Abstract
Fourteen lakes, situated in the Borecka Forest, Poland and including 8 dimictic and 6 polymictic lakes, were investigated over a period of 10 years. The water bodies were at low risk from eutrophication. A positive and statistically significant correlation between total phosphorus (TP) and total nitrogen (TN) concentrations and chlorophyll levels was reported in all of the studied lakes. The results of the study validate the hypothesis that aquatic vegetation in lakes may have a delayed response to nutrient supply because the phosphorus delivered to and present in lakes may be partially unavailable for producers. Redundancy analysis (RDA) results revealed a significant correlation between relative plant abundance and chlorophyll concentrations and a weaker relationship with the remaining lake parameters (TP, Secchi depth, TN). The positive correlation between chlorophyll and seston concentrations was significant only in dimictic lakes (r = 0.62, p = 0.001). In polymictic water bodies, chlorophyll levels decreased with Secchi depth, whereas chlorophyll concentrations in dimictic lakes varied irregularly with an increase in water transparency. The correlation between seston and Secchi depth was significant in all lakes. Seston distribution in response to a drop in water transparency did not differ significantly between the lake types. The analyzed parameters supported the identification of two groups of lakes which correspond to habitat types of the Natura 2000 network.
Introduction
The main drivers of macrophyte abundance in lakes are water chemistry (Beal Citation1977; Hoyer and Canfield Citation1996; Grzybowski et al. Citation2010a, Citation2010b), trophic state (Spence Citation1967; Canfield et al. 1983; Bini et al. Citation1999; Kolada Citation2010; Kowalczewska-Madura and Gołdyn Citation2012), and light availability (Canfield et al. Citation1985). Macrophytes affect the physical, chemical, and biological conditions of lakes and they reflect the impact of various environmentalfactors such as lake morphometry, water chemistry, and biotic interactions (Lacoul and Freedman Citation2006; Cheruvelil and Soranno Citation2008; Kolada Citation2010).
The relationships between abiotic habitat and macrophyte abundance in aquatic ecosystems have been well researched (Rørslett Citation1991; Grzybowski et al. Citation2005; Grzybowski and Endler Citation2008; Kowalczewska-Madura and Gołdyn Citation2012). Despite some controversy (e.g., Linton and Goulder Citation2000), the presence of positivecorrelations between lake area and biological diversity can be regarded as an important indicator of aquatic plant status (Møller and Rørdam Citation1985; Gee et al. Citation1997; Jeffries Citation1998; Oertli et al. Citation2002). The physicochemical properties of water and substrate as well as the availability of light in the habitat influence the distribution patterns of aquatic vegetation (Hakanson and Boulion Citation2002; Søndergaard et al. Citation2005, Citation2010).
The mechanisms regulating nutrient cycling differ between dimictic and shallow polymictic lakes and nutrient cycling can influence macrophyte colonization patterns. The vegetation of shallow lakes is a robust indicator of their environmental status (biotope–biocenosis relationship) and it also modifies the lake ecosystem. Macrophytes colonize up to 100% of the area of polymictic lakes, whereas in dimictic lakes, plant distribution is limited to the littoral zone, which is referred to as the phytolittoral zone. Thus, the impact of macrophytes in deep, dimictic lakes is less than in shallow polymictic lakes (Rounsefell Citation1946; Tilzer and Serruya Citation1990; Ciecierska Citation2008). Plants also exert powerful stabilizing effects on shallow water bodies (biocenosis–biotope), in particular in ecosystems where the vegetated zone covers the entire lake basin. Many authors have observed that, unlike in dimictic (deep) lakes, macrophytes have habitat-forming effects in shallow polymictic water bodies (Scheffer et al. Citation1993; Jeppesen et al. Citation1998; Scheffer Citation1998; Scheffer and van Nes Citation2007). These differences between polymictic and dimictic lakes were used to evaluate the ecological status of Polish lakes (under the Framework Water Directive) based on macrophyte characteristics (Kolada Citation2009).
