1.6–3.6%) in the green part of the spectrum (ca 500 nm), those with X=OC12H25 showed quantum yields up to 36% in the yellow part of the spectrum (ca 550 nm). The best external quantum efficiencies (EQE) in devices were just above 7%.
Figure 1. Liquid-crystalline complexes of gold(III) with phosphorescent properties; R1=CnH2n+1, R2, R3=H; or R1=OCnH2n+1, R2, R3=H or R1=R2=OCnH2n+1, R3=H or R1=R2=R3=OCnH2n+1.
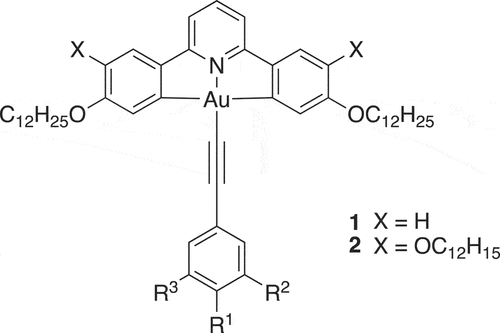
As a development of these initial studies, we were interested in the extent to which control could be exerted over the way in which these complexes organised in the columnar phase and whether, inter alia, this might impact ultimately on photophysical and/or device response in the condensed phase. In order to do this, the complexes were modified so that one or more of R1 to R3 was a semiperfluorinated chain of the formula OCH2CH2CmF2m+1 where m = 6, 8 or 10. The comprehensive study of these materials in terms of mesomorphism and photophysics is beyond the scope of this paper, in which we focus on a single derivative, 3, shown in .
Results
Synthesis
Complex 3 was prepared by the copper-promoted coupling of the corresponding chlorogold(III) complex with a terminal alkyne in a manner identical to that employed for complexes 2. However, the synthesis of the appropriate 3,4-disubstituted phenylalkyne requires description.
Thus, while 1-iodo(1H,1H,2H,2H-perfluoroalkane)s are available commercially, they can have a tendency to undergo elimination side-reactions under Williamson ether alkylation conditions to form alkenes, which can lead to poor yields of the desired alkylated product. However, the hydroxy equivalents, 1H,1H,2H,2H-perfluoroalkan-1-ols, are also available commercially, and can easily be transformed into the related triflates by reaction with triflic anhydride (). The poor solubility of 1H,1H,2H,2H-perfluorododecan-1-ol in common organic solvents led to the reaction being carried in a mixture of 1,4-dioxane and trifluoromethylbenzene.
Figure 2. Synthetic procedure to form 1H,1H,2H,-2H-perfluorododecyl trifluoromethanesulfonate. Conditions: (a) triflic anhydride (Tf2O), pyridine (in dioxane/PhCF3), 0 °C, 1 h, N2.

The required substituted phenylacetylene was then obtained in three steps as outlined in . Thus, the chains were coupled to the ring in 79% yield using a Williamson ether methodology, after which a Corey-Fuchs protocol was used to first obtain the 1,1-dibromovinyl compound (94%) and then the acetylene (64%). The final complex was determined to be pure by elemental analysis and was characterised further by 1H and 19F NMR spectroscopy.
Mesomorphism
Microscopy: On heating, complex 3 melts at 88.4°C to form a mesophase, which undergoes a phase transition at 93.5°C to form a second mesophase. On the basis of the molecular structure, these were assumed at this stage to be columnar in nature. Then at 139.4°C, a more fluid phase forms which persists to 156.1°C at which temperature a more ordered phase forms once more (again assumed columnar) which remains until clearing at 201.3°C. The phase sequence is reversed on cooling and, in addition, a further, monotropic phase is found below 76.4°C. The optical textures are shown in while thermodynamic data are found in , noting that two transitions are not observed by DSC. As can be seen in , the fluid phase has a brightly coloured, somewhat marbled texture and the properties suggest a nematic phase which, given its position between two columnar phases, is very surprising.
