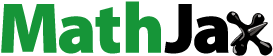
ABSTRACT
Purpose: By using four national data sets, this study aimed to examine the changes in U.S. children’s fundamental movement skills (FMS) from 1985 to 2019. Method: Three Tests Gross of Motor Development (TGMD) normative studies, i.e. TGMD-1 (N = 909, male% = 50), TGMD-2 (N = 1143, male% = 50) and TGMD-3 (N = 864, male% = 51), and the TGMD-2 data of the 2012 NHANES National Youth Fitness Survey (NNYFS-2012; raw N = 352, national represented population = 12,509,706, male% = 51) were used for analyses. Each item in TGMD-2/NNYFS and TGMD-3 was carefully examined, and these that did not match to TGMD-1 were deleted. Cohen’s D effect size (ES) was utilized for the difference among year-to-year comparisons. Result: From 1985 to 2000, FMS in US children of 3–7 years old (90% increase & 10% no change) increased temporarily, but remained stable (17% increase & 83% no change) among 8–10 years old. Between 2000 and 2019, however, U.S. children began to demonstrate a slight/stable drop in FMS among 3–5 years old (22% decrease & 78% no change), and a distinct decline among 6–10 years old (80% decrease & 20% no change). While children with normal BMI showed the highest FMS score, the direct cause of reduction in FMS is still unknown. Conclusion: An overall up-and-down change was observed in U.S. children’s FMS between 1985 and 2019, and more longitudinal studies with FMS-related variables are needed.
KEYWORDS:
Fundamental movement skills (FMS) are defined as “motor skills that involve the large, force-producing muscles of the trunk, arms and legs” (Clark, Citation1994, p. 245). FMS are regarded as “building blocks” that can be altered and refined to various movement situations and sports in the future for children, adolescents, and adults (Clark & Metcalfe, Citation2002; Goodway et al., Citation2020). Generally developed during childhood and subsequently, in context- and sport-specific skills, FMS include locomotor skills (LMS) (e.g., running and hopping), manipulative or object-control skills (OCS) (e.g., catching and throwing), and balance (e.g., one food standing) (Gallahue & Donnelly, Citation2003). In the 1970s, FMS began to gain traction as a unique discipline, advocating for the improvement of a variety of product- and process-oriented assessments (Cattuzzo et al., Citation2016).
FMS assessment can typically be divided into two categories: process- and product-oriented measures. Product-oriented measures emphasize the outcomes of skill execution (e.g., the velocity of a throw, or distance range) and process-oriented measures evaluate the quality of movements performed during skill execution (e.g., how a child performs during running). Since the 1970s, motor development research shifted from describing children’s motor performance to determining the causes of that performance (Clark & Whitall, Citation1989). Various measurements of FMS have been developed thereafter to evaluate LMS and OCS that are required to function in context-specific sports, games, and other daily physical activity (PA) (Logan et al., Citation2018). According to Logan et al. (Citation2018), the most widely used FMS assessment in practice is the Test of Gross Motor Development (TGMD, Ulrich, Citation1985), which is labeled as “TGMD-1” in this study to distinguish it from later versions. Included norm-referenced evaluation standards, the TMGD-1 was developed to address several issues with motor function assessment tools, including a lack of standardization, difficulties recognizing specific features of various movements, a lack of referential explanation, and the inability to conduct progress comparisons. The TGMD-1 was updated in 2000 as a follow-up to the original edition’s test reviews (TGMD-2; Ulrich, Citation2000). The TGMD-2 has been used widely in schools both in the U.S. and around the world, as well as in U.S. national studies, e.g., the National Youth Fitness Survey (NNYFS-2012). By collecting new normative data from 2014 to 2017, the TGMD-2 was further updated and published in 2019 (TGMD-3; Ulrich, Citation2019).
Corbin and Pangrazi (Citation1992) published an interesting study in Research Quarterly for Exercise and Sport titled: “Are American children and youth fit?” and suggested that most U.S. children and youth did not meet the criterion-referenced health standard, and also noted a decline of fitness after a decade-to-decade comparison. That begs the question of whether or not the FMS of U.S. children has declined, stayed unchanged, or improved. According to a literature search, there seem to be only international studies that have examined the secular trends in FMS so far (Hardy et al., Citation2013; Huotari et al., Citation2018; Roth et al., Citation2010). The first study (Roth et al., Citation2010) investigated secular trends in the FMS in German (3- to 7-year-old) children from 1973 to 2007 using the motor-proficiency-test (MOT 4–6) (Zimmer & Volkamer, Citation1987). In 2007, children acted equally or significantly improved in standing broad jump and obstacle-course tests compared to the children examined in 1973 and 1989; however, the scores in balancing and throwing dropped from 1985 to 2007. The second study (Hardy et al., Citation2013) examined a 13-year trend study in children and adolescents (9- to 15-year-olds) in 1997, 2004, and 2010 using process-oriented checklists for each skill (NSW Department of Education and Training, Citation2000). According to the study (Hardy et al., Citation2013), the number of components of each skill (e.g., four out of five trials) that each student correctly displayed was added to generate a total score for each skill. If all components of a skill were correctly demonstrated, the performance was defined as “competent,” otherwise, it was classified as “noncompetent.” That study showed that students’ FMS proficiency (i.e., four of five components of a skill were correctly demonstrated) was still low with prevalence rarely above 50%, particularly in kick and overarm throws by girls. Changes in practice and policy to facilitate the teaching of FMS in schools were credited with a significant increase in FMS competency from 2004 to 2010. The third study (Huotari et al., Citation2018) examined the secular trend in FMS (15- to 16-year-olds) in Finland between 2003 and 2010 using Figure 8 Test (OCS) and motor coordination track test (LMS and stability skills). Their findings demonstrated that, while the FMS sum index did not vary between boys and girls, there were losses in coordination test scores in both gender groups between 2003 and 2010, despite an improvement in girls’ OCS.