Early models that relied on chlorophyll and phosphorus concentrations in lake water were simple interpretations of Liebig's Law of the Minimum. The first productivity model (Sakamoto Citation1966; Dillon and Rigler Citation1974; Carlson Citation1977) demonstrated a linear correlation between those variables. Chlorophyll–nutrient relationships were further examined by McCauley et al. Citation(1989) who identified a series of non-linear correlations resulting from the modifying role of environmental factors (for example, chlorophyll concentrations in shallow lakes may be limited by nutrient availability or light, depending on the contradictory role of resuspended particles and other factors). This phenomenon is frequently observed in nature (Scheffer et al. Citation2001; Dent et al. Citation2002; McClanahan et al. Citation2002; Foley et al. Citation2003), in particular in shallow polymictic freshwater ecosystems (Scheffer et al. Citation1993; Hosper Citation1998; Kufel Citation1999, Citation2001). Rørslett Citation(1991) performed a comprehensive review of literature relating to aquatic vegetation in 622 Scandinavian lakes across a broad trophic spectrum and observed that the main prognostic indicators of macrophyte species richness were lake area, elevation, trophic state, and several variables describing water quality. The above findings were validated by Murphy Citation(2002). It should be noted, however, that the aforementioned study focused on soft-water lakes, which are lime-deficient water bodies characterized as oligo-eutrophic to meso-eutrophic and with low to circumneutral pH. A broad range of species can usually be supported in these lakes, including plants more characteristic of intermediate-water to hard-water lakes (Murphy Citation2002), but local conditions may play modifying roles in influencing actual outcomes in individual lakes (Murphy Citation2002). A lack of resuspension and negligible macrophyte impact on nutrients in deep, dimictic lakes should result in different chlorophyll–nutrient and chlorophyll–seston relationships in dimictic and polymictic lakes.
Major threats to the survival of soft-water lake vegetation in northern Europe include acidification, eutrophication, increased recreational use of the lakes, and the effects of lake regulation for hydroelectric power (Murphy Citation2002). Such pressures cause changes in the functioning of lake ecosystems (Kolada Citation2010). Environmental pressures affect a growing number of water bodies whose behavior is vital for the preservation of habitat diversity in Europe (Murphy Citation2002). Rapid environmental changes observed in recent years necessitate regular evaluations of ecosystems, including observations of their present state and deviations from reference conditions expected with minimal or no anthropogenic pressure. This method is known as the reference condition approach (RCA) and it has been promoted since the 1990s mainly in the USA (Karr Citation1991; Bailey et al. Citation2004) and Great Britain (Wright Citation1995; Wright et al. Citation2000). RCA has significant practical implications in Europe in the process of implementing the provisions of the Framework Water Directive (EC 1992; Penning et al. Citation2008a, Citation2008b). The obtained results can be used to establish reference conditions for lakes. The lakes of the Borecka Forest, Poland, that were analyzed in this study were characterized by minimal anthropogenic influence and low trophic state. Their status can be attributed to the low level of urbanization in the catchment area, absence of point source pollution and supportive hydrological conditions which prevent the accumulation of biogenic substances in lakes.
The aim of this study was to determine differences and similarities in chlorophyll–nutrient–Secchi depth–aquatic macrophyte relationships between dimictic and polymictic lakes. The ability of the predictors to differentiate between natural lake types and their usefulness in identifying Natura 2000 habitats were also examined. The above correlations were analyzed in a long-term study of lakes in the Borecka Forest.
Methods
The study region is situated in the Borecka Forest (N 54º07.31’, E 22º08.54’) in the northeastern part of the Masurian Lakeland in Poland. This large forest complex occupies an area of 25,340.1 ha and is characterized by low anthropogenic pressure. Agriculture and forestry represent the main types of human activity in the region. Tourism pressure is low and there is an absence of industrial plants and cities in the vicinity. All of the 14 analyzed lakes (, ) are protected habitats of the Natura 2000 network (SDF Citation2008). The studied lakes have a regular shape (ratio of maximum length to maximum width < 6.0). Their catchment areas consist mainly of farmland and forests (eight lakes) or mainly forests (six lakes).
Table1. Morphometric and trophic characteristics of the sampled lakes.
Figure1. Geographical distribution of the studied lakes in the Borecka Forest; lake types are marked with numbers; dimictic lakes: 1 – Zabinki, 2 – Krzywa Kuta, 3 – Lekuk, 4 – Litygajno, 5 – Lazno, 6 – Szwalk Maly, 7 – Szwalk Wielki, 8 – Wolisko; polymictic lakes: 9 – Biala Kuta, 10 – Smolak, 11 – Kacze, 12 – Dubinek, 13 – Pilwag, 14 – Ciche.