Figure 4. (Colour online) Photomicrographs of complex 3 on cooling from the isotropic liquid, showing (a) Colh (188.3°C); (b) N (156.4°C); (c) Colr2 (142.0°C); (d) Colr1 (90.9°C); (e) monotropic Colr3 (70.9°C). Reproduced with permission from the copyright holder at the University of York.
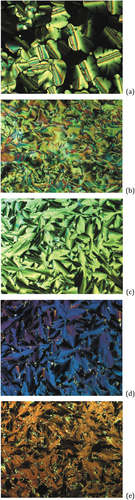
Table 1. Thermal data for compound 3.
X-Ray Scattering: SAXS experiments were carried out to provide structural information and phase identification. Thus, data in the highest-temperature phase confirm its identity as Colh with reflections () corresponding to d(10), d(11) and d(20) giving an intercolumnar distance a = 32.4 Å (). Below the Colh phase is the fluid phase identified as nematic on the basis of its optical texture and the SAXS data were consistent with this assignment. Thus, there is a broadish reflection at 2θ = 3.24° corresponding to a distance of 27.2 Å with a weak reflection at wider angle (ca 15° 2θ ≈ 5.9 Å) corresponding to spacings between mobile fluorocarbon chains ().
Table 2. X-ray diffraction data for complex 3.
Below the nematic phase are three columnar phases, one of which is monotropic. Both of the enantiotropic phases have similar patterns (), showing three reflections between 2θ = 1–2°, a very strong reflection at around 3° and then a series of much weaker reflections at higher angle out to about 2θ = 6° or so. In Colr2, the reflections index well for a centred orthorhombic lattice with large spacings in 3D: a = 115.5 Å, b = 83.1 Å and c = 71.8 Å. Comparison with Colr1 shows that the reflections appear extremely similar but shifted to slightly smaller angles, which would correspond to a slightly expanded lattice with a = 121.8 Å, b = 88.2 Å and c = 79.7 Å. In contrast, the monotropic Colr3 phase appears much simpler and shows just two strong reflections () that are readily indexed as d(11) and d(20) of a 2D rectangular plane with a = 62.0 Å and b = 63.3 Å. The difference in ordering in these phases will be addressed below.
The nature of the nematic phase
To find a less ordered mesophase between two more ordered mesophases is uncommon. This is perhaps best known in so-called re-entrant phases [Citation9] where a typical set of transitions in calamitic materials might look like (on cooling):
In such a situation a nematic phase is formed first on cooling the isotropic liquid, giving way to a SmA phase which, on further cooling, reveals another nematic phase below which is a second SmA phase. Seen first in mixtures, the origin of this behaviour often relates to the existence of strong, longitudinal dipoles in the constituent molecules, which give rise to antiparallel molecular correlations. The extent of the molecular overlap is then found to be different in the two nematic phases (in this example) so that the effective molecular length is different in each. However, such a change in molecular associations is evidently not always necessary as, for example, we have observed a re-entrant nematic phase between a SmA and a SmC phase [Citation10,Citation11], as well as a re-entrant cubic phase [Citation12], both examples coming from stilbazole complexes of silver(I) where such changes in local organisation are at best unlikely.
However, such behaviour, particularly with regard to nematic phases, is much less common in disc-like materials. The phenomenon was observed by the Bordeaux group at the beginning of the 1980s in a series of related hexasubstituted truxenes (). Thus, they first reported [Citation13] the existence of a nematic ND phaseFootnote1 below two columnar phases in 4-n (n = 6, 7, 9) which they assigned tentatively as Colh and Colr (the former on the basis of its uniaxial nature) to give the following cooling sequence with the ND phase being enantiotropic only in 4–9:
Then they reported [Citation14] a longer-chain homologue, 4–14 in which they reported truly re-entrant columnar phases and a nematic phase once more out of sequence, finding on cooling:
It is noted that the two lower-temperature columnar phases are monotropic and that the ND phase may be monotropic or enantiotropic depending on the thermal cycling and rate of heating and cooling. This report was quickly followed [Citation15] by a description of the mesomorphism of the related material 5–11 in which both a columnar and a nematic phase are re-entrant:
with all phases being enantiotropic. An extensive study of the materials and the structure of their mesophases using X-ray methods was published a few years later [Citation16]. However, while allusions were made to different forms of aggregation by analogy with the behaviour of calamitic systems, there was no real model that was advanced to account for the observations. Interestingly, this study reported that it was possible to align the nematic phase (applied field of 1.7 T) and also that in 5–12, cybotactic groups were found in the nematic phase. No evidence for either has so far been found in complex 3.
