Abstract
Background: The ability to communicate using speech is a remarkable skill, which requires precise coordination of articulatory movements and decoding of complex acoustic signals. According to the traditional view, speech production and perception rely on motor and auditory brain areas, respectively. However, there is growing evidence that auditory-motor circuits support both speech production and perception.
Aims: In this article we provide a review of how transcranial magnetic stimulation (TMS) has been used to investigate the excitability of the motor system during listening to speech and the contribution of the motor system to performance in various speech perception tasks. We also discuss how TMS can be used in combination with brain-imaging techniques to study interactions between motor and auditory systems during speech perception.
Main contribution: TMS has proven to be a powerful tool to investigate the role of the articulatory motor system in speech perception.
Conclusions: TMS studies have provided support for the view that the motor structures that control the movements of the articulators contribute not only to speech production but also to speech perception.
The human auditory system plays a critical role in processing acoustic speech signals. Evidently it also supports speech production. Monitoring of one's own speech, i.e., the auditory consequences of one's own articulatory movements, is an essential aspect of speech production. For example, alterations of auditory feedback during speaking can affect motor control of articulatory movements (Houde & Jordan, Citation1998). Reactivity of the auditory regions in the superior temporal cortex is modified during articulation, presumably via efference copy mechanisms (Curio, Neuloh, Numminen, Jousmäki, & Hari, Citation2000; Numminen & Curio, Citation1999). Also, perception of acoustic speech signals is altered during execution of articulatory movements (Sams, Möttönen, & Sihvonen, Citation2005). Thus it is uncontroversial that the auditory system interacts with the articulatory motor system during speech production. The idea that neural processing of acoustic speech signals produced by someone else would also rely on interacting auditory and motor systems is more controversial and the topic is under active debate among speech scientists (Hickok, Citation2010; Hickok, Houde, & Rong, Citation2011; Lotto, Hickok, & Holt, Citation2009; Pulvermuller & Fadiga, Citation2010; Rauschecker & Scott, Citation2009; Scott, McGettigan, & Eisner, Citation2009; Wilson, Citation2009).
A key question in speech perception research is how phonetic categories are extracted from acoustic speech signals. How is a listener able to perceive that a speaker said the word “cat” rather than, for example, “bat” or “cap”? And how is it possible that auditory tokens of “cat” produced by different speakers are perceived so similarly? Speech comprehension is a challenging task, because there is no one-to-one relationship between phonetic categories and acoustic features of speech sounds and the speech signals produced by different speakers in different contexts are highly variable. The speakers' articulatory gestures are somewhat less variable. For example, all speakers produce the word “cat” by first closing the vocal tract by moving the root of the tongue (“c”), then keeping the vocal tract open (“a”) and finally closing it by moving the tip of the tongue (“t”). Generation of internal motor models of the speaker's articulatory movements could possibly facilitate speech comprehension, since there are physical constraints on how articulators can move during speech production. In other words, speech perception could (in addition to auditory processes) engage complementary motor processes that are involved in solving an inverse problem: Which articulatory movements could have produced a given acoustic speech signal?
The idea that the speech production system would be involved in speech perception is not new. A central claim in Liberman's motor theory of speech perception is that the listener detects the speaker's “neuromotor commands to the articulators” (or “intended articulatory gestures”) from the acoustic signal (Galantucci, Fowler, & Turvey, Citation2006; Liberman, Cooper, Shankweiler, & Studdert-Kennedy, Citation1967; Liberman & Mattingly, Citation1985). The theory proposes that there is a one-to-one relationship between these neuromotor commands and the phonetic categories and, therefore, differing speech sounds can be mapped onto the same phonetic category. Thus, according to the motor theory, speech perception is inherently linked to speech production. However, most speech scientists have rejected this claim (and many other claims) of the motor theory; they consider speech perception to be a purely auditory process, not a motor or auditory-motor process (Diehl, Lotto, & Holt, Citation2004; Lotto et al., Citation2009). Moreover, neuroimaging studies of speech perception have consistently shown that speech processing specifically activates non-primary auditory regions in the superior temporal cortex, giving support for the view that the auditory system is critical for speech perception (e.g., Binder et al., Citation2000; Dehaene-Lambertz et al., Citation2005; Möttönen et al., Citation2006).
During the past decade, transcranial magnetic stimulation (TMS) has become a widely used method in cognitive neuroscience, because it can provide information that is complementary to neuroimaging. It has also provided speech scientists with new tools to investigate whether the motor system, not only the auditory system, could be involved in speech perception. Importantly, TMS has made it possible to test experimentally whether the neural circuits that control movements of the articulators are facilitated during listening to speech and whether these circuits contribute to speech perception. In this article we provide a review of studies that have used TMS to address these questions and discuss methodological and theoretical aspects of these studies. We also present new ideas of how TMS can be used in combination with other brain imaging techniques to investigate putative interaction between auditory and motor systems during speech perception.
USING TMS TO MEASURE MOTOR EXCITABILITY DURING SPEECH PERCEPTION
TMS activates cortical neuronal elements by electromagnetic induction. Single TMS pulses over the primary motor (M1) cortex elicit motor evoked potentials (MEPs) in the contralateral target muscles that can be measured using electromyography. The MEPs recorded from the hand muscles peak approximately 24 ms after the pulse, whereas MEPs recorded from the lip muscles typically peak approximately 10 ms after the pulse, because the pathway from the corticobulbar pathway to the lips is shorter that the corticospinal pathway to the hands. The TMS intensity that is required to elicit MEPs is highly variable across participants, most likely due to variability in the distance from the simulating coil to the cortical surface (Stokes et al., Citation2005). Moreover, within-participant variation in amplitudes of the MEPs is dependent on the functional state of the motor system. For example, TMS pulses of constant intensity elicit larger MEPs when the participant contracts the target muscle than when the muscle is relaxed. The MEP measurements provide an accurate tool to localise cortical representations of the different muscles in each participant and to normalise the TMS intensity across participants. Importantly, they also provide a direct measure (i.e., MEP amplitude) of the excitability of the motor pathways connecting muscles with their cortical representations.