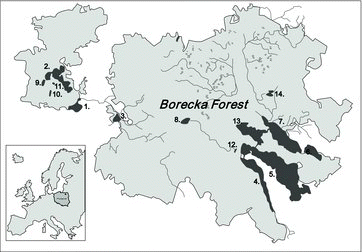
Water samples were collected at a depth of 1 m from dimictic and polymictic lakes twice a year during the summer stagnation period () from the central part of the thermocline region, which coincided with the period of macrophyte studies. Three samples were collected each time and the results are the means of three replicates. Water transparency (SD, in meters) was measured with the Secchi disc. Physical and chemical analyses of water samples were performed by standard methods (Standard Methods Citation1999). Total phosphorus (TP), total nitrogen (TN), and iron concentration were determined colometrically using the Shimadzu UV 1601 spectrophotometer. Chlorophyll a was analyzed by the acetone extraction method (Golterman Citation1969) and corrected for pheophytin. A specified amount of lake water was filtered through preweighed Whatman GF/C filters, and seston concentrations were determined after drying the filters at 105°C to a constant weight.
Aquatic vegetation was studied from 2000 to 2010 and the results are presented in . The study involved inventories of plant communities (phytosociological surveys), floristic assessments, and mapping of plant colonies in the littoral zone. It was carried out at the peak of every growing season (from the second half of June to the end of August) and the results were analyzed using mean values reported for the 10-year period of the study.
Table2. Diversity indices of aquatic vegetation in two types of lakes.
Macrophyte data were gathered at species level and indicator species were identified in the analyzed communities. The following parameters were determined: maximum colonization depth, total colonized area, and percentage share of every identified association in the total phytolittoral area. Plant communities were classified based on a syntaxonomic system proposed by Braun-Blanquet Citation(1964), which is consistent with the International Code of Phytosociological Nomenclature (Weber et al. Citation2000). The system has been adapted to Polish conditions (Brzeg and Wojterska Citation2001; Matuszkiewicz Citation2002). Further analyses were carried out based on the identified plant communities belonging to higher syntaxonomic units in line with the methodology proposed by Ciecierska Citation(2008). The following is the list of plant associations covered: Magnocaricion – 15 associations, Phragmition – 10 associations, Charetea – 6 associations, Potamion – 18 associations, Lemnetea – 2 associations, Utricularietea intermedio–minoris – 1 association.
The relationships between aquatic vegetation and environmental factors were examined based on abundance, phytocenotic diversity, and relative macrophyte abundance (. The abbreviations applied in are used further in the text. The phytocenotic diversity index (H) was calculated based on the Shannon-Weaver index:
where, ni is the area occupied by the ith plant community and N is the total area of the phytolittoral zone.
Pielou's evenness index (J) was calculated as the ratio of actual diversity to maximum diversity (Magurran Citation1988). Diversity indices were determined using the MultiVariate Statistical Package version 3.1 (KCS Citation2007).
The collected dataset was analyzed statistically to determine the minimum and maximum values, arithmetic mean, and standard deviation. The parameters describing dimictic and polymictic were compared with the use of a t-test. Data were processed in the Statistica For Windows Package version 11 (StatSoft Inc. Citation2011). Regression coefficients were determined in successive analyses.
A preliminary detrended correspondence analysis (DCA) revealed the first gradient length of 1.38 standard deviations, indicating that models based on a linear species response were appropriate for the data structure (Ter Braak and Šmilauer Citation2002; Lepš and Šmilauer Citation2007).
The contemporary aquatic macrophyte community in the 14 lakes was explored using redundancy analysis (RDA, Van den Wollenberg Citation1977), a constrained form of principal component analysis (Hotelling Citation1933) in CANOCO (Ter Braak and Šmilauer Citation2002). Chemical parameters were log transformed to meet the assumptions of normality. For the RDA, a split plot design was used, with each sampling year representing a split plot and each lake representing a whole plot. Statistical significance tests were carried out using Monte Carlo permutation tests.