Therefore, how might the observation of this nematic phase be understood and, indeed, sandwiched between two columnar phases, what might its nature be? First here it is noted that the phase appears stable thermodynamically inasmuch as it does not change when held at a temperature between 140°C and 156°C for multiple hours. Confocal microscopy also showed the phase to have a negative birefringence as expected for a phase composed of disc-like molecules.
Now considering the phase sequence in which the ND phase is observed, above it is a Colh phase which shows a reasonably well-developed 2D order (the d(11) and d(20) reflections are readily evident), while below it is a 3D, centred orthorhombic mesophase. Interestingly, DSC does not find the transition between the ND and Colh phase (although this is absolutely evident from microscopy), while the transition from ND to Colr2 shows an entropy change of only 7 J K–1 mol–1. Both data points imply that the re-organisation required is small. If the nematic phase is then truly a ND phase, then the transition from Colh to ND would require both a ‘decoupling’ of the hexagonal order of the columns and a ‘decoupling’ of the molecules within the columns themselves. Forming the Colr2 phase would require the reverse process with enhanced 3D order. The other possibility, of course, is that the phase is not in fact ND rather it is NCol and, were this to be the case, then transitions in and out of nematic phase would simply require a melting of the 2D lattice, no doubt accompanied by a reduction in correlation length along the column axis. Given the smaller degree of reorganisation required, it is tempting to assign the phase as NCol.
Discussion
In considering complex 3, it should be remembered that it is in fact amphiphilic as it contains what would otherwise be incompatible hydrocarbon and fluorocarbon chains. Previous work showed that in conventional polycatenar mesogens containing both hydrocarbon and fluorocarbon chains, their immiscibility was demonstrated by, for example, an effective doubling of the SmC layer spacing from 37 Å to 76 Å on changing from the all-hydrocarbon molecule 6 to the amphiphilic 7 (). Thus, the SmC phase of 7 is bilayer in nature with only hydrocarbon–hydrocarbon or fluorocarbon–fluorocarbon sub-layers [Citation17]. Similar effects have been observed more recently in the SmA phase of some triphilic ionic LCs [Citation18].
In our previous report concerning the discotic gold(III) complexes 2 [Citation8], in common with related studies [Citation19–21], we found evidence for aggregation of the complexes in solution using 1H NMR spectroscopy. The data revealed that the complexes associated in a ‘back-to-back’ manner, although there was no correlation between this observation and the organisation implied in the mesophase from consideration of the lattice parameter.
Variable-concentration 1H NMR spectra were then obtained for compound 3 and the results are shown in . Once more, the data show clearly that there is aggregation in solution and, in common with data for complex 2, show evidence of back-to-back organisation in solution (). Thus, the resonances for the hydrogen atoms on the pincer ligand (labelled a, b and c) change with concentration as do those for the O–CH2 hydrogens of the chains on the pincer. The resonances for the aromatic hydrogens of the phenylacetylene and the O–CH2 hydrogens of the semiperfluorocarbon chains, however, are unchanged.
Figure 12. (Colour online) Variable-concentration 1H NMR spectra for complex 3 (a) in the aromatic region and (b) in the region of the methylene chain carbons O–CH2.