Several studies have demonstrated that the amplitudes of MEPs recorded from the articulatory muscles increase during speech perception, suggesting that excitability of the motor representations of articulators is increased. Watkins, Strafella, and Paus (Citation2003) stimulated the lip representation in the left M1 cortex and showed that these MEPs were enhanced while participants were listening to continuous speech or viewing a talking face (see also Sundara, Namasivayam, & Chen, Citation2001). In contrast, MEPs elicited by the stimulation of the hand representation in the left M1 or the lip representation in the right M1 were unchanged during auditory and visual speech perception, indicating that the increased motor excitability was specific to the lip representation in the left hemisphere. Moreover, excitability of the lip representation was not increased, while participants watched random movements of the upper face, eyes, and brows. Interestingly, when participants listened to environmental sounds, excitability of the lip representation was also increased in the left hemisphere (relative to right hemisphere) and there was no significant difference between this condition and speech conditions. This suggests that the increased motor excitability in the left articulatory motor system may not be specific to speech. Watkins et al. (Citation2003) suggested that this increased excitability in the left M1 cortex was due to the fact that some environmental sounds could be imitated using the vocal tract (e.g., engine noise). In a similar way, listening to sounds that can be produced using hand actions (e.g., typing sounds) increases excitability in the hand area of the left M1 cortex (Aziz-Zadeh, Iacoboni, Zaidel, Wilson, & Mazziotta, 2010). Watkins et al. (Citation2003) did not report statistical tests between a baseline condition (listening to white noise) and other experimental conditions. We have re-analysed the original data reported previously to provide the statistics for the comparisons of each condition with baseline. Lip MEPs induced by left M1 stimulation during both speech conditions were significantly increased from baseline; listening to speech: t(7) = 2.18, p = .03; viewing speech-related lip movements: t(7) = 2.63, p = .02, whereas non-speech conditions did not differ from baseline; listening to environmental noise: t(7) = 1.46; viewing eye movements: t(7) = –0.19. We have also analysed data from a group of 13 participants (the original eight published and an additional five new participants) and found the same pattern (see ); paired t-test with baseline condition: listening to speech: t(12) = 2.33, p = .02; viewing lip movements: t(12) = 1.92, p = .04; listening to environmental noise: t(12) = 1.28; viewing eye movements: t(12) = –1.28. It should be noted, however, that the difference between listening to speech and listening to environmental noise was not significant in this group either.
Figure 1. MEP sizes elicited in the contralateral orbicularis oris muscle by single-pulse TMS over the lip representation of left M1. Bars show the mean (+ standard error; SEM) change in MEP size (mV.ms) from a baseline condition (in which participants viewed visual noise and listened to white noise) while participants (n = 13) were listening to speech (Speech, mean = 167.65, SEM = 70.62) or environmental sounds (Nonspeech, mean = 67.10, SEM = 43.86), or viewing speech-related lip movements (Lips, mean = 114.33, SEM = 57.82) or eye and brow movements (Eyes, mean = –28.14, SEM = 41.86). MEP sizes (area under the curve of the rectified electromyographic recording) were adjusted for background contraction of the lip muscle using analysis of covariance (for details see Watkins et al., Citation2003). A paired t-test was used to compare MEP areas in each of these conditions with those in the baseline. The MEPs for the Speech and Lips conditions were significantly increased relative to the baseline condition (*p < .05).
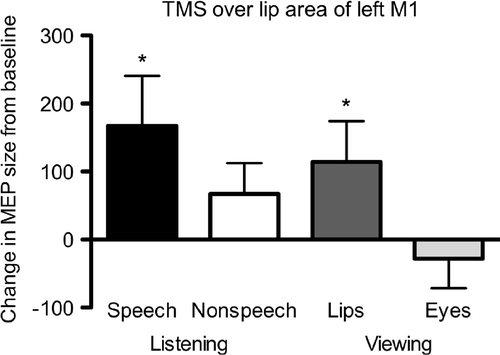
Recently, Murakami, Restle, and Ziemann (Citation2011) showed that decreasing intelligibility of auditory speech (by adding noise) and visual speech (by speeding up the articulatory movements) further enhanced the facilitation of the MEPs recorded from the lips. This suggests that the engagement of the articulatory motor system is increased when speech signals are difficult to understand. This finding is in agreement with an idea that the motor system is a complementary system, which is recruited especially in challenging conditions (Wilson, Citation2009). It should be noted, however, that in this recent study (Murakami et al., Citation2011) and in the earlier study (Watkins et al., Citation2003) a significant facilitation was also found during listening to speech signals that were easy to understand, indicating that articulatory motor system is engaged even in optimal listening conditions (and without behavioural tasks). Murakami et al. (Citation2011) also showed that listening to speech and viewing speech-related movements increased short-interval intracortical inhibition of lip MEPs using paired-pulse TMS. This may reflect inhibition of involuntary muscle activity during speech perception.
Fadiga, Craighero, Buccino, and Rizzolatti (Citation2002) examined whether the motor system is activated in an articulator-specific manner during speech perception. They stimulated the tongue representation in the left M1 while participants listened to Italian words that included phonemes that are articulated using the tongue (e.g., “r” in “birra”) and words that are not articulated using the tongue (e.g., “biffa”). The tongue MEPs were found to be enhanced more during listening to words requiring tongue movements in production, compared to listening to other words. Moreover, the MEP enhancement was stronger for words than for pseudowords. Importantly, the pulses were delivered only 100 ms after the onset of the critical middle phoneme in this study, suggesting that the motor system is involved in speech processing at an early stage. Later, Roy, Craighero, Fabbri-Destro, and Fadiga (Citation2008) replicated the earlier finding by showing that tongue MEPs elicited by pulses delivered 100 ms after the onset of the critical phoneme are sensitive to the articulatory features of the phonemes in pseudowords. The tongue MEPs elicited by pulses that were delivered later (200–300 ms after the onset) were sensitive to the frequency of the words (rare words caused stronger facilitation than frequent words), giving further support for the idea that lexical factors also affect the strength of motor excitability.
The increased size of MEPs elicited by stimulation of the cortical representation of the articulators reflects increased excitability in the motor pathways from the cortex to the brainstem nuclei innervating the lip and tongue muscles. Changes in excitability could therefore occur at either the cortical level in the upper motor neurons or at the level of the facial nucleus in the lower motor neurons. In addition, these changes in excitability could be brought about by inputs from other areas that are either cortical or subcortical. Paired-pulse TMS experiments over M1 representations have demonstrated muscle-specific modulation of intracortical facilitation and inhibition during observation of hand and arm movements (Strafella & Paus, Citation2000) and during auditory and visual perception of speech (Murakami et al., Citation2011). These findings support the view that the changes in excitability during action and speech perception occur at the cortical level rather than at the brainstem nuclei. The M1 cortex is not known to receive direct inputs from the visual or auditory cortex. It is thought that M1 cortical excitability is modulated instead by inputs from ventral premotor cortex (see Shimazu, Maier, Cerri, Kirkwood, & Lemon, Citation2004, for action observation in monkeys, and Cattaneo et al., Citation2005, for object perception prior to grasping in humans).Consistent with this suggestion, we found increased blood flow in ventral premotor cortex (Broca's area) correlated with motor excitability during speech perception in a combined positron emission tomography (PET) and TMS study (Watkins & Paus, Citation2004). Finally, the findings in speech perception studies that motor excitability is influenced by lexical factors (Fadiga et al., Citation2002, Roy et al., Citation2008) and is specific to the left hemisphere (Watkins et al., Citation2003) also point to a cortical origin for these effects, although additional subcortical influences cannot be ruled out.