Results
Summer average TP concentrations in the studied ecosystems were lower in dimictic lakes (0.051 ± 0.035 mg/L) than in polymictic lakes (0.059 ± 0.064 mg/L) (t = −3.073, p = 0.003; ). The average values of TN concentrations were also lower in dimictic lakes (1.71 ± 0.77 mg/L) in comparison with polymictic water bodies (1.87 ± 0.73 mg/L) (t = −2.91, p < 0.01; ). The average TN:TP ratio in the epilimnion was higher in shallow lakes (54) than in dimictic lakes (38, t = −2.575, p < 0.02).
Chlorophyll a concentrations, seston content, and Secchi depth are indicators of a lake's trophic state (Carlson Citation1977; Kufel Citation2001). Chlorophyll a concentrations differed between the dimictic and polymictic lakes. Average chlorophyll levels were 23 ± 0.77 μg/L in dimictic lakes and 18.9 ± 0.73 μg/L in polymictic lakes (t = −3.079, p = 0.002; ).
The correlation between summer epilimnetic chlorophyll a levels and TP concentrations was significant in all of the lakes (; log Chl = 3.4075 + 1.726 * log TP, r = 0.6, p < 0.001). The above relationship was noted in both dimictic (r = 0.46, p < 0.02) and polymictic lakes (r = 0.76, p < 0.001). This correlation was weaker in dimictic lakes and this could explain the low variation in chlorophyll concentration, which could point to the presence of a limiting factor in this group of lakes.
Figure2. The regression between Chl a and TP (log Chl = 3.4075 + 1.726 * log TP) was significant for summer epilimnetic concentrations in Borecka Forest lakes: dimictic lakes (dots); polymictic lakes (circles).
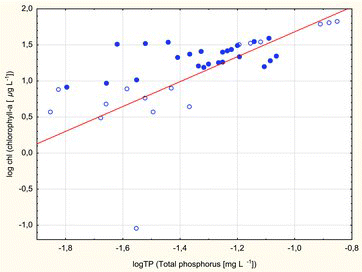
The correlation between summer epilimnetic chlorophyll a concentrations and TN was also significant in all lakes (; log Chl = 0.4309 + 2.6789 * log TN, r = 0.44, p < 0.0035). This dependency was observed in dimictic (r = 0.76, p < 0.00002) as well as in polymictic lakes (r = 0.76, p < 0.0002) and it suggests a linear relationship between chlorophyll a and nutrient concentrations. In both lake types, chlorophyll a seems to have responded positively to changes in phosphorus and nitrogen concentrations. Changes in chlorophyll levels do not rule out limited nutrient availability.
Figure3. The regression between Chl a and TN (log Chl = 0.4309 + 2.6789 * log TN) was significant for summer epilimnetic concentrations in Borecka Forest lakes: dimictic lakes (dots); polymictic lakes (circles).
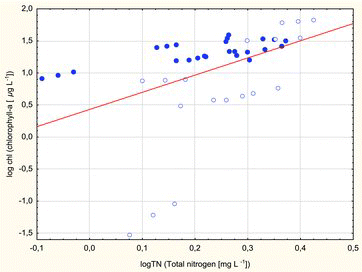
The correlation between chlorophyll levels and seston content in all the lakes was not significant. A significant linear correlation was determined between chlorophyll and seston concentrations in dimictic lakes (r = 0.62, p = 0.001). Chlorophyll a varied more irregularly with an increase in the seston content of polymictic lakes. Significant variations in those correlations are shown in . When the relatively high seston content of polymictic lakes was accompanied by lower chlorophyll concentrations, a higher contribution of detritus and/or mineral particles to seston should be expected. In cases where the high seston content of polymictic lakes was accompanied by high chlorophyll levels, a higher share of algae in the seston should be expected, which is characteristic of dimictic water bodies, but less frequently noted in the discussed type of lakes.
Figure4. Significant correlation between chlorophyll and seston concentrations in dimictic lakes (dots, r = 0.62, p = 0.001). Data from polymictic lakes (circles) point to an irregular and usually lower contribution of chlorophyll to seston in those lakes.