There has long been interest in U.S. children’s FMS although its secular trend has not been examined. Stodden et al. (Citation2008) extensively described a conceptual model for the role of FMS in children’s health. That model symbolized the relationship between FMS, PA, physical fitness, perceived FMS and weight status (e.g., body mass index [BMI]) across childhood). As indicated by a recent published work (Barnett et al., Citation2021), longitudinal and experimental evidence was conducted to examine the Stodden’s model. Overall, regarding pathways, studies indicated strong evidence for a negative association between weight status and FMS. Strong evidence for the pathway from FMS to fitness and indeterminate evidence for the opposite were thus documented. There was an indeterminate effect of the FMS to PA pathway and no evidence for the opposite. There was insufficient verification to establish a link between FMS and perceived FMS. For example, the study found that BMI (negative) and fitness (positive) were associated with developmental trajectories of FMS, but not PA in children from six age cohorts (aged 5–9 years) over a three-year period in Portuguese children (Reyes et al., Citation2019). A similar study with children aged 6–9 years discovered that fitness was related to FMS change but not PA, and that children with a more linear body shape/size had better FMS over time (Dos Santos et al., Citation2018). It is imperative to note, however, that fitness mediated the associations between FMS, PA, and body fatness, and that FMS mediated the associations between PA and body fatness, whereas PA simply influenced body fatness implicitly (Lima et al., Citation2017). All of these studies suggest that,due to its more stable trait, fitness in the Stodden et al. (Citation2008) model is more likely correlated with FMS than PA, which is a behavior and could vary greatly. The paradigms of FMS and fitness are indeed synergistically correlated, which might facilitate to elaborate why fitness seems to play a significant role in the model pathways (Barnett et al., Citation2021).
Similar to children’s fitness trends reported by Corbin and Pangrazi (Citation1992), one may wonder if U.S. children’ FMS have improved or declined over the past decades. Given the critical role of FMS in the development of an active and healthy lifestyle in children and youth, it is essential to explore how FMS evolve during childhood over time. Although there was some evidence demonstrating an association between FMS, PA, and physical fitness, recent literature reviews (Lopes et al., Citation2021) indicated that secular trend studies investigating changes in children’s FMS over time are limited.
Fortunately, the question if U.S. children’s FMS has changed can be examined by using four distinct data sets between 1985 and 2019, including the TGMD-1, TGMD-2, NNYFS-2012, and TGMD-3. By taking the advantage of these available data, firstly, the purpose of this study was to investigate if the FMS of U.S. children has changed over the past three decades; and secondly, to examine whether the FMS changes are related to the weight status of the children using NNYFS-2012 data. It is hypothesized that children’s FMS will have declined over the years.
Data and participants
The data of three raw TGMD normative studies, i.e., TGMD-1 (data collected in 1983–1984, N = 909, male% = 50, age-range = 3–10 year) (Ulrich, Citation1985), TGMD-2 (data collected in 1997–1998, N = 1143, male% = 50; age-range = 3–10 year) (Ulrich, Citation2000), and TGMD-3 (data collected in 2014–2017, N = 864, male% = 51; age-range = 3–10 year) (Ulrich, Citation2019), and the TGMD-2 data of NNYFS-2012 (data collected in 2012, “raw” N = 352, and N to the size of represented population = 12,509,706, male% = 51, age-range = 3–5 year; https://wwwn.cdc.gov/Nchs/Nnyfs/Y_GMX.htm) were used for this study. Two variations were observed across different TGMD versions. First, the subtest items varied slightly from one version to the next, e.g., “skip” was removed from the TGMD-2, but was reintroduced back in the TGMD-3. Second, the number of trials changed from three in the TGMD-1 to two in the TGMD-2/3. As a result, a score of “1” in TGMD-1 means “demonstrating mastery,” whereas a score of “2” means “demonstrating mastery” in TGMD-2/3, respectively.
All authors acknowledge ethical responsibility for the content of the manuscript and will accept the consequences of any ethical violation.