Some functional MRI (fMRI) studies have also demonstrated that blood-oxygenation level dependent (BOLD) responses in the motor system are enhanced during listening to speech sounds (e.g., Callan, Callan, Gamez, Sato, & Kawato, Citation2010; Pulvermüller et al., Citation2006; Wilson, Saygin, Sereno, & Iacoboni, Citation2004). Increased BOLD responses in superior ventral premotor cortex are seen during listening to meaningless monosyllables, but this activity does not encompass primary motor cortex. Rather, it is localised to the border between primary motor (Brodmann area 4a) and premotor (Brodmann area 6) cortex (Wilson et al., Citation2004). Using region-of-interest (ROI) analyses, Pulvermüller and colleagues (Citation2006) showed that listening to lip- or tongue-related phonemes modulated activity in the precentral gyrus in an articulator-specific manner. A dorsal ROI defined by activation to lip movement was more active during perception of lip-related phonemes (“p”) than tongue-related phonemes (“t”), whereas a ventral ROI defined by activation to tongue movement showed the reverse pattern (Pulvermüller et al., Citation2006). Activity during speech perception in the primary motor cortex was not extensive and did not survive statistical thresholding in the whole-brain analysis. In a study comparing activations to an illusory percept “ta” created by the McGurk effect (combining auditory stimulus “pa” with visual stimulus “ka”) with auditory or visual syllables “pa”, “ta”, and “ka”, activation was found in the left precentral gyrus during listening to syllables (Skipper, van Wassenhove, Nusbaum, & Small, Citation2007). This activity was at the level of the lip representation and overlapped a region active in production of the same syllables.
It should be noted that many imaging studies using fMRI or PET, which have found enhanced activity in the temporal lobes during listening to speech, have failed to find such activity in the motor system. There are several possible explanations as to why imaging and MEP studies could have different sensitivities to speech processing in the motor system. First, there are likely to be individual differences in the somatotopic organisation and anatomy of the motor cortex (see Takai, Brown, & Liotti, Citation2010). Typically, in fMRI studies, brains of individual participants are registered into a standard space and the group-level statistical analyses are performed in this space. Therefore it is not surprising that the activations in the motor system do not reach statistical significance, if the individual representations of the articulatory organs (lip, tongue etc) do not overlap in the standard space (e.g., Pulvermüller et al., Citation2006). TMS studies avoid this problem by measuring MEPs from the same muscle in each participant (e.g., orbicularis oris for the lips) and separately localising the target regions in individuals. Second, the haemodynamic responses measured by PET and fMRI and motor excitability measured by TMS-induced MEPs may reflect different neural phenomena. This is supported by a recent fMRI study, which measured BOLD responses during anodal and cathodal transcranial direct current stimulation (tDCS) over the M1 cortex (Antal, Polania, Schmidt-Samoa, Dechent, & Paulus, Citation2011). Anodal tDCS increases and cathodal tDCS decreases motor excitability measured by TMS-induced MEPs from hand muscles (Nitsche & Paulus, Citation2000). However, neither anodal nor cathodal tDCS altered BOLD signals in the M1 cortex during rest (Antal et al., Citation2011). It should also be noted that in our combined TMS and PET study we did not find increased rCBF in the left M1 cortex during speech perception, even though simultaneously recorded lip MEPs were enhanced and there was no correlation between motor excitability and rCBF in the M1 cortex (Watkins & Paus, Citation2004). These studies demonstrate that haemodynamic responses are not necessarily increased when motor excitability is increased. Changes in motor excitability are thought to be caused by changes in the resting membrane potentials of motor neurons (Rothwell, Citation2008). These changes do not necessarily cause any changes in the level of spiking activity in the motor system. TMS-induced MEPs will, however, increase because a larger number of corticobulbar (or corticospinal) neurons are closer to the threshold and therefore fire (when the TMS pulse is delivered). The synchronisation of the TMS-induced motor neuron discharges or the number of repetitive discharges of individual neurons will also affect MEP size (Rösler & Magistris, Citation2008). Simply put, the changes in MEP size reflect changes in synaptic efficacy and membrane potentials of neuronal populations in the stimulated area due to excitatory or inhibitory inputs from other cortical areas (see above). The haemodynamic responses as measured by rCBF in PET or BOLD in fMRI reflect changes in energy consumption, but their underlying neuronal mechanisms are not fully understood. It is thought that they reflect both synaptic and spiking activity (see, e.g., Logothetis, Citation2002; Rosa, Kilner, & Penny, Citation2011). Possibly the neuronal mechanisms that increase the motor excitability do not consume enough energy in the M1 cortex to induce changes in haemodynamic responses.
In sum, recording of TMS-induced MEPs from articulatory muscles provides a powerful tool to study involvement of the motor system during speech perception. This method provides information that is complementary to fMRI and PET. To date, relatively few studies have used MEP measurements to study excitability of the articulatory motor regions during speech perception. This method has been more widely used to examine motor excitability changes in the hand area of the M1 cortex during action observation (e.g., Aziz-Zadeh et al., Citation2004; Fadiga, Fogassi, Pavesi, & Rizzolatti, Citation1995). In our opinion the full potential of this method has not been realised among speech scientists. For example, further studies using both speech and well-controlled non-speech signals are needed to clarify whether the excitability of the articulatory motor system is enhanced specifically during listening to speech or whether processing of some non-speech signals also engages this part of the motor system.
THE “VIRTUAL LESION” APPROACH: USING TMS TO DISRUPT SPEECH PERCEPTION
The single-pulse TMS studies demonstrate that the excitability of the articulatory motor system is increased during speech perception. They do not, however, indicate that the motor system contributes to speech perception. Therefore a number of recent studies have used TMS to induce temporary “virtual lesions” in the healthy human brain to address whether the motor system plays a causal role in speech perception.
Meister, Wilson, Deblieck, Wu, and Iacoboni (Citation2007) applied low-frequency repetitive TMS (rTMS) over the premotor cortex in order to induce a temporary disruption (i.e., a “virtual lesion”) in this area. After rTMS over the premotor cortex, participants were impaired at identifying syllables (“pa”, “ta”, and “ka”) that were presented in noise. In contrast, participants were unimpaired in the control tasks (colour and tone discrimination) after rTMS over the premotor cortex. These findings were the first to provide confirmatory evidence that the motor system plays a causal role in speech perception.
We have also used low-frequency rTMS to induce temporary disruptions in the motor system to examine the role of the motor representations of articulators in the discrimination of speech sounds (Möttönen & Watkins, Citation2009). We stimulated the lip representations in the left M1 cortex for 15 minutes with rTMS and showed MEPs recorded from the lips were suppressed after rTMS, indicating reduced motor excitability. This rTMS-induced disruption impaired the ability of the participants to discriminate synthetic speech sounds that are lip-articulated from speech sounds that are not articulated by the lips (“ba” vs “da” and “pa” vs “ta”, see ). However, this disruption did not influence their ability to discriminate two speech sounds that are not articulated by the lips (“ka” vs “ga” and “da” vs “ga”, see ). An rTMS-induced disruption in the hand area of the M1 cortex did not impair discrimination of any speech sounds. These findings support the view that the motor representations of articulators contribute to discrimination of speech sounds in an articulatory-feature-specific manner. Possibly the disruption of the motor lip representation inhibited the generation of internal motor models that include lip movements. Discrimination of speech sounds that belong to the same phonetic category (i.e., within-category pairs) was poorer than discrimination of across-category pairs, although the acoustic distance between the sounds was the same (). Enhanced discriminability of across-category pairs is a hallmark of categorical speech perception. The rTMS-induced disruption of the motor lip representation did not affect discrimination of any within-category pairs, which suggests that it relies completely on auditory mechanisms, not on motor or auditory-motor mechanisms.