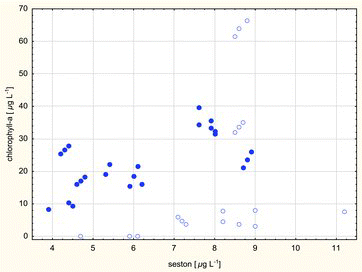
Significant correlations between log SD and log Chl were not obtained for the combined lakes. The correlation between log SD and log Chl was significant only in polymictic lakes (log SD = −0.1368–0.1343 * log Chl, r = −0.71, p = 0.0009), whereas in dimictic lakes, chlorophyll varied irregularly with an increase in water transparency (). In many lakes, a constant relationship can be derived between estimates of light penetration and SD (Megard et al. Citation1980). This approach has certain weaknesses. SD does not account for variability in surface light conditions over time and seasonal variations in water transparency. Secchi depth is not consistently correlated with light exposure; it is not a direct measure of the amount of light energy used by photosynthesizing organisms; and it is prone to variability in vision between observers. The above limitations could have contributed to the ambiguity of correlations between SD and chlorophyll a concentrations (). Other researchers pointed to limitations associated with the above correlations, in particular in lakes with high concentrations of non-algal material in the water column (Brezonik Citation1978; Megard et al. Citation1980; Lind Citation1986) or large macrophyte populations (Canfield et al. Citation1983).
Figure5. Regression between log SD and log Chl a in dimictic lakes (dots) and data from polymictic lakes (circles). Logarithmic regression between SD and chlorophyll was significant only in polymictic lakes (r = –0.71, p = 0.0009).
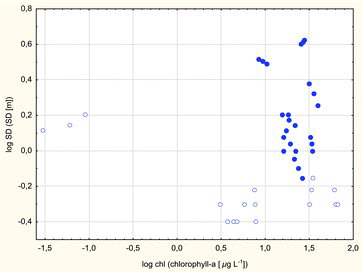
Logarithmic regression between SD and seston concentration was significant in all of the investigated lakes (; log SD = 1.6038–1.9131 * log seston; r = −0.7513; p < 0.001). The changes in SD (visibility) in response to seston concentrations (slope of regression line) did not differ significantly between the two lake groups, and differences in the optical properties of suspended particles (mainly of algal origin in dimictic lakes and with variable admixtures of detritus/mineral components in polymictic lakes) were not accentuated in the distribution of variables (r = −0.65, p < 0.0007 in dimictic lakes; r= −0.67, p < 0.0025 in polymictic lakes).
Figure6. Regression between log SD and log seston (solid line, log SD = 1.6038–1.9131 * log seston; r = –0.751; p < 0.001) in dimictic lakes (circles) and data from polymictic lakes (dots).
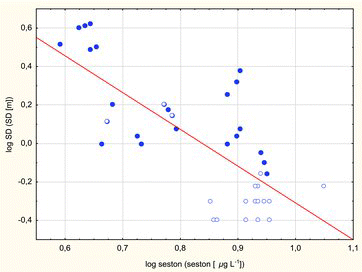
Macrophyte indicators differed between the studied lake groups. The average value of Shannon's index (H) in dimictic lakes reached 1.3 ± 0.62 in dimictic lakes and 1.75 ± 0.73 in polymictic lakes (t = −5.74, p < 0.001; ), and the hydrophyte/helophyte ratio was 2.03 ± 1.1 and 13.08 ± 24.2 in dimictic and polymictic water bodies, respectively (t = −2.713, p = 0.02; ).
No significant correlations were reported between the distribution of SD values and hydrophyte/helophyte parameter values. The distribution of H:SD variables produced significant correlations in the entire collection of examined lakes (r = −0.48, p = 0.0015), but this relationship was not reported in the analyses performed separately for each group of lakes.
The first and second ordination axes explained approximately 39% of variation in structural features (gradient lengths of 0.282 and 0.106) and 90.8% of variation in the relationships between vegetation features and environmental parameters. Of the variables correlated with the first canonical axis, the longest vectors were chlorophyll a and TP, whereas TN and SD were less significant (). The significance of the first canonical axis was tested to reveal a significant gradient that determined differences among the studied lakes (p = 0.05). The first axis was strongly correlated with chlorophyll a (0.8) and TP (0.6), and was weakly correlated with SD (0.4) and TN (0.3). Chlorophyll a (λ = 0.24, p = 0.002, F = 3.85) was a statistically significant parameter (Monte Carlo permutation test, p < 0.05), which explained 9.3% of total variation in the examined lakes.