Data analysis
Each item in the TGMD-2/NNYFS-2012 and the TGMD-3 was carefully examined and deleted if the new or revised ones did not match to the TGMD-1. After matching at least two items through three versions of the TGMD, a total of nine subtests (LMS: run, gallop, hop, jump, and slide; OCS: strike, bounce, catch and throw) were included for final analysis. The details regarding how to select the inclusion and exclusion criteria were also added as Appendix 1 in the supplementary files.
The mean of the first and second trials from the TGMD-2/NNYFS-2012 and the TGMD-3 was computed to make a comparison with the TGMD-1 consistent because the TGMD-1 had three trials with “1” for performing two out of the three trials correctly, but the TGMD-2/NNYFS-2012 and the TGMD-3 both had two trials with “2” for mastering the criteria accurately.
To be able to conduct the regression, previous research found that when the number of events per variable (EPV) was 10 or higher, no major problems occurred. However, for EPV values less than 10, the regression coefficients were biased both positively and negatively (Peduzzi et al., Citation1996). Harrell (Citation2001) generally recommends at least 15 in generating the reliable outcome. A recent study also suggests that regression-based research use N ≥ 25 to avoid confusion and low reproducibility across disciplines (Jenkins et al., Citation2020). Unfortunately, our study only had four data points, therefore, did not meet this requirement. Additionally, we have conducted a pilot analysis with the trational linear regression using the four-data-point years (1985, 2000, 2012, and 2019) as the independent variable and the FMS as the dependent variable by age and gender. There were no differences (p > .05) in most FMS variable patterns based on age or gender. In contrast, the analyses based on Cohen’s d Effect Size (SE) provided more valuable information if we broke down in a detail year–year comparison, thus merited an appropriate strategy. As a result, the difference between each year was determined using Cohen’s D ES, with small effect = 0.2, medium effect = 0.5, and large effect = 0.8, where Cohen’s D = (M2 – M1) ⁄ SD pooled; while SD pooled = ((SD12 + SD22) ⁄ 2) (Cohen, Citation1988). The change and trend using the percentage of positive and negative ES from various aspects were further analyzed and summarized. According to Pate et al. in 2012, if the relationship between a fitness test and health outcomes has not been confirmed, a comparatively relative position method, e.g., set the classification below vs. at or above a specific percentile (e.g., 80th percentile), can be used to set cutoff-points. Accordingly, we used 80%/20% to determine the trend, i.e., the trend threshold was defined with 80% of ES (increased), 21%–79% of ES (no change), and 20% or less (declined).
Lastly, to examine the relationship between BMI and FMS, Cohen’s D ES was computed and compared between the “normal” vs. “Overweight/Obese” groups by using the NNYFS-2012 data, which included both FMS and weight status information.
Results
The U.S. Children displayed a temporary increase in FMS (LMS and OCS) at ages 3–7 years old, but showed a decrease/stable at 8–10 years old from 1985 to 2000. However, the U.S. children started to show a slight decline/stable in FMS (LMS and OCS) at ages 3–6 years old and a clear decline at ages 7–10 years old from 2000 to 2019. Some sex differences were also observed. Details on the descriptive and trend analyses of FMS can be found in , as mentioned below.
Table 1. Descriptive statistics (MSD) of each component of TGMD-1, TGMD-2a, TGMD-2b, and TGMD-3 between aged 3 and 10 years old, boys (example of selected subtests).
Table 2. ES of TGMD-1, TGMD-2a, TGMD-2b, and TGMD-3 between aged 3 and 10 years old, boys.
Table 3. Trends based on total FMS score, among different years (before 2000 vs. after 2000), 3–10-year children.
Table 4. Percentage of children demonstrating mastery (selected subtests) using NNYFS 2012 based on BMI categories on LMS, and OCS among 3-year-old boys and girls.
Due to the space constrain, shows only an example of mean and standard deviation (M SD) in the “Run” subtest in four different data points. Children’s FMS scores grew with age, e.g., in the TGMD-1, boys’ “Run” scores were 2.58
0.98 vs. 3.94
0.30 when they were ages 3–10 years old. The girls showed comparable trends with the “Run” scores of 2.33
0.88 vs. 3.86
0.35. More details of the remaining subtests can be found in Appendix 2 in the supplementary files.
shows the ES of each subtest when compared to one another across four data points in boys and girls aged 3–10 years old. For example, the ES of 0.38 in 3-year-old boys of “Run” in “TGMD-2a—TGMD-1” was calculated as (2.95–2.58)/((0.982 +0.972) ⁄ 2) from . Shown the rest in are the detailed “year vs. year comparison,” ES, with the latter years minus the previous years.
Based the ES reported on summarizes the percentage of “+ ES” (represents later year had a better performance) and “− ES” (later year had a poorer performance). According to Cohen’s D (Cohen, Citation1988), ES is categorized into small (0 to 0.19), medium (0.20 to 0.79), and large (0.8), respectively. The trend threshold (Pate et al., Citation2012) was defined with 80% of ES (increased), 21%–79% of ES as “no change,” and 20% or less ES as “declined.” The percentage of + ES and –ES in LMC was calculated from “run,” “gallop,” “hop,” “jump” and “slide.” The percentage of + ES and –ES in OCS was calculated from “strike,” “bounce,” “catch” and “overhand throw.” The percentage of + ES and –ES in “Total” was calculated from all sub-items from LMC, and OCS and the total raw score.