Figure 2. The effect of TMS-induced disruption in the motor lip representation on discrimination of speech sounds. Participants were presented with synthetic speech sounds from eight-step acoustic continua between two speech sounds. The pairs of sounds were categorised as “across-category” and “within-category” pairs based on the place of category boundaries that were determined for each participant individually. In the discrimination task participants were presented with pairs of synthetic speech sounds and asked to indicate whether the sounds are “same” or “different”. Proportions of “different” responses are plotted. Participants gave more “different” responses when discriminating across-category pairs than within-category pairs, indicating categorical perception of speech sounds. Each participant performed the discrimination tasks before a low-frequency TMS train was applied over the lip representation in the left M1 cortex (pre-TMS) and after it (post-TMS). After TMS, participants were poorer in discriminating across-category pairs that included lip-articulated speech sounds (“ba” vs “da” and “pa” vs “ta”) than before TMS. Discriminability of other pairs stayed stable. Data are from Möttönen and Watkins (Citation2009). *p < .01, **p < .001.
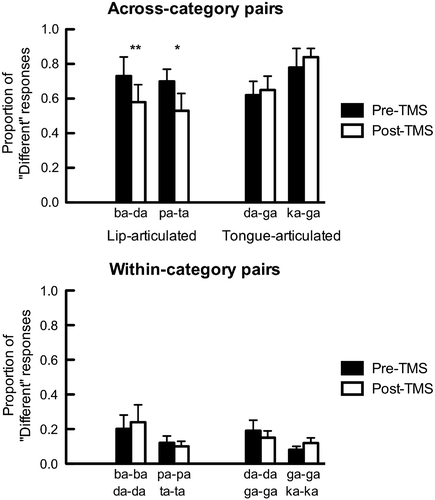
Using a dual-pulse paradigm, D'Ausilio et al. (Citation2009) have also found evidence supporting the contribution of the articulatory motor system to speech sound identification. Just before presentation of each syllable in noise the left motor cortex was primed by applying two TMS pulses either over the lip or tongue representation. The pulses over the lip area facilitated identification of the lip-articulated syllables (“pa”), whereas the pulses over the tongue area facilitated the identification of tongue-articulated syllables (“ta”). This TMS-induced facilitation was demonstrated by improved accuracy and reduced reaction times in this study. More recently, D'Ausilio, Bufalari, Salmas, Busan, and Fadiga (Citation2011) have demonstrated that dual pulses over the representation of the larynx in the left motor cortex reduce reaction times in a demanding vocal pitch discrimination task (compared to dual pulses over the representations of the articulators), suggesting that this motor region, which controls phonation during speech production, may contribute to processing of pitch of speech sounds.
Sato, Tremblay, and Gracco (Citation2009) found that low-frequency rTMS over the left ventral premotor cortex increased participants' reaction times in a phonetic segmentation task, but not in phoneme identification and syllable discrimination tasks. The accuracy was not affected by rTMS in any of the tasks. All speech stimuli were presented without noise in this study. These negative findings, and the fact that effects of TMS were weak and modulatory in previous studies (D'Ausilio et al., Citation2009; Meister et al., Citation2007; Möttönen & Watkins, Citation2009), have been interpreted in support of the argument that the motor system is not necessary for speech perception and that it contributes to speech perception only when listening conditions are compromised (Hickok et al., Citation2011; McGettigan, Agnew, & Scott, Citation2010). This may be true, but it is important to keep in mind the limitations of the TMS technique and the extent to which TMS can affect behaviour. TMS can add some noise to the neural processes in the stimulated areas but it cannot stop functioning of these areas. For example, during rTMS-induced disruption of the lip representation in the M1 cortex participants are able to move their lips and—unsurprisingly—comprehend clear speech (unpublished observations). Because the effects of TMS on neural processes in the stimulated areas are subtle, very sensitive behavioural tasks must be used to detect their behavioural consequences. Therefore in previous TMS studies noise has been added to the speech sounds (D'Ausilio et al., Citation2009; Meister et al., Citation2007) or their formant transitions have been manipulated (Möttönen & Watkins, Citation2009) to make their identification difficult. The fact that rTMS over the motor cortex can impair only slightly the ability to recognise and discriminate these types of speech sounds, but cannot abolish these abilities completely, should not be interpreted as indicating that the motor system plays a role only when speech perception is difficult.
Further TMS studies using variable tasks and speech stimuli (degraded and non-degraded) are needed to clarify in which conditions the articulatory motor system contributes to speech perception. In particular, it is not entirely clear whether in previous studies TMS-induced modulations in the motor system influenced task-related processes (such as phonemic segmentation and verbal working memory) that are not crucial for speech perception in everyday life. Furthermore, the tasks can force the participants to focus their attention on distinctive features of speech sounds. Therefore a challenge for further studies is to design tasks that are as natural as possible. Also, since previous TMS studies have focused on determining the role of the motor cortex in identification and discrimination of stop plosives that differ in the place of articulation, further studies focusing on other articulatory features of consonants (e.g., the manner of articulation) and vowels are needed.
Patients with real lesions have provided crucial information about the contributions of different brain areas to speech and language functions since the days of Wernicke and Broca. So far, studies in patients with lesions in the speech production system have reported somewhat conflicting evidence on these patients' speech perception abilities. Our attention was drawn to neuropsychological data obtained by Laughlin Taylor, who worked at the Montreal Neurological Institute and Hospital and tested patients before and after surgery in which part or all of their sensorimotor face area around the central sulcus was removed because of epilepsy (Taylor, Citation1979; see also Kolb & Wishaw, Citation2003, p. 417). Among other tests, Taylor presented patients with a phoneme identification task (Stitt & Huntington, Citation1969) requiring them to point to one of six letters corresponding to the six stop plosives (“p”, “b”, “t”, “d”, “k”, “g”) to indicate the phoneme heard. The phonemes were embedded in nonwords constructed by combining each with a nasal or a fricative sound and beginning and ending with the vowel “a” as in “father” (e.g., “apma”,” abma”, “atma”, “adma”, “akma”, “agma”). Taylor found that patients with lesions to the left face motor area recognised fewer than half of the stop plosives before (42%) and after (28%) the surgery. These patients performed worse than patients with lesions in the right face area (before: 63%, after: 68%), left frontal lobe patients (before: 58%, after: 58%) and left temporal lobe patients (before: 72%, after: 66%) even though the sizes of the surgical excisions in all of these groups were typically considerably bigger than the face area removals in the left hemisphere (Taylor, Citation1979; Kolb & Milner, Citation1981). Verbal fluency was also significantly impaired in patients with left central face area lesions compared even to left frontal lobe patients who also show restricted verbal fluency. However, they were unimpaired at performance of complex sequences of arm and facial movements (Kolb & Milner, Citation1981). This latter finding is somewhat at odds with a report that impairments in phoneme identification and in copying oral movements can be elicited by cortical stimulation of the same site, including stimulation of the left motor representation of the face (Ojemann & Mateer, Citation1979). Taylor concluded that the left face area patients “were unable to make effective use of phonetic elements of language” (Taylor, Citation1979, p. 171). It should be noted, however, that the central lesions extend to the post-central gyrus and although somatosensory function recovered well if the lesion was limited to the face area, we cannot rule out the effects on speech perception of damage to non-motor cortex. Also, the patients described by Taylor and Ojemann typically have had intractable epilepsy for many years and so may not be representative of normal brain organisation. These intriguing data warrant further investigations of similar patients with modern imaging techniques to determine exact lesion location and possible diaschisis.