Figure7. RDA ordination diagrams for dimictic lakes (dots) and polymictic lakes (circles); coverage of analyzed communities: %Chara – Class (Cl.) Charetea; %Pata – Alliance (All.) Potamion; %Nymph – All. Nymphaeion; %Phra – All. Phragmition; %Mcar – All. Magnocaricion; %Lemn – Cl. Lemnetea; %Utric – Cl. Utricularietea intermedio-minoris; these abbreviations are also used in the text.
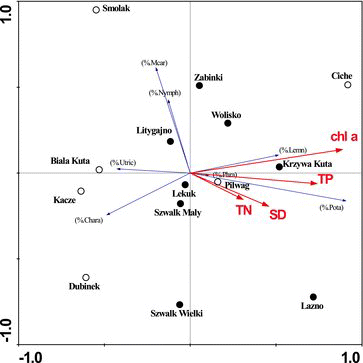
Discussion
Summer average total nutrient concentrations were higher in polymictic lakes than in dimictic water bodies. Phosphorus and nitrogen probably accumulated mostly in bottom sediments as has been validated by other researchers (Wetzel Citation2001). The larger the area of bottom sediments which are stirred by wave motion, the more nutrients reach the pelagic zone as a result of mixing (spring and autumn circulation), air movement, and convection. In polymictic lakes, which mix many times a year, phosphorus and nitrogen are released from the sediments into water at a faster rate than in dimictic lakes which undergo mixing twice a year.
The linear correlation between chlorophyll a concentrations and nutrient levels in these polymictic and dimictic lakes is indicative of a good qualitative and quantitative status of the studied ecosystems. This particularly applies to polymictic lakes where the uncoupling of chlorophyll a and nutrient concentrations is not unusual (Dokulil and Padisak Citation1994; Scheffer Citation1998; Kufel Citation1999). Nixdorf and Deneke Citation(1997) and Kufel Citation(1999) suggested that P utilization (Chl:TP ratio) increases with a decrease in lake depth. Their observations are confirmed by the findings of our study of the Borecka Forest lakes. The mean summer ratio of chlorophyll:particulate P differed between dimictic (0.50 μg/μg) and polymictic lakes (0.28 μg/μg). The reported values are lower than those presented by Pridmore et al. Citation(1989) and Kufel Citation(1998). Differences were also reported in chlorophyll: particulate N ratios, which reached 13.4 μg/mg and 8.5 μg/mg on average in dimictic and polymictic lakes, respectively. Variations in phosphorus utilization and significantly lower chlorophyll a concentrations in polymictic lakes imply that primary producers are controlled by mechanisms which lead to nutrient limitation.
RDA supported the classification of the analyzed lakes into groups. The ordination diagram clearly identifies a group of soft-water, dystrophic, oligohumic lakes with calcium content below 25 mg/L in a different study (Grzybowski, unpublished data). The parameters of lakes Smolak, Biała Kuta, Kacze, and Dubinek are characteristic of mesotrophic brown-water lakes, whereas the remaining water bodies were classified into a group of eutrophic lakes identified by Toivonen and Huttunen Citation(1995). These authors reported the results of a survey of 57 small lakes (0.5–200 ha) in relation to ecological gradients under different environmental conditions and concluded that trophic state and water transparency were the strongest determinants of euhydrophyte species occurrence. Numerous authors have argued that aquatic vegetation in lakes may have a delayed response to nutrient supply because the phosphorus delivered to and present in lakes maybe partially unavailable for producers (Zdanowski Citation1982; Hillbricht-Ilkowska Citation1993; Hillbricht-Ilkowska and Wiśniewski Citation1993; Kufel Citation1999; Ciecierska Citation2008). This concept explains the strong correlation between relative plant abundance and chlorophyll a concentrations and a weaker relationship with the remaining lake parameters. Despite the above, a significant correlation between nutrient concentrations (TP and TN) and chlorophyll levels suggests that those parameters are important drivers of relative plant abundance in these lakes. Despite significant differences between the parameters characterizing the analyzed dimictic and polymictic lakes (TP, TN, Chl, H, hydrophyte/helophyte), RDA failed to differentiate between groups of dimictic and polymictic lakes ().
