ABSTRACT
The synthesis and characterisation of two groups of nonsymmetric dimers, the 1-(4-cyanobiphenyl-4‘−yloxy)-ω-(4-butyl, 4-(1-methylpropyl) or 4-t-butylanilinebenzylidene-4’−oxy)alkanes, and the 1-(4-cyanobiphenyl-4‘−yl)-ω-(4-butyl, 4-(1-methylpropyl) or 4-t-butylanilinebenzylidene-4’−oxy)alkanes, are reported. The length and parity of the flexible spacer is varied. The tert-butyl homologues show higher melting points than the corresponding sec-butyl or n-butyl substituted dimers, suggesting that chain branching improves packing efficiency within the crystalline structure. The branched chain homologues have a stronger tendency to exhibit smectic phases than the n-butyl-substituted dimers, and for longer spacers are exclusively smectic. A comparison of the nematic-isotropic transition temperatures (TNI) for dimers containing the different terminal chains is possible for one set of materials, and reveals a large reduction in TNI on passing from the n-butyl to sec-butyl-substituted, but a much smaller decrease on changing sec-butyl for tert-butyl. A different trend is observed for the smectic A-isotropic transition temperatures for which the tert-butyl substituted dimers show a higher value than the corresponding sec-butyl homologue, and only marginally lower than that of the n-butyl-substituted dimer. This surprising behaviour is interpreted in terms of the ability of the tert-butyl group to pack more efficiently into the intercalated smectic A phase as the spacer length increases.
GRAPHICAL ABSTRACT
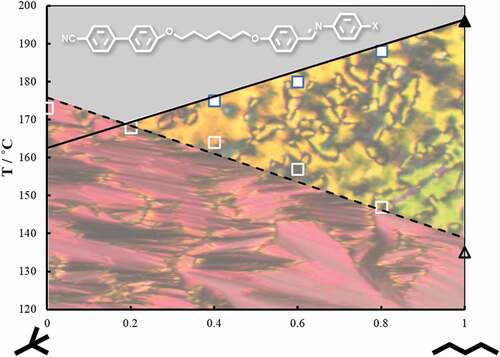
Acknowledgements
EG and DP thank the National Science Centre (Poland) for financial support under the grant no. 2016/22/A/ST5/00319. RW gratefully acknowledges The Carnegie Trust for the Universities of Scotland for funding the award of a PhD scholarship 2015–2018
Disclosure statement
No potential conflict of interest was reported by the author(s).