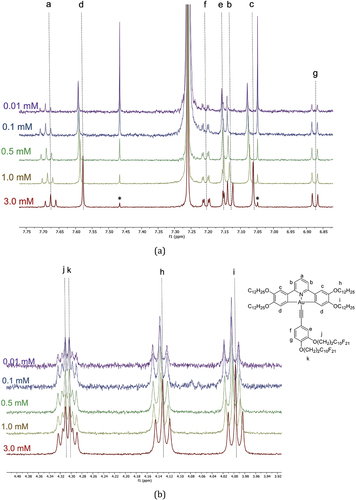
Figure 13. (Colour online) Proposed aggregate of 3 in concentrated solution showing a superposed dimer. Distances exaggerated for clarity. Fluorinated chains shown in green.
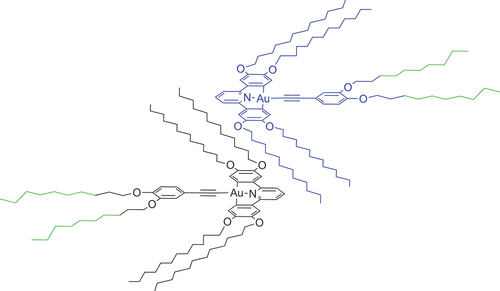
To try to understand the behaviour of 3, first a comparison is made with the behaviour of the parent, all-hydrocarbon analogue (monotropic Colr3 phase of 3 is omitted) [Citation8]:
Parent:
The first observation is that the clearing point is higher in 3 where there are fluorinated chains, something that is common in the behaviour of calamitic materials [Citation22]. Both complexes also have a Colh phase below the isotropic liquid and the intercolumnar distance for the two is all but identical at 31.4 Å for the parent and 32.4 Å for 3. This suggests that the presence of the two semiperfluorocarbon chains has no influence on the nature or organisation of the Colh phase in 3. Further, this distance rather closely matches the diameter of one molecule of complex, determined from the crystal structures reported in reference [Citation8] and the known length of carbon chains. The question then arises as to why this Colh phase in 3 does not simply persist down to the melting point.
One slightly curious observation in these complexes is the 3D nature of the Colr1 and Colr2 mesophases, implying organisation in the c direction and well as in the ab plane. To account for this, it is proposed that the origin of the 3D order is a correlation induced by a localised nanoscale organisation that would allow self-association of the fluorocarbon chains and indeed the diffraction patterns show a broad reflection around 16° 2θ that corresponds to a fluorocarbon–fluorocarbon spacing (ca 5.5 Å). The extent to which this organisation persists will depend on the balance of the different forces that determine the molecular arrangements, among which will be the relative magnitude of the dispersion forces promoting the organisation versus kT. Therefore, it can be considered that at the transition from Colr1 to what we propose as ColN, there is enough energy in the system so that 3D order of the phase, which it is proposed is related to effects of self-association of the fluorocarbon chains, can no longer be sustained. As indicated by the persistence of the ColN phase under isothermal conditions, this is not a kinetic phenomenon and so the structure that results maintains intracolumnar organisation but with uncorrelated columns – hence ColN. As the temperature increases further, so the thermal energy now overcomes totally the hydrocarbon/fluorocarbon immiscibility and so the disks of 3 become freer to orient allowing organisation into the observed Colh phase. This would be consistent with the observed similarity of the intercolumnar distance in the parent and 3, as in the former it was be expected that there is little if any barrier to molecular rotation about the columnar axis.
These observations lead to the proposal that the ColN phase is somehow a frustrated phase between a lower-temperature Colr phase where inter- and intra-columnar organisation is affected by the molecular amphiphilicity and the Colh phase where it is not. That the fluorocarbon/hydrocarbon immiscibility is overcome thermally is something we have previously likened to a consolution effect [Citation18]. The implications of this are as follows. Correlation of the fluorocarbon chains within the columns is implied for Colr1 and Colr2, and the fact that the rectangular lattice breaks apart at the transition to ColN implies also that the fluorocarbon chains have an influence on the 2D self-organisation. At minimum, therefore, the 2D orienting effect of the fluorocarbon chains is overcome thermally (loss of Colr phase) and is not seen in the higher-temperature phases (ColN and Colh). The fact that Colh is seen above ColN would then support the loss of a 2D-orienting capability of the fluorocarbon chains. This would then leave open the question of the extent of any specific intermolecular correlations within the columns.