There is also evidence that patients with Broca's aphasia are impaired in recognition of auditory words that are degraded, although their performance is at ceiling in recognition of non-degraded words (Moineau, Dronkers, & Bates, Citation2005). Patients with lesions encompassing the inferior frontal gyrus have also been suggested to be mildly impaired in syllable discrimination (Baker, Blumstein, & Goodglass, Citation1981; Blumstein, Citation2009; Blumstein, Baker, & Goodglass, Citation1977). On the other hand, speech perception can be relatively unimpaired in patients whose speech production abilities are severely impaired (for a review see Hickok, Citation2010; see also Bishop, Brown, & Robson, Citation1990, for speech perception in cerebral palsy). Recently a study in aphasic patients with fronto-parietal lesions (i.e., lesions in the “mirror neuron system”) demonstrated that these patients show normal speech perception performance when non-degraded auditory stimuli are used (Rogalsky, Love, Driscoll, Anderson, & Hickok, Citation2011). These findings are consistent with the idea that the motor speech production system does not contribute to speech perception in optimal listening conditions.
The “virtual lesion” approach has several strengths relative to patient studies in the investigation of causal relationships between brain and behaviour (Walsh & Cowey, Citation2000). Damage to a particular region in patients is rare, and these natural lesions are often widespread and can lead to functional reorganisation of the brain over time. TMS-induced disruptions are temporary and more focal, which allows investigation of contributions of relatively small brain regions (e.g., motor representations of articulators) to behaviour in the healthy human brain. Due to these strengths, the “virtual lesion” approach has become a widely used tool. However, the approach has some limitations. First, it is not fully understood how TMS modulates neuronal processing (see, e.g., Classen & Stefan, Citation2008; Ziemann, Citation2010). Second, TMS can affect functioning of brain regions that are connected to the stimulated region. This complicates the conclusions that can be drawn from “virtual lesion” studies, because impairment in behaviour can be due to disrupted processing in connected brain regions, not only in the stimulated brain region (see, e.g., Siebner, Hartwigsen, Kassuba, & Rothwell, Citation2009). Moreover, it is difficult to estimate the size of the cortical area that is directly stimulated by a TMS coil. The (usually unwanted) spread of stimulation depends on the stimulation intensity. Therefore, it is advantageous to use as low intensity as possible in “virtual lesion” studies, which aim to test contributions of focal brain areas to behaviour (e.g., sub-threshold intensity when M1 is stimulated). Appropriate control sites and tasks are also essential. The articulatory-feature-specific effects found in our “virtual lesion” study suggest that it is possible to suppress excitability of motor representations in the M1 cortex selectively using sub-threshold low-frequency rTMS (Möttönen & Watkins, Citation2009). Also, rTMS over the hand motor representation have been shown to suppress specifically MEPs recorded from the hand, but not the biceps, muscles, suggesting that low-frequency rTMS can induce a relatively spatially focused effect within the cortex under the coil (Muellbacher, Ziemann, Boroojerdi, & Hallet, Citation2000).
COMBINING TMS WITH OTHER IMAGING MODALITIES: A NEW TOOL TO STUDY AUDITORY-MOTOR INTERACTIONS UNDERLYING SPEECH PERCEPTION
The combination of TMS with techniques that can measure brain activity in the whole brain makes it possible to look at how TMS affects neural processes outside the stimulated region (for reviews, see Driver, Blankenburg, Bestmann, Vanduffel, & Ruff, Citation2009; O'Shea, Taylor, & Rushworth, Citation2008; Ruff, Driver, & Bestmann, Citation2009). Since brain regions interact with each other during perceptual and cognitive processes, it is not surprising that “a virtual lesion” in one of the brain regions that are engaged in a process modulates functioning of other brains regions that are also engaged in the same process. Combining TMS with other techniques provides a new interesting method to examine how interacting brain regions support perception (e.g., Ruff et al., Citation2006, Citation2008).
There is strong evidence that the auditory regions in the superior temporal cortex play a critical role in speech perception. Therefore, in order to advance our understanding of the role of the motor system in speech perception, it is essential to investigate how the auditory and motor systems interact during speech processing and how this auditory-motor interaction supports speech perception. These questions can be addressed using a combination of TMS with brain imaging. For example, it is possible to examine influences of TMS-induced disruptions in the articulatory motor system on processing of speech signals in the superior temporal cortex using electroencephalography (EEG), magnetoencephalography (MEG), fMRI, and PET. Unpublished data from combined TMS and EEG experiments carried out in our laboratory demonstrate that TMS-induced virtual lesions in the articulatory motor cortex modulate automatic discrimination of speech sounds in the auditory system, suggesting the auditory and motor systems are functionally coupled during speech processing (Möttönen, Dutton, & Watkins, Citation2011). Importantly, the combination of TMS and neuroimaging also provides a tool to look at motor contributions to different stages of speech processing without demanding behavioural tasks that can be quite artificial and recruit additional processes that are not necessary for speech perception per se.
CONCLUSIONS AND FUTURE DIRECTIONS
The TMS studies reviewed here have provided consistent evidence that the articulatory motor system is activated during listening to speech and that it contributes to performance in various speech perception tasks. However, the role of the motor system in speech perception is not yet fully understood. Future experiments are needed to clarify in which conditions the motor system is recruited for processing of speech sounds. For example, the effects of the properties of speech signals, experimental tasks and selective attention need to be examined in future studies.
In this article we have focused mainly on studies that have looked at the role of the motor system in phonological processing of speech sounds. It is, however, likely that the motor system plays a role also in other aspects of speech and language processing, for example, in semantic processing of action-related words (Pulvermüller, Citation2005) and in turn-taking during conversations (Scott et al., Citation2009). One of the claims of Liberman's motor theory of speech perception was that “speech is special”, meaning that only speech perception engages motor processes (Liberman et al., Citation1967; Liberman & Mattingly, Citation1985). It should be noted that according to current understanding this is not true, since the motor system seems to be involved in processing of variety of sensory inputs.