Overall, it appears that the trends before 2000 (1985–2000) and after 2000 (Citation2000–2012, 2000–2019, and 2012–2019) differ from each other based on the trend of total FMS total raw scores. From 1985 to 2000, FMS in US children of 3–7 years old (90% increase & 10% no change) increased temporarily, but remained stable (17% increase & 83% no change) among 8–10 years old. Between 2000 and 2019, however, U.S. children of 3–5 years old began to demonstrate a slight/stable drop in FMS (22% decrease & 78% no change), and a distinct decline among 6–10 years old (80% decrease & 20% no change). Additional information regarding the FMS subtest (LMS and OCS) can be found on Appendix 3 in the supplemental files.
Children at age 3-year old, between 1985 and 2000, both boys and girls showed an “increased” trend. Between 2000–2012, 2000–2019, and 2012–2019, boys and girls showed “no change” trend. Overall, children’s FMS at age 3-year old increased before 2000 but start to stay stable after 2000.
Children at age 4-year old, between 1985 and 2000, both boys and girls showed an “increased” trend. Between 2000 and 2012, boys and girls showed a “no change” trend. Between 2000 and 2019, both boys and girl showed a “declined” trend. Between 2012 and 2019, boys and girls showed “no change” trend. Overall, children’s FMS at age 4-year old increased before 2000 but start to decline between 2000 and 2019, but stay stable between 2000–2012 and 2012–2019.
Children at age 5-year old, between 1985 and 2000, both boys and girls showed an “increased” trend. Between 2000 and 2012, boys and girls showed a “no change” trend. Between 2000 and 2019, both boys and girls showed a “declined” trend. Between 2012 and 2019, boys and girls showed “no change” trend. Overall, children’s FMS at age 5-year old increased before 2000 but start to decline between 2000 and 2019, but stay stable between 2000–2012 and 2012–2019.
Children at age 6-year old, between 1985 and 2000, both boys and girls showed an “increased” trend. Since NNYFS only contained 3–5 years old data, therefore only year comparsion 2000–2019 being reported. Between 2000 and 2019, boys showed “declined” while girls showed “no change” trend. Overall, children’s FMS at age 6-year old showed increased before 2000 but start to decline in boys after 2000.
Children at age 7-year old, between 1985 and 2000, boys showed “no change” but girls showed an “increased” trend. Between 2000 and 2019, both boys and girl showed a “declined” trend. Overall, children’s FMS at 7-year old remained stable in boys, but increased in girls before 2000, then declined significantly in both boys and girls after 2000.
Children at age 8-year old, between 1985 and 2000, boys and girls showed “no change” trend. Between 2000 and 2019, both boys and girl showed a “declined” trend. Overall, children’s FMS at age 8-year old (both boys and girls) remained in stable before 2000, but begun to decline significantly after 2000.
Children at age 9-year old, between 1985 and 2000, both boys and girls showed “no change” trend. Between 2000 and 2019, both boys and girls showed a “declined” trend. Overall, children’s FMS at age 9-year showed no change before 2000 but declined significantly after 2000.
Children at age 10-year old, between 1985 and 2000, boys showed a “declined” but girls showed a “no change” trend. Between 2000 and 2019, boys showed a “declined” while girl showed “no change” trend. Overall, children’s FMS at age 10-year old declined in boys, but remained stable in girls before and after 2000.
Discussion
Despite the FMS trends had been documented by previous international studies, it is still impossible to compare them directly since each study used different FMS tests. While FMS comparability was recently conducted with the TGMD-2 and the TGMD-3 to examine how FMS scores changed among children in middle childhood (N = 270; Mage = 8 years 6 months) using percent of maximum possible (POMP) scores, the study did not represent national representatives. Since sometest items had been changed in different TGMD versions, our study employed the only same test measures utilized throught TGMD-1, TGMD-2, and TGMD-3. Furthermore, using the raw score for research questions in comparison is considered acceptable (Ulrich, Citation2017). The direct comparison to earlier years FMS using the same test items with national data in the U.S has not been done; as a result, the overall trend of U.S. children’s FMS is still unknown. This study was the first attempt to assess the changes of the U.S. children’s FMS using the data of the TGMD from 1985 to 2019. Overall, children showed an increase in FMS at ages 3–7 year old, but a decrease/stable at ages 8–10 year before the year 2000. However, children showed a slight decline/no change in FMS at ages three to six year and a decline at ages 7–10 year old, after 2000.