Finally, in the light of the conclusions drawn above, it remains to revisit the truxene families of compounds and see the extent to which there may be any commonality in the explanations. As detailed earlier, there are different patterns for the observation of the nematic phase in these compounds and we would note that the idea of the ColN phase was not in common usage at the time these papers were published. Compound 4–14 has the overall phase behaviour most closely related to that of 3, but it is very difficult to see ways in which any of the arguments used for the latter could meaningfully be applied to the former. This does not rule out any notion of commonality and indeed such similar observations almost beg for a consistent and coherent explanation. As such, two concluding observations are drawn and it is the authors’ contention that BKS would have approved of both. First, we should make more compounds, in particular related to the gold complexes for which at the present time there is a single-point observation, and consider what other measurements may be attempted. Second, we should not confuse our inability to find a commonality of interpretation with its absence. It may seem as though detailed and systematic studies to clarify what may be smaller points of detail and understanding are hard to justify, yet through those systematic studies comes that true understanding that helps us to move the subject on. BKS knew this and his canon of publications shows an admirable blend of breadth and depth from which great insight arose. Indeed, his breadth of knowledge was staggering and Oh! how enlightening it would have been to discuss this problem with him, overlapping as it does with some of his major interests over the years – re-entrancy and frustration, metallomesogens and, of course, discotics. It is then with huge respect and great affection that we dedicate this paper to his memory.
Experimental
Equipment and techniques are as described previously [Citation8].
Synthesis of 1H,1H,2H,2H-perfluorodecyl trifluoromethanesulfonate
Triflic anhydride (5.1 cm3, 30.2 mmol) was taken into dry CH2Cl2 (30 cm3) and the solution degassed with N2 and cooled to 0°C. 1H,1H,2H,2H-perfluorodecan-1-ol (10.1 g, 21.8 mmol) and pyridine (1.73 cm3, 21.3 mmol) were added CH2Cl2:dioxane (20 cm3, 1:1) and the reaction mixture was stirred under a flow of N2 for 1 hour. The resulting precipitate was removed by filtration and volatiles removed from the filtrate in vacuo. The product was purified using 8:2 petroleum ether(40–60):ethyl acetate as eluent. 8.80 g. (68%).
1H NMR (400 MHz, CDCl3): δ = 4.77 (2H, t, 3JHH = 6.3 Hz), 2.67 (2H, m) ppm. 19F NMR (376 MHz, MeOD-d4): δ = −74.63 (3 F, s), −82.26 (3 F, t, 3JFF = 10.0 Hz), −114.3 (2F, m), −122.6 (2 F, m), −122.8 (4 F, m), −123.7 (2 F, m), −124.5 (2 F, m), −127.2 (2 F, m) ppm.
3,4-Bis(1H,1H,2H,2H-perfluoro(decyloxy))benzaldehyde
3,4-Dihydroxybenzaldehyde (781 mg, 5.70 mmol) and K2CO3 (1.72 g, 12.4 mmol) were dissolved in acetonitrile (20 cm3) and stirred at room temperature. 1H,1H,2H,2H-Perfluorodecyl trifluoromethanesulfonate (7.39 g, 12.4 mmol) was dissolved in acetonitrile (50 cm3) and added to the benzaldehyde solution. The resulting reaction mixture was stirred at room temperature for 16 hours, after which the precipitate which formed was isolated by filtration and washed with water and acetonitrile and air dried to give the product, which was used without further purification.