A few TMS studies have provided support for the idea that the speech sounds are processed in an articulatory-feature specific manner in the motor system. MEPs recorded from the tongue muscle are enhanced specifically during listening to words that include phonemes that are produced by closing the vocal tract with the tongue (e.g., “r”), but not during listening to words that that include phonemes that are produces by closing the vocal tract with the lips (e.g., “f”) (Fadiga et al. Citation2002; Roy et al., Citation2008). Also, discrimination and identification of consonants that differ in place of articulation is affected in a somatotopic manner by stimulation of motor lip and tongue representations (D'Ausilio et al., Citation2009; Möttönen & Watkins, Citation2009). Although comparing consonants that differ in place of articulation has been a logical starting point and provided valuable information about motor influences on speech sound processing, categorising phonemes to lip- and tongue-articulated ones should be seen as a simplification. When continuous speech is produced, each articulator moves in time in a well-controlled manner and the articulatory movements are modulated by their phonetic context (due to coarticulation). Therefore it is a simplification to say, for example, that the lips would not be involved when dental and velar consonants (e.g., “t” and “k”) are produced. In line with this the lip MEPs are enhanced even when TMS pulses are randomly delivered during continuous speech, not time-locked to “lip-articulated” phonemes (Murakami et al., Citation2011; Watkins et al., Citation2003). Further studies should try to take into account the complexity of the articulatory movements during speech production.
In face-to-face communication a speaker's articulatory movements are visible. Viewing these movements, not only listening to speech, activates the articulatory motor system (Campbell et al., Citation2001; Murakami et al., Citation2011; Watkins et al., Citation2003). It has been suggested that the motor system generates forward models of sensory consequences of observed articulatory movements (Kauramäki et al., Citation2010; Möttönen, Järveläinen, Sams, & Hari, Citation2005; Sams et al., Citation2005; Skipper et al., Citation2007; van Wassenhove, Grant, & Poeppel, Citation2005). It is likely that the motor system contributes to the integration of auditory and visual speech signals during audiovisual speech perception (Callan et al., Citation2003, Citation2004; Jääskeläinen, Citation2010; Ojanen et al., Citation2005). The articulatory motor system may have a stronger role in speech processing when the speaker's articulatory movements are visible compared to when the perceiver has to rely on auditory speech signals alone.
TMS has proven to be a powerful tool in investigating the role of the articulatory motor system in speech processing. This technique has potential to help speech scientists to address many remaining open questions in this research area. In our opinion, to better understand the role of the motor system in the processing of auditory and visual speech signals, it is important to investigate its interaction with the auditory system and other brain regions that support speech perception. Combining TMS with brain-imaging techniques offers a promising tool with which to examine these interregional interactions during speech processing.
Acknowledgments
KW is grateful to the late Laughlin Taylor for discussions of the data in patients with face area lesions, and to Ned Jenkinson for discussions of TMS effects. RM was supported by the Medical Research Council.
REFERENCES
- Antal , A. , Polania , R. , Schmidt-Samoa , C. , Dechent , P. and Paulus , W. 2011 . Transcranial direct current stimulation over the primary motor cortex during fMRI . NeuroImage , 55 ( 2 ) : 590 – 596 .
- Aziz-Zadeh , L. , Iacoboni , M. , Zaidel , E. , Wilson , S. and Mazziotta , J. 2004 . Left hemisphere motor facilitation in response to manual action sounds . European Journal of Neuroscience , 19 ( 9 ) : 2609 – 2612 .
- Baker , E. , Blumstein , S. E. and Goodglass , H. 1981 . Interaction between phonological and semantic factors in auditory comprehension . Neuropsychologia , 19 ( 1 ) : 1 – 15 .
- Binder , J. R. , Frost , J. A. , Hammeke , T. A. , Bellgowan , P. S. , Springer , J. A. Kaufman , J. N. 2000 . Human temporal lobe activation by speech and nonspeech sounds . Cerebral Cortex , 10 : 512 – 528 .
- Bishop , D. V. , Brown , B. B. and Robson , J. 1990 . The relationship between phoneme discrimination, speech production, and language comprehension in cerebral-palsied individuals . Journal of Speech and Hearing Research , 33 ( 2 ) : 210 – 219 .
- Blumstein , S. E. 2009 . Auditory word recognition: Evidence from aphasia and functional neuroimaging . Lang Linguistic Compass , 3 ( 4 ) : 824 – 838 .
- Blumstein , S. E. , Baker , E. and Goodglass , E. 1977 . Phonological factors in auditory comprehension in aphasia . Neuropsychologia , 15 : 19 – 30 .
- Callan , D. , Callan , A. , Gamez , M. , Sato , M. A. and Kawato , M. 2010 . Premotor cortex mediates perceptual performance . NeuroImage , 51 ( 2 ) : 844 – 858 .
- Callan , D. E. , Jones , J. A. , Munhall , K. , Kroos , C. , Callan , A. M. and Vatikiotis-Bateson , E. 2004 . Multisensory integration sites identified by perception of spatial wavelet filtered visual speech gesture information . Journal of Cognitive Neuroscience , 16 ( 5 ) : 805 – 816 .
- Callan , D. E. , Jones , J. A. , Munhall , K. G. , Callan , A. M. , Kroos , C. and Vatikiotis-Bateson , E. 2003 . Neural processes underlying perceptual enhancement by visual speech gestures . Neuroreport , 14 : 2213 – 2217 .
- Campbell , R. , MacSweeney , M. , Surguladze , S. , Calvert , G. , McGuire , P. Suckling , J. 2001 . Cortical substrates for the perception of face actions: An fMRI study of the specificity of activation for seen speech and for meaningless lower-face acts (gurning) . Brain Research Cognitive Brain Research , 12 ( 2 ) : 233 – 243 .
- Cattaneo , L. , Voss , M. , Brochier , T. , Prabu , G. , Wolpert , D. M. and Lemon , A. 2005 . A cortoco-cortical mechanism mediating object-driven grasp in humans . Proceedings of the National Academy of Sciences of the United States of America , 102 ( 3 ) : 898 – 903 .
- Classen , J. and Stefan , K. 2008 . “ Changes in TMS measures induced by repetitive TMS ” . In The Oxford handbook of transcranial stimulation , Edited by: Wassermann , E. M. , Epstein , C. M. , Zieman , U. , Walsh , V. , Paus , T. and Lisanby , S. H. 185 – 200 . Oxford , , UK : Oxford University Press .
- Curio , G. , Neuloh , G. , Numminen , J. , Jousmäki , V. and Hari , R. 2000 . Speaking modifies voice-evoked activity in the human auditory cortex . Human Brain Mapping , 9 ( 4 ) : 183 – 191 .
- D'Ausilio , A. , Bufalari , I. , Salmas , P. , Busan , P. and Fadiga , L. 2011 . Vocal pitch discrimination in the motor system . Brain and Language , 118 ( 1–2 ) : 91 – 214 .
- D'Ausilio , A. , Pulvermüller , F. , Salmas , P. , Bufalari , I. , Begliomini , C. and Fadiga , L. 2009 . The motor somatotopy of speech perception . Current Biology , 19 ( 5 ) : 381 – 385 .
- Dehaene-Lambertz , G. , Pallier , C. , Serniclaes , W. , Sprenger-Charolles , L. , Jobert , A. and Dehaene , S. 2005 . Neural correlates of switching from auditory to speech perception . NeuroImage , 24 : 21 – 33 .