All of the studied lakes (14) were classified as habitats of the Natura 2000 network based on Habitat Directive criteria (EC Citation1992; CID Citation2011). The main criterion for classifying water bodies as Natura 2000 ecosystems is the types of aquatic vegetation (plant communities) in the lakes. The following lake groups were identified based on RDA results: natural eutrophic lakes with Magnopotamion or Hydrocharition-type vegetation (Natura 2000 code 3150–1: lakes Litygajno, Żabinki, Szwalk Maly, Lekuk, Szwalk Wielki, Wolisko, Krzywa Kuta, and Lazno; Natura 2000 code 3150-2: lakes Pilwag and Ciche), hard oligo-mesotrophic waters with benthic vegetation of Chara spp. (Natura 2000 code 3140: lakes Biala Kuta and Dubinek), and natural dystrophic lakes and ponds (Natura 2000 code 3160: lakes Smolak and Kacze).
Hard oligo-mesotrophic waters with benthic vegetation of Chara spp. (Biala Kuta and Dubinek) were characterized by low phosphorus concentrations (). Dense stonewort meadows act as nutrient traps by blocking internal sources of biogenic substances, limiting sediment resuspension, and impairing phytoplankton growth (Van den Berg et al. Citation1998, Citation2003; Van den Berg Citation1999; Kufel and Kufel Citation2002). The catchments of the two dystrophic lakes (Smolak and Kacze) are largely occupied by raised bogs (<70%) which are a source of humic substances. Most humic substances dissolved in surface water originate from the catchment (Wilkinson and Negre Citation1997) and only a small fraction are autochthonous (De Haan Citation1992; Wetzel Citation2001). In general, humic substances stain water, affect thequality and quantity of light in aquatic ecosystems, increase sedimentation, bind calcium,nitrogen and phosphorus, and neutralize oligotrophic and acidic water bodies (SzmejaCitation2000). Dissolved humic substances support the formation of complex phosphorus compounds (De Haan Citation1992) and they accelerate and stabilize calcite precipitation. In acidic environments, humic substances limit the solubility of silica (Otsuki and Wetzel Citation1974; Conzonno and Cirelli Citation1995) and similar activity is observed in lakes colonized by stonewort meadows (Kufel and Kufel Citation2002). Humic substances react with water and sediment components to regulate the quantity and availability of nutrients for plants (Banaś Citation2002, Citation2005). The above mechanisms explain the RDA results reported in this study.
The results of our analyses () point to the importance of the plant community criterion in identifying habitats of the Natura 2000 network (3140, 3150, 3160). The implementation of the Framework Water Directive (Penning et al. Citation2008a, Citation2008b) gave rise to numerous methods for evaluating the ecological condition of water bodies based on macrophyte indicators. Those methods are widely used to monitor the health of aquatic ecosystems across the EU. The efforts aimed at implementing a water management system compliant with the provisions of the Framework Water Directive are carried out independently of measures supporting the management of habitats (including lakes and dependent ecosystems) protected under the Natura 2000 network. Lake analyses relying on the RCA should account for the water bodies which are part of the Natura 2000 network despite the fact that those habitats have not been investigated in the water management systems based on macrophyte indicators of the lakes’ ecological condition (Penning et al. Citation2008a, Citation2008b; Kolada Citation2010). The results of this study fill this gap and indicate that the water management system should be integrated with nature conservation principles.
Acknowledgements
The study was supported by the University of Warmia and Mazury. Special thanks go to Julita Dunalska for providing the hydrochemical data from littoral zone on studied lakes in year 2010. I also thank the constructive comments of Jeffrey A Simmons and two anonymous Reviewers.
References
- Bailey RC, Norris RH, Reynoldson TB. 2004. Bioassessment of freshwater ecosystems using the reference condition approach. Boston: Kluwer Academic Publishers.
- Banaś K. 2002. Impact of humic substances on Sphagnum denticulatum habitats. Acta Societatis Botanicorum Poloniae. 71:63–69.
- Banaś K. 2005. The effect of dissolved organic carbon on pelagial and near-sediment water traits in lakes. Acta Societatis Botanicorum Poloniae. 74:133–139.