A possible explanation of the increased children’s FMS before 2000 in three-to seven-year-olds in this study might be due to the utilization and familiarization of the TGMD since 1985. For the same age range and research period, there was little published information. However, Hardy et al. (Citation2013) indicated that the observed rise in FMS competency was due to improvements in practice and policy to support the teaching of FMS in schools. Previous studies proved that FMS must be trained and strengthened and do not improve naturally or automatically over time (Clark, Citation2005). This was further supported by the meta-analysis study by Logan et al. (Citation2011), in which a significant improvement of FMS in children was documented (d = 0.39, p < .001), withOCS (d = 0.41, p < .001) and LMS (d = 0.45, p < .001) improved from pre- to post-intervention, respectively. In contrast, the overall effect size for control groups (i.e. free play) was insigninicant (d = 0.06, p = .33). Similarly, the study by Case and Yun (Citation2019) showed that interventions had a large effect on FMS performace among children with autism spectrum disorder ( = 0.99, SE = 0.19, p < .001, 95% confidence interval [0.62, 1.36]), using a random effects model. Robinson and Goodway (Citation2009) also discovered that children who were taught motor skills by specialists performed better in FMS than those who engaged in free-play. Despite the comparable findings, the method used, the year of study, and the age group studied differed from the study by Hardy et al. (Citation2013).
We were not able to examine the relationship between the TGMD and weight status in large-scale studies in the past since the TGMD-1, −2, and −3 studies did not collect height and body-weight information in this present study. Fortunately, 2012 NNYFS had provided the BMI categories of underweight, normal, overweight, and obese. Therefore, analyzing the national data set (see ) to determine whether higher BMI produces a lower percentage of children demonstrating mastery becomes available. For example, children demonstrated mastery of 23.5%, 11.6%, and 5.9% in normal, overweight, and obese BMI categories, respectively in “Gallop1” for three-year-old boys. Also, children demonstrated mastery of 38.4%, 15.4%, and 8.2% in normal, overweight, and obese categories, respectively in “Gallop1” for three-year-old girls. These findings should be able to support the finding that higher BMI results in a lower percentage of children demonstrating mastery in LMS. Similarly, children demonstrated mastery of 33.2%, 12.3%, and 4.1% in normal, overweight, and obese categories, respectively in “Strike1” for three-year-old boys. Also, children demonstrated mastery of 22.4%, 3.9%, and 8.2% in normal, overweight, and obese categories, respectively in “Strike1” for three-year-old girls. This finding might also support that higher BMI results in a lower percentage of children demonstrating mastery in OCS. Furthermore, by applying ES, despite inconsistencies, ES magnitude between normal vs. overweight/obese still be realized when comparing weight status by age in 3–5-year old children using NNYFS-2012 data. However, it seems a tendency that as children get older, weight status tends to have more impact (e.g., more positive ES [8/9 = 89%] in 5 year old compared with 3–4 yr. old [44%–66%]) in FMS (see Appendix 4).
An increase in obese and overweight children during the several past decades has been reported (Fryar et al., Citation2018). For example, an increase in obesity rate from 7.2% (1988–1994) to 13.4% (2017–2018) in 2–5-year-old children, and a 11.3% (1988–1994) to 20.3% (2017–2018) in 6–11-year-old children was reported in the U.S. (Fryar et al., Citation2018). Inverse associations between FMS and weight status emerge in children (Deforche et al., Citation2003; Gentier et al., Citation2013; Logan et al., Citation2011) and became stronger during the elementary school years (D’Hondt et al., Citation2010; Lopes et al., Citation2012). One argument is that having more mass inhibits body stabilization and force, leading to lower actual and FMS, limiting the chance of overweight/obese people participating in PA (Hume et al., Citation2008; Morgan et al., Citation2008; Southall et al., Citation2004). It has been reported that FMS insufficiency is associated with neonatal weight status (Slining et al., Citation2010). Also, in childhood, BMI was found to be a major predictor of future FMS (D’Hondt et al., Citation2013, Citation2014).
According to Barnett et al. (Citation2021), many factors could have an impact on FMS. For example, Webster et al. (Citation2019) reported that preschool children’s FMS were positively related to vigorous physical activity (VPA), but inversely related to screen-time (using questionnaire), which indicated that further about inquiry the implications of high exposure to screen-time in young children is needed. Similar cross-sectional studies (e.g., Martins et al., Citation2020; Rocha et al., Citation2021) found that excess screen time exposure was highly associated with FMS, indicating that interventions to reduce screen exposure in younger children should be a focus. All these further confirm that, to to test the Stodden’s conceptual model, longitudinal studies across
childhood and adolescence that contains all keyvariables, as well as potential confounding factors, are required (Barnett et al., Citation2021).
One advantage of this study is the vast sample size derived from normative and national research, which might reflect the child population in U.S. The number/percentage of boys and girls engaged in these studies were roughly equal when other factors were considered. The second advantage was that when comparing boys and girls in a different setting, a comparable trend pattern was detected on most occasions of the year-to-year comparisons when the data were collected. Nevertheless, limitations and disadvantages have remained in this study. To begin with, one limitation is the use of the BMI which is only an estimate of body composition, the impact of the body composition on the children’s FMS trend needs further examining. Second, because the data in this study were completely cross-sectional, it could not be utilized to establish causation. Another restriction relates to the timing of the data’s collection over the four time points. It is difficult to explain patterns over time because they were not evenly distributed, which is given the secondary analytical aspect of this study. Longitudinal study designs should be used in future research to better understand FMS trends and related determinants.