Yield = 5.14 g, 87%. 1H NMR (400 MHz, CDCl3): δ/ppm = 9.87 (1H, s), 7.52 (1H, dd, 3JHH = 8.2, 4JHH = 1.8), 7.45 (1H, d, 4JHH = 1.8), 7.02 (1H, d, 3JHH = 8.2), 4.38 (2H, t, 3JHH = 6.5 Hz), 4.36 (2H, t, 3JHH = 6.5 Hz), 2.69 (4H, m) ppm; 19F NMR δF (376 MHz, CDCl3): δ/ppm = – 80.7 (6F, t, 3JFF = 9.8 Hz), – 113.2 (4F, m), – 121.8 (4F, m), – 121.9 (8F, m), – 122.7 (4F, m), – 123.5 (4F, m), – 126.1 (4F, m).
(2,2-Dibromovinyl)-3,4-bis(1H,1H,2H,2H-perfluoro(decyloxy))benzene
Under a nitrogen atmosphere and cooled in an ice bath to 0°C, a solution of tetrabromomethane (1.88 g, 5.67 mmol) in CH2Cl2 (50 cm3) was added to a solution of triphenylphosphine (2.98 g, 11.4 mmol) in CH2Cl2 (20 cm3), with the temperature maintained below 15°C. After full addition, the mixture was cooled again to 0°C. A solution of 3,4-bis(1H,1H,2H,2H-perfluoro(decyloxy))benzaldehyde (4.52 g, 4.37 mmol) in CH2Cl2 (40 cm3)2 was added dropwise and the mixture stirred for 30 minutes. The mixture was then warmed to room temperature and stirred for 1 hour before being poured into hexane. The resulting precipitate was removed via filtration and the filtrate was concentrated in vacuo to give a residue which was purified by column chromatography on silica gel using CH2Cl2:petroleum ether(40–60) (1:1) as eluent to give the product.
Yield = 4.22 g, 81%. 1H NMR (400 MHz, CDCl3): δ/ppm = 7.40 (1H, s), 7.23 (1H, d, 4JHH = 2.0), 7.15 (1H, dd, 3JHH = 8.3, 4JHH = 2.0), 6.91 (1H, d, 3JHH = 8.3), 4.31 (2H, t, 3JHH = 6.6 Hz), 4.30 (2H, t, 3JHH = 6.6 Hz), 2.65 (4H, m); 19F NMR (376 MHz, CDCl3): δ/ppm = – 80.7 (6F, t, 3JFF = 9.8 Hz), −113.2 (4F, m), −121.8 (4 F, m), −121.9 (8F, m), −122.7 (4F, m), −123.5 (4F, m), −126.1 (4F, m).
1,2,-Bis(1H,1H,2H,2H-perfluoro(decyloxy))-4-ethynylbenzene
3,4-Bis(2,2-dibromovinyl)-1-(1H,1H,2H,2H-perfluoro(decyloxy))benzene (4.02 g, 3.37 mmol) was dissolved in THF (50 cm3) under an atmosphere of dinitrogen. A solution of EtMgBr (3 M in diethyl ether, 2.2 cm3, 6.62 mmol) was added dropwise, with stirring at room temperature, and the resulting reaction mixture was stirred for 2 h. Solid NH4Cl (excess) was then added to quench the reaction. Excess and resultant salts were removed via filtration, and the filtrate concentrated in vacuo. The residue was purified by column chromatography on silica gel using CH2Cl2:petroleum ether(40–60) (3:2) as the eluent.
Yield = 1.97 g, 57%. 1H NMR (400 MHz, CDCl3): δ/ppm = 7.15 (1H, dd, 3JHH = 8.4, 4JHH = 2.0), 7.05 (1H, d, 4JHH = 2.0), 6.86 (1H, d, 3JHH = 8.5), 4.30 (2H, t, 3JHH = 6.6 Hz), 4.28 (2H, t, 3JHH = 6.6 Hz), 3.03 (1H, s), 2.65 (4H, m); 19F NMR (376 MHz, CDCl3): δ/ppm = – 80.7 (6F, t, 3JFF = 9.8 Hz), −113.2 (4F, m), −121.8 (4F, m), −121.9 (8F, m), −122.7 (4F, m), −123.5 (4F, m), −126.1 (4F, m); CHN elemental analysis: observed (calculated): %C 32.6 (32.8), %H 1.2 (1.2).