- Diehl , R. L. , Lotto , A. J. and Holt , L. L. 2004 . Speech perception . Annual Review of Psychology , 55 : 149 – 179 .
- Driver , J. , Blankenburg , F. , Bestmann , S. , Vanduffel , W. and Ruff , C. C. 2009 . Concurrent brain-stimulation and neuroimaging for studies of cognition . Trends in Cognitive Sciences , 13 ( 7 ) : 319 – 327 .
- Fadiga , L. , Craighero , L. , Buccino , G. and Rizzolatti , G. 2002 . Speech listening specifically modulates the excitability of tongue muscles: A TMS study . European Journal of Neuroscience , 15 ( 2 ) : 399 – 402 .
- Fadiga , L. , Fogassi , L. , Pavesi , G. and Rizzolatti , G. 1995 . Motor facilitation during action observation: A magnetic stimulation study . Journal of Neurophysiology , 73 ( 6 ) : 2608 – 2611 .
- Galantucci , B. , Fowler , C. A. and Turvey , M. T. 2006 . The motor theory of speech perception reviewed . Psychonomic Bulletin Review , 13 ( 3 ) : 361 – 377 .
- Hickok , G. 2010 . The role of mirror neurons in speech perception and action word semantics . Language and Cognitive Processes , 25 ( 6 ) : 746 – 776 .
- Hickok , G. , Houde , J. and Rong , F. 2011 . Sensorimotor integration in speech processing: Computational basis and neural organisation . Neuron , 69 ( 3 ) : 407 – 422 .
- Houde , J. F. and Jordan , M. I. 1998 . Sensorimotor adaptation in speech production [see comments] . Science , 279 ( 5354 ) : 1213 – 1216 .
- Jääskeläinen , I. P. 2010 . The role of speech production system in audiovisual speech perception . Open Neuroimaging Journal , 4 : 30 – 36 .
- Kauramäki , J. , Jääskeläinen , I. P. , Hari , R. , Möttönen , R. , Rauschecker , J. P. and Sams , M. 2010 . Lipreading and covert speech production similarly modulate human auditory-cortex responses to pure tones . Journal of Neuroscience , 30 ( 4 ) : 1314 – 1321 .
- Kolb , B. and Milner , B. 1981 . Performance of complex arm and facial movements after focal brain lesions . Neuropsychologia , 19 : 505 – 514 .
- Kolb , B. and Wishaw , I. Q. 2003 . Fundamentals of human neuropsychology , 5th , New York , NY : Worth Publishers .
- Liberman , A. M. , Cooper , F. S. , Shankweiler , D. P. and Studdert-Kennedy , M. 1967 . Perception of the speech code . Psychological Review , 74 ( 6 ) : 431 – 461 .
- Liberman , A. M. and Mattingly , I. G. 1985 . The motor theory of speech perception revised . Cognition , 21 ( 1 ) : 1 – 36 .
- Logothetis , N. K. 2002 . The neural basis of the blood-oxygen-level-dependent functional magnetic resonance imaging signal . Philosophical Transactions of the Royal Society of London B , 357 : 1003 – 1037 .
- Lotto , A. J. , Hickok , G. S. and Holt , L. L. 2009 . Reflections on mirror neurons and speech perception . Trends in Cognitive Sciences , 13 ( 3 ) : 110 – 114 .
- McGettigan , C. , Agnew , Z. K. and Scott , S. K. 2010 . Are articulatory commands automatically and involuntarily activated during speech perception? . Proceedings of the National Academy of Sciences of the United States of America , 107 ( 12 ) E42; author reply E43
- Meister , I. G. , Wilson , S. M. , Deblieck , C. , Wu , A. D. and Iacoboni , M. 2007 . The essential role of premotor cortex in speech perception . Current Biology , 17 ( 19 ) : 1692 – 1696 .
- Moineau , S. , Dronkers , N. F. and Bates , E. 2005 . Exploring the processing continuum of single-word comprehension in aphasia . Journal of Speech, Language and Hearing Research , 48 ( 4 ) : 884 – 896 .
- Möttönen , R. , Calvert , G. A. , Jääskeläinen , I. P. , Matthews , P. M. , Thesen , T. Tuomainen , J. 2006 . Perceiving identical sounds as speech or non-speech modulates activity in the left posterior superior temporal sulcus . NeuroImage , 30 : 563 – 569 .
- Möttönen , R. , Dutton , R. and Watkins , K. E. 2011 . Auditory-motor interaction supports automatic discrimination of speech sounds , Manuscript in preparation
- Möttönen , R. , Järveläinen , J. , Sams , M. and Hari , R. 2005 . Viewing speech modulates activity in the left SI mouth cortex . NeuroImage , 24 ( 3 ) : 731 – 737 .
- Möttönen , R. and Watkins , K. E. 2009 . Motor representations of articulators contribute to categorical perception of speech sounds . Journal of Neuroscience , 29 ( 31 ) : 9819 – 9825 .
- Muellbacher , W. , Ziemann , U. , Boroojerdi , B. and Hallet , M. 2000 . Effects of low-frequency transcranial magnetic stimulation on motor excitability and basic motor behaviour . Clinical Neurophysiology , 111 ( 6 ) : 1002 – 1007 .
- Murakami , T. , Restle , J. and Ziemann , U. 2011 . Observation–execution matching and action inhibition in human primary motor cortex during viewing of speech-related lip movements or listening to speech . Neuropsychologia , 49 ( 7 ) : 2045 – 2054 .
- Nitsche , M. A. and Paulus , W. 2000 . Excitability changes induced in the human motor cortex by weak transcranial direct current stimulation . Journal of Physiology , 527 : 633 – 639 .
- Numminen , J. and Curio , G. 1999 . Differential effects of overt, covert and replayed speech on vowel-evoked responses of the human auditory cortex . Neuroscience Letters , 272 ( 1 ) : 29 – 32 .
- O'Shea , J. , Taylor , P. C. and Rushworth , M. F. 2008 . Imaging causal interactions during sensorimotor processing . Cortex , 44 ( 5 ) : 598 – 608 .
- Ojanen , V. , Möttönen , R. , Pekkola , J. , Jääskeläinen , I. P. , Joensuu , R. Autti , T. 2005 . Processing of audiovisual speech in Broca's area . NeuroImage , 25 ( 2 ) : 333 – 338 .
- Ojemann , G. and Mateer , C. 1979 . Human language cortex: Localisation of memory, syntax, and sequential motor-phoneme identification systems . Science , 205 ( 4413 ) : 1401 – 1403 .
- Pulvermüller , F. 2005 . Brain mechanisms linking language and action . Nature Reviews Neuroscience , 6 ( 7 ) : 576 – 582 .
- Pulvermüller , F. and Fadiga , L. 2010 . Active perception: Sensorimotor circuits as a cortical basis for language . Nature Reviews Neuroscience , 11 ( 5 ) : 351 – 360 .
- Pulvermüller , F. , Huss , M. , Kherif , F. , Moscoso del Prado Martin , F. , Hauk , O. and Shtyrov , Y. 2006 . Motor cortex maps articulatory features of speech sounds . Proceedings of the National Academy of Sciences of the United States of America , 103 ( 20 ) : 7865 – 7870 .