Conclusion
FMS is critical in the development and well-being of children. By analyzing the four large TGMD related data sets, it was found that U.S. children showed an increase in FMS at 3–7 years old, but a decrease/stable at 8–10 years old before the year 2000. However, children showed a slight decline/no change in FMS at 3–6 years old and a significant decline at ages 7–10 years old after 2000. Despite the children with normal BMI showed the highest FMS score, the direct cause of decreases in FMS is still unknown. More longitudinal studies with FMS-related factors and measures are needed.
What does this article add?
By analyzing the four large TGMD data sets, this study, for the first time, noticed a decrease in U.S children’s FMS after 2000. Despite the children with normal BMI showed the highest FMS score, the direct cause of decreases in FMS is still unknown. Meanwhile, other variables, e.g., screen time, physical fitness, level of PA, could also contribute the change of FMS. Given the global rise in childhood obesity, excess screen time, lower PA and physical fitness level, the decline in children’s FMS will likely be the upcoming trend. Educators thus are encouraged to create an active play and practice environment. More longitudinal studies should be conducted in the future to understand the influencing factors to children’s FMS.
Supplementary Files
Download MS Word (112.5 KB)Disclosure statement
No potential conflict of interest was reported by the author(s).
Supplementary material
Supplemental data for this article can be accessed online at https://doi.org/10.1080/02701367.2023.2250828.
Additional information
Funding
References
- Barnett, L. M., Webster, E. K., Hulteen, R. M., De Meester, A., Valentini, N. C., Lenoir, M., & Rodrigues, L. P. (2021). Through the looking glass: A systematic review of longitudinal evidence, providing new insight for motor competence and health. Sports Medicine, 52(4), 875–920. https://doi.org/10.1007/s40279-021-01516-8
- Case, L., & Yun, J. (2019). The effect of different intervention approaches on gross motor outcomes of children with autism spectrum disorder: A meta-analysis. Adapted Physical Activity Quarterly: APAQ, 36(4), 501–526. https://doi.org/10.1123/apaq.2018-0174
- Cattuzzo, M. T., dos Santos Henrique, R., Ré, A. H. N., de Oliveira, I. S., Melo, B. M., de Sousa Moura, M., de Araújo, R. C., & Stodden, D. (2016). Motor competence and health related physical fitness in youth: A systematic review. Journal of Science and Medicine in Sport, 19(2), 123–129. https://doi.org/10.1016/j.jsams.2014.12.004
- Clark, J. E. (1994). Motor development. In V. S. Ramachandran (Ed.), Encyclopedia of human behavior (3rd ed., pp. 245–255). Academic Press.
- Clark, J. E. (2005). From the beginning: A developmental perspective on movement and mobility. Quest, 57(1), 37–45. https://doi.org/10.1080/00336297.2005.10491841
- Clark, J. E., & Metcalfe, J. S. (2002). The mountain of motor development: A metaphor. In J. E. Clark & J. Humphrey (Eds.), Motor development: Research and reviews (pp. 163–190). NASPE Publications.
- Clark, J. E., & Whitall, J. (1989). What is motor development? The lessons of history. Quest, 41(3), 183–202. https://doi.org/10.1080/00336297.1989.10483969
- Cohen, J. (1988). Statistical power analysis for the behavioral sciences (2nd ed.). Lawrence Erlbaum Associates.
- Corbin, C. B., & Pangrazi, R. P. (1992). Are American children and youth fit? Research Quarterly for Exercise and Sport, 63(2), 96–106. https://doi.org/10.1080/02701367.1992.10607566
- D’Hondt, E., Deforche, B., Gentier, I., De Bourdeaudhuij, I., Vaeyens, R., Philippaerts, R., & Lenoir, M. (2013). A longitudinal analysis of gross motor coordination in overweight and obese children versus normal-weight peers. International Journal of Obesity, 37(1), 61–67. https://doi.org/10.1038/ijo.2012.55
- Deforche, B., Lefevre, J., De Bourdeaudhuij, I., Hills, A. P., Duquet, W., & Bouckaert, J. (2003). Physical fitness and physical activity in obese and nonobese Flemish youth. Obesity Research, 11(3), 434–441. https://doi.org/10.1038/oby.2003.59
- D’Hondt, E., Deforche, B., Gentier, I., Verstuyf, J., Vaeyens, R., De Bourdeaudhuij, I., Philippaerts, R., & Lenoir, M. (2014). A longitudinal study of gross motor coordination and weight status in children. Obesity, 22(6), 1505–1511. https://doi.org/10.1002/oby.20723
- D’Hondt, E., Deforche, B., Vaeyens, R., Vandorpe, B., Vandendriessche, J., Pion, J., Philippaerts, R., De Bourdeaudhuij, I., & Lenoir, M. (2010). Gross motor coordination in relation to weight status and age in 5- to 12-year-old boys and girls: A cross-sectional study. International Journal of Pediatric Obesity, 6(2–2), e556–e564. https://doi.org/10.3109/17477166.2010.500388
- Dos Santos, M. A. M., Nevill, A. M., Buranarugsa, R., Pereira, S., Gomes, T. N. Q. F., Reyes, A., Barnett, L. M., & Maia, J. A. R. (2018). Modeling children’s development in gross motor coordination reveals key modifiable determinants. An allometric approach. Scandinavian Journal of Medicine & Science in Sports, 28(5), 1594–1603. https://doi.org/10.1111/sms.13061
- Field, S. C., Esposito Bosma, C. B., & Temple, V. A. (2020). Comparability of the test of gross motor development–second edition and the test of gross motor development–third edition. Journal of Motor Learning and Development, 8(1), 107–125. https://doi.org/10.1123/jmld.2018-0058
- Fryar, C. D., Carroll, M. D., & Ogden, C. L. (2018). Prevalence of overweight, obesity, and severe obesity among children and adolescents aged 2–19 years: United States, 1963–1965 through 2015–2016. https://stacks.cdc.gov/view/cdc/58669
- Gallahue, D. L., & Donnelly, F. C. (2003). Developmental physical education for all children (4th ed.). Human Kinetics.