Complex 3
The [Au(C^N^C)Cl] precursor (91 mg, 80.2 μmol) and CuI (10 mol%) were added to a 3-necked flask which was placed under N2. Dry, degassed dichloromethane (40 cm3) was added, followed by 3,4-bis(1H,1H,2H,2H-perfluorodecyloxy)ethynylbenzene (138 mg, 114 μmol) and triethylamine (45 mol%). The reaction mixture was stirred at reflux for 5 h under a N2 atmosphere, after which the reaction mixture was filtered through a pad of Celite and the solvent was removed in vacuo. The residue was purified by flash chromatography on silica gel using petroleum ether/CH2Cl2 (initially 7:3, followed by 1:1) as eluent and subsequently crystallised from hexane.
Yield = 110 mg, 61%. 1H NMR (400 MHz, CDCl3): δ = 7.68 (1H, t, 3JHH = 8.0 Hz), 7.58 (2H, s), 7.21 (1H, dd, 3JHH = 8.1 Hz, 4JHH = 1.8 Hz), 7.15 (1H, d, 4JHH = 2.0 Hz), 7.13 (2H, d, 3JHH = 8.5 Hz), 7.06 (2H, s), 6.87 (2H, d, 3JHH = 8.2 Hz), 4.31 (2H, t, 3JHH = 6.6 Hz), 4.30 (2H, t, 3JHH = 6.6 Hz), 4.13 (4H, t, 3JHH = 6.6 Hz), 4.00 (4H, t, 3JHH = 6.6 Hz), 2.66 (4H, m), 1.84 (8H, m), 1.47 (8H, m), 1.25 (64H, broad s), 0.87 (12H, m) ppm; 19F NMR (376 MHz, CDCl3): δ = – 80.7 (6F, m), −113.18 (4F, m), −121.7 (4F, m), −121.9 (12F‡, m), −122.8 (4F, m), −123.5 (4F, m), −126.1 (4F, m); MS m/z (APCI+): 2389.80 (M+H, calc. 2388.91), 2289.81 (M+H with 1x C10 chain), 2189.81 (M+H with 2x C10 chain, 15–10); CHN elemental analysis: observed (calculated): %C 49.7 (49.8), %H 5.1 (5.0), %N 0.5 (0.6).
Acknowledgments
We thank the University of York for funding (RRP and AJM), Johnson Matthey for generous loans of gold salts and Dr Stephen Cowling for recording some SAXS data.
Disclosure statement
No potential conflict of interest was reported by the authors.
Additional information
Funding
Notes
1. The term ND is used here for convenience to imply a nematic phase of disc-like molecules. In fact, a mesophase should be defined only by its own symmetry and not that of the molecules of which it is composed.
References
- Qian GW, Yang XF, Wang XB, et al. Chiral platinum-based metallomesogens with highly efficient circularly polarized electroluminescence in solution-processed organic light-emitting diodes. Adv Opt Mater. 2020;8:2000775.
- Parker RR, Sarju JP, Whitwood AC, et al. Synthesis, mesomorphism, and photophysics of 2,5-Bis(dodecyloxyphenyl)pyridine complexes of platinum(IV). Chem Eur J. 2018;24:19010–19023.
- Wang YF, Liao YW, Cabry CP, et al. Highly efficient blueish-green fluorescent OLEDs based on AIE liquid crystal molecules: from ingenious molecular design to multifunction materials. J Mater Chem C. 2017;5:3999–4008.
- Kumar NSS, Shafikov MZ, Whitwood AC, et al. Mesomorphism and photophysics of some metallomesogens based on hexasubstituted 2,2‘:6‘,2 “-terpyridines. Chem Eur J. 2016;22:8215–8233.
- Spencer M, Santoro A, Freeman GR, et al. Phosphorescent, liquid-crystalline complexes of platinum(II): influence of the beta-diketonate co-ligand on mesomorphism and emission properties. Dalton Trans. 2012;41:14244–14256.