- Rauschecker , J. P. and Scott , S. K. 2009 . Maps and streams in the auditory cortex: Nonhuman primates illuminate human speech processing . Nature Reviews Neuroscience , 12 ( 6 ) : 718 – 724 .
- Rogalsky , C. , Love , T. , Driscoll , D. , Anderson , S. W. and Hickok , G. 2011 . Are mirror neurons the basis of speech perception? Evidence from five cases with damage to the purported human mirror system . Neurocase , 17 ( 2 ) : 178 – 187 .
- Rosa , M. J. , Kilner , J. M. and Penny , W. D. 2011 . Bayesian comparison of neurovascular coupling models using EEG-fMRI . PLoS Computational Biology , 7 ( 6 ) : e10002070
- Rösler , K. M. and Magistris , M. R. 2008 . “ The size of motor-evoked potentials: Influencing parameters and quantification ” . In The Oxford handbook of transcranial stimulation , Edited by: Wassermann , E. M. , Epstein , C. M. , Zieman , U. , Walsh , V. , Paus , T. and Lisanby , S. H. 77 – 90 . Oxford , , UK : Oxford University Press .
- Rothwell , J. C. 2008 . “ TMS measures and voluntary motor function ” . In The Oxford handbook of transcranial stimulation , Edited by: Wassermann , E. M. , Epstein , C. M. , Zieman , U. , Walsh , V. , Paus , T. and Lisanby , S. H. 171 – 184 . Oxford , , UK : Oxford University Press .
- Roy , A. C. , Craighero , L. , Fabbri-Destro , M. and Fadiga , L. 2008 . Phonological and lexical motor facilitation during speech listening: A transcranial magnetic stimulation study . Journal of Physiology, Paris , 102 ( 1–3 ) : 101 – 105 .
- Ruff , C. C. , Bestmann , S. , Blankenburg , F. , Bjoertomt , O. , Josephs , O. Weiskopf , N. 2008 . Distinct causal influences of parietal versus frontal areas on human visual cortex: Evidence from concurrent TMS-fMRI . Cerebral Cortex , 18 ( 4 ) : 817 – 827 .
- Ruff , C. C. , Blankenburg , F. , Bjoertomt , O. , Bestmann , S. , Freeman , E. Haynes , J. D. 2006 . Concurrent TMS-fMRI and psychophysics reveal frontal influences on human retinotopic visual cortex . Current Biology , 16 ( 15 ) : 1479 – 1488 .
- Ruff , C. C. , Driver , J. and Bestmann , S. 2009 . Combining TMS and fMRI: From ‘virtual lesions’ to functional-network accounts of cognition . Cortex , 45 ( 9 ) : 1043 – 1049 .
- Sams , M. , Möttönen , R. and Sihvonen , T. 2005 . Seeing and hearing others and oneself talk . Brain Research Cognitive Brain Research , 23 ( 2–3 ) : 429 – 435 .
- Sato , M. , Tremblay , P. and Gracco , V. L. 2009 . A mediating role of the premotor cortex in phoneme segmentation . Brain & Language , 111 ( 1 ) : 1 – 7 .
- Scott , S. K. , McGettigan , C. and Eisner , F. 2009 . A little more conversation, a little less action – candidate roles for the motor cortex in speech perception . Nature Reviews Neuroscience , 10 ( 4 ) : 295 – 302 .
- Shimazu , H. , Maier , M. A. , Cerri , G. , Kirkwood , P. A. and Lemon , R. N. 2004 . Macaque ventral premotor cortex exerts powerful facilitation of motor cortex outputs to upper limb motoneurons . Journal of Neuroscience , 24 ( 5 ) : 1200 – 1211 .
- Siebner , H. R. , Hartwigsen , G. , Kassuba , T. and Rothwell , J. C. 2009 . How does transcranial magnetic stimulation modify neuronal activity in the brain? Implications for studies of cognition . Cortex , 45 ( 9 ) : 1035 – 1042 .
- Skipper , J. I. , van Wassenhove , V. , Nusbaum , H. C. and Small , S. L. 2007 . Hearing lips and seeing voices: How cortical areas supporting speech production mediate audiovisual speech perception . Cerebral Cortex , 17 ( 10 ) : 2387 – 2399 .
- Stitt , C. and Huntington , D. 1969 . Some relationships among articulation, auditory abilities, and certain other variables . Journal of Speech and Hearning Research , 12 : 576 – 593 .
- Stokes , M. G. , Chambers , C. D. , Gould , I. C. , Henderson , T. R. , Janko , N. E. Allen , N. B. 2005 . Simple metric for scaling motor threshold based on scalp-cortex distance: Application to studies using transcranial magnetic stimulation . Journal of Neurophysiology , 94 ( 6 ) : 4520 – 4527 .
- Strafella , A. P. and Paus , T. 2000 . Modulation of cortical excitability during action observation: A transcranial magnetic stimulation study . Neuroreport , 11 ( 10 ) : 2289 – 1192 .
- Sundara , M. , Namasivayam , A. K. and Chen , R. 2001 . Observation-execution matching system for speech: A magnetic stimulation study . Neuroreport , 12 ( 7 ) : 1341 – 1344 .
- Takai , O. , Brown , S. and Liotti , M. 2010 . Representation of the speech effectors in the human motor cortex: Somatotopy or overlap? . Brain and Language , 113 ( 1 ) : 39 – 44 .
- Taylor , L. B. 1979 . “ Psychological asessment of neurosugical patients ” . In Functional neurosurgery , Edited by: Rasmussen , T. and Marino , R. 165 – 180 . New York , NY : Raven Press .
- van Wassenhove , V. , Grant , K. W. and Poeppel , D. 2005 . Visual speech speeds up the neural processing of auditory speech . Proceedings of the National Academy of Sciences of the United States of America , 102 ( 4 ) : 1181 – 1186 .
- Walsh , V. and Cowey , A. 2000 . Transcranial magnetic stimulation and cognitive neuroscience . Nature Reviews Neuroscience , 1 ( 1 ) : 73 – 79 .
- Watkins , K. and Paus , T. 2004 . Modulation of motor excitability during speech perception: The role of Broca's area . Journal of Cognitive Neuroscience , 16 ( 6 ) : 978 – 987 .
- Watkins , K. E. , Strafella , A. P. and Paus , T. 2003 . Seeing and hearing speech excites the motor system involved in speech production . Neuropsychologia , 41 ( 8 ) : 989 – 994 .
- Wilson , S. M. 2009 . Speech perception when the motor system is compromised . Trends in Cognitive Sciences , 13 ( 8 ) : 329 – 330 . author reply 330–321
- Wilson , S. M. , Saygin , A. P. , Sereno , M. I. and Iacoboni , M. 2004 . Listening to speech activates motor areas involved in speech production . Nature Neuroscience , 7 ( 7 ) : 701 – 702 .
- Ziemann , U. 2010 . TMS in cognitive neuroscience: Virtual lesion and beyond . Cortex , 46 ( 1 ) : 124 – 127 .