- Gentier, I., D’Hondt, E., Shultz, S., Deforche, B., Augustijn, M., Hoorne, S., Verlaecke, K., De Bourdeaudhuij, I., & Lenoir, M. (2013). Fine and gross motor skills differ between healthy-weight and obese children. Research in Developmental Disabilities, 34(11), 4043–4051. https://doi.org/10.1016/j.ridd.2013.08.040
- Goodway, J. D., Ozmun, J. C., & Gallahue, D. L. (2020). Understanding motor development: Infants, children, adolescents, adults (8th ed.). McGraw-Hill.
- Hardy, L. L., Barnett, L., Espinel, P., & Okely, A. D. (2013). Thirteen-year trends in child and adolescent fundamental movement skills: 1997-2010. Medicine and Science in Sports and Exercise, 45(10), 1965–1970. https://doi.org/10.1249/MSS.0b013e318295a9fc
- Harrell, F. E. (2001). Regression modeling strategies: With applications to linear models, logistic regression, and survival analysis (Vol. 608). Springer New York. https://doi.org/10.1007/978-1-4757-3462-1
- Hume, C., Bagley, S., Crawford, D., Salmon, J., Okely, A., Telford, A., & Booth, M. (2008). Does weight status influence associations between children’s fundamental movement skills and physical activity? Research Quarterly for Exercise and Sport, 79(2), 158–165. https://doi.org/10.1080/02701367.2008.10599479
- Huotari, P., Heikinaro-Johansson, P., Watt, A., & Jaakkola, T. (2018). Fundamental movement skills in adolescents: Secular trends from 2003 to 2010 and associations with physical activity and BMI. Scandinavian Journal of Medicine and Science in Sports, 28(3), 1121–1129. https://doi.org/10.1111/sms.13028
- Jenkins, D. G., Quintana-Ascencio, P. F., & Han, G. (2020). A solution to minimum sample size for regressions. PloS one, 15(2), e0229345. https://doi.org/10.1371/journal.pone.0229345
- Lima, R. A., Bugge, A., Pfeiffer, K. A., & Andersen, L. B. (2017). Tracking of gross motor coordination from childhood into adolescence. Research Quarterly for Exercise and Sport, 88(1), 52–59. https://doi.org/10.1080/02701367.2016.1264566
- Logan, S. W., Robinson, L. E., Wilson, A. E., & Lucas, W. A. (2012). Getting the fundamentals of movement: A meta-analysis of the effectiveness of motor skill interventions in children. Child: Care, Health and Development, 38(3), 305–315. https://doi.org/10.1111/j.1365-2214.2011.01307.x
- Logan, S. W., Ross, S. M., Chee, K., Stodden, D. F., & Robinson, L. E. (2018). Fundamental motor skills: A systematic review of terminology. Journal of Sports Sciences, 36(7), 781–796. https://doi.org/10.1080/02640414.2017.1340660
- Logan, S. W., Scrabis-Fletcher, K., Modlesky, C., & Getchell, N. (2011). The relationship between motor skill proficiency and body mass index in preschool children. Research Quarterly for Exercise and Sport, 82(3), 442–448. https://doi.org/10.1080/02701367.2011.10599776
- Lopes, L., Santos, R., Coelho-E-Silva, M., Draper, C., Mota, J., Jidovtseff, B., Clark, C., Schmidt, M., Morgan, P., Duncan, M., O’Brien, W., Bentsen, P., D’Hondt, E., Houwen, S., Stratton, G., De Martelaer, K., Scheuer, C., Herrmann, C., García-Hermoso, A., and Agostinis-Sobrinho, C. (2021). A narrative review of motor competence in children and adolescents: What we know and what we need to find out. International Journal of Environmental Research and Public Health, 18(1), 1–20. https://doi.org/10.3390/ijerph18010018
- Lopes, V. P., Stodden, D. F., Bianchi, M. M., Maia, J. A., & Rodrigues, L. P. (2012). Correlation between BMI and motor coordination in children. Journal of Science and Medicine in Sport, 15(1), 38–43. https://doi.org/10.1016/j.jsams.2011.07.005
- Martins, C. M. L., Bandeira, P. F. R., Lemos, N. B. A. G., Bezerra, T. A., Clark, C. C. T., Mota, J., & Duncan, M. J. (2020). A network perspective on the relationship between screen time, executive function, and fundamental motor skills among preschoolers. International Journal of Environmental Research and Public Health, 17(23), 8861. https://doi.org/10.3390/ijerph17238861
- Morgan, P. J., Okely, A. D., Cliff, D. P., Jones, R. A., & Baur, L. A. (2008). Correlates of objectively measured physical activity in obese children. Obesity, 16(12), 2634–2641. https://doi.org/10.1038/oby.2008.463
- NSW Department of Education and Training. (2000). Get skilled get active: A K-6 resource to support the teaching of fundamental movement skills.