- Prokhorov AM, Santoro A, Williams JAG, et al. Phosphorescent mesomorphic dyads based on tetraacetylethane complexes of iridium(III). Angew Chem Int Ed. 2012;51:95–98.
- Santoro A, Prokhorov AM, Kozhevnikov VN, et al. Emissive metallomesogens based on 2-phenylpyridine complexes of iridium(III). J Am Chem Soc. 2011;133:5248–5251.
- Parker RR, Liu DH, Yu XK, et al. Synthesis, mesomorphism, photophysics and device performance of liquid-crystalline pincer complexes of gold(III). J Mater Chem C. 2021;9:1287–1302.
- Cladis PE, Mandle RJ, Goodby JE. Re-entrant phase transitions in liquid crystals. In: Goodby JW, Collings PJ, Kato T, et al., editors. Handbook of liquid crystals, Vol. 2, Chpt. 16, 2nd. Wiley-VCH; Weinheim, 2014.
- Adams H, Bailey NA, Bruce DW, et al. Mesomorphic stilbazole complexes of silver octyl sulfate - Crystal and molecular-structure of Bis[4-(4-Methoxystyryl)Pyridinato]Silver(I) octyl sulfate hemihydrate. J Mater Chem. 1992;2:395–400.
- Bruce DW, Donnio B, Hudson SA, et al. X-ray diffraction from mesophases of some stilbazole complexes of Silver(I): monodomain determination of a thermotropic cubic phase. Journal De Physique II. 1995;5:289–302.
- Bruce DW, Hudson SA. Mesomorphic complexes of silver trifluoromethanesulfonate and silver dodecylsulfate with 2-Fluoro-4-alkoxystilbazoles and 3-Fluoro-4-alkoxystilbazoles. J Mater Chem. 1994;4:479–486.
- Destrade C, Malthete J, Tinh NH, et al. Truxene derivatives - Temperature inverted nematic-columnar sequence in disc-like mesogens. Phys Lett A. 1980;78:82–84.
- Tinh NH, Malthete J, Destrade C. Reentrant phenomenon in disc-like liquid-crystal. Mol Cryst Liq Cryst. 1981;64:291–298.
- Tinh NH, Malthete J, Destrade C. Reentrant nematic and columnar phases in disc-like liquid-crystals at atmospheric-pressure. J Phys Lett-Paris. 1981;42:L417–L419.
- Tinh NH, Foucher P, Destrade C, et al. Reentrant mesophases in disc-like liquid-crystals. Mol Cryst Liq Cryst. 1984;111:277–292.
- Gainar A, Tzeng MC, Heinrich B, et al. Incompatibility-driven self-organization in polycatenar liquid crystals bearing both hydrocarbon and fluorocarbon chains. J Phys Chem B. 2017;121:8817–8828.
- Riccobono A, Lazzara G, Rogers SE, et al. Synthesis and mesomorphism of related series of triphilic ionic liquid crystals based on 1,2,4-triazolium cations. J Mol Liq. 2021;321:114758.
- Yim KC, Au VKM, Hung LL, et al. Luminescent dinuclear bis-cyclometalated gold(III) alkynyls and their solvent-dependent morphologies through supramolecular self-assembly. Chem Eur J. 2016;22:16258–16270.
- Yim KC, Au VKM, Wong KMC, et al. Luminescent bis-cyclometalated gold(III) complexes with alkynyl ligands of hexaphenylbenzene and hexabenzocoronene derivatives and their supramolecular assembly. Chem Eur J. 2017;23:5772–5786.
- Yim KC, Lam ESH, Wong KMC, et al. Synthesis, characterization, self-assembly, gelation, morphology and computational studies of alkynylgold(III) complexes of 2,6-Bis(benzimidazol-2 ‘-yl)pyridine derivatives. Chem Eur J. 2014;20:9930–9939.
- Guittard F, Taffin de Givenchy E, Geribaldi S, et al. Highly fluorinated thermotropic liquid crystals: an update. J Fluorine Chem. 1999;100:85–96.