- Pate, R., Oria, M., & Pillsbury, L. (2012). Committee on fitness measures and health outcomes in youth, food and nutrition board & institute of medicine (Eds.). In Fitness measures and health outcomes in youth (pp. 71–72). National Academies Press (US).
- Peduzzi, P., Concato, J., Kemper, Z., Holford, T. R., & Feinstein, A. R. (1996). A simulation study of the number of events per variable in logistic regression analysis. Journal of Clinical Epidemiology, 49(12), 1373–1379. https://doi.org/10.1016/S0895-4356(96)00236-3
- Reyes, A. C., Chaves, R., Baxter-Jones, A. D. G., Vasconcelos, O., Barnett, L. M., Tani, G., Hedeker, D., & Maia, J. (2019). Modelling the dynamics of children’s gross motor coordination. Journal of Sports Sciences, 37(19), 2243–2252. https://doi.org/10.1080/02640414.2019.1626570
- Robinson, L. E., & Goodway, J. D. (2009). Instructional climates in preschool children who are at-risk. Part I: Object-control skill development. Research Quarterly for Exercise and Sport, 80(3), 533–542. https://doi.org/10.1080/02701367.2009.10599591
- Rocha, H. A. L., Correia, L. L., Leite, Á. J. M., Machado, M. M. T., Lindsay, A. C., Rocha, S. G. M. O., Campos, J. S., Cavalcante E Silva, A., & Sudfeld, C. R. (2021). Screen time and early childhood development in Ceará, Brazil: A population-based study. BMC Public Health, 21(1), 2072. https://doi.org/10.1186/s12889-021-12136-2
- Roth, K., Ruf, K., Obinger, M., Mauer, S., Ahnert, J., Schneider, W., Graf, C., & Hebestreit, H. (2010). Is there a secular decline in motor skills in preschool children? Scandinavian Journal of Medicine and Science in Sports, 20(4), 670–678. https://doi.org/10.1111/j.1600-0838.2009.00982.x
- Slining, M., Adair, L. S., Goldman, B. D., Borja, J. B., & Bentley, M. (2010). Infant overweight is associated with delayed motor development. Journal of Pediatrics, 157(1), 20–25. https://doi.org/10.1016/j.jpeds.2009.12.054
- Southall, J. E., Okely, A. D., & Steele, J. R. (2004). Actual and perceived physical competence in overweight and nonoverweight children. Pediatric Exercise Science, 16(1), 15–24. https://doi.org/10.1123/pes.16.1.15
- Stodden, D. F., Langendorfer, S. J., Goodway, J. D., Roberton, M. A., Rudisill, M. E., Garcia, C., & Garcia, L. E. (2008). A developmental perspective on the role of motor skill competence in physical activity: An emergent relationship. Quest, 60(2), 290–306. https://doi.org/10.1080/00336297.2008.10483582
- Ulrich, D. A. (1985). Test of gross motor development. Pro-Ed.
- Ulrich, D. A. (2000). Test of gross motor development (2nd ed.). Pro-Ed.
- Ulrich, D. A. (2017). Introduction to the special section: Evaluation of the psychometric properties of the TGMD-3. Journal of Motor Learning and Development, 5(1), 1–4. https://doi.org/10.1123/jmld.2017-0020
- Ulrich, D. A. (2019). Test of gross motor development (3rd ed.). Pro-Ed.
- Walkley, J., Holland, B. V., Treloar, R., & O’Connor, J. (1996). Fundamental motor skills: A manual for classroom teachers. Department of Education.
- Webster, E. K., Martin, C. K., & Staiano, A. E. (2019). Fundamental motor skills, screen-time, and physical activity in preschoolers. Journal of Sport and Health Science, 8(2), 114–121. https://doi.org/10.1016/j.jshs.2018.11.006
- Zimmer, R., & Volkamer, M. (1987). Motoriktest für vier- bis sechsjährige kinder : mot 4-6; manual (2., überarb. und erw. Aufl). Beltz-Tes.