- Beal EO. 1977. A manual of marsh and aquatic vascular plants of North Carolina with habitat data. Technical Bulletin No. 247. Raleigh (NC): North Carolina Agricultural Research Service.
- Bini LM, Thomaz SM, Murphy KJ, Camargo AFM. 1999. Aquatic macrophyte distribution in relation to water and sediment conditions in the Itaipu reservoir, Brazil. Hydrobiologia. 415:147–154.
- Braun-Blanquet J. 1964. Pflanzensoziologie. New York: Springer.
- Brezonik PL, 1978. Effect of organic color and turbidity on Secchi disk transparency. Journal of the Fisheries Research Board of Canada. 35:1410–1416.
- Brzeg A, Wojterska M. 2001. Plant communities in Wielkopolska: the state of knowledge and threats. In: Wojterska M, editor. Flora and vegetation of Wielkopolska and South-Pomerania Lakeland. Guide book of 52. Poznan: Reunion of Polish Botanical Society. p. 39–110.
- Canfield DE Jr, Langeland KA, Linda SB, Haller W. 1985. Relations between water transparency and maximum depth of macrophyte colonization. Journal of Aquatic Plant Management. 23:25–28.
- Canfield DE Jr, Langeland KA, Maceina MJ, Haller WT, Shireman JV, Jones JR. 1983. Trophic state classification of lakes with aquatic macrophytes. Canadian Journal of Fisheries and Aquatic Sciences. 40:1713–1718.
- Carlson RE. 1977. A trophic state index for lakes. Limnology and Oceanography. 22:361–369.
- Cheruvelil KS, Soranno PA. 2008. Relationships between lake macrophyte cover and lake and landscape features. Aquatic Botany. 88:219–227.
- CID. 2011. Commission Implementing Decision of 11 July 2011 concerning a site information format for Natura 2000 sites, (notified under document C(2011) 4892) (2011/484/EU). Official Journal of the European Union. L 198:39–70.
- Ciecierska H. 2008. Macrophyte-based indices of the ecological state of lakes [ dissertation and monographs]. [Olsztyn (Poland)]: University of Warmia and Mazury. ( Polish with english summary).
- Conzonno V, Cirelli AF. 1995. Dissolved organic matter in Chascomus Pond (Argentina). Factors influencing distribution and dynamics. Hydrobiologia. 297:55–59.
- De Haan H. 1992. Impact of environmental changes on the biogeochemistry of aquatic humic substances. Hydrobiologia. 229:59–71.
- Dent CL, Cumming GS, Carpenter SR. 2002. Multiple states in river and lake ecosystems. Philosophical Transactions of the Royal Society of London Series B – Biological Sciences. 357:635–645.
- Dillon PJ, Rigler FH. 1974. The phosphorus–chlorophyll relationships in lakes. Limnology and Oceanography. 19:767–773.
- Dokulil MT, Padisak J. 1994. Long-term compositional response of phytoplankton in a shallow, turbid environment, Neusiedlersee (Austria/Hungary). Hydrobiologia. 275/276:125–137.
- EC. 1992. Council directive 92/43/EEC of 21 May 1992 on the conservation of natural habitats and of wild fauna and flora. Official Journal of the European Union. L 206:7–50
- Foley JA, Coe MT, Scheffer M, Wang GL. 2003. Regime shifts in the Sahara and Sahel: interactions between ecological and climatic systems in northern Africa. Ecosystems. 6:524–539.
- Gee JHR, Smith BD, Lee KM, Griffths SW. 1997. The ecological basis of freshwater pond management for biodiversity. Aquatic Conservation: Marine and Freshwater Ecosystem. 7:91–104.
- Golterman HL. 1969. Methods for chemical analysis of fresh waters. Oxford (England): Blackwell Scientific Publications.
- Grzybowski M, Endler E. 2008. The state of plant conservation in small water bodies located within the city limits of Olsztyn. Oceanological and Hydrobiological Studies. 37(3):73–83.
- Grzybowski M, Szarek J, Skibniewska K, Guziur J. 2010a. Ecological status and phytocoenotic diversity of macrophytes of lake Szeląg Wielki (north-east Poland). Polish Journal of Natural Sciences. 25(4):401–414.
- Grzybowski M, Szarek J, Skibniewska K, Guziur J. 2010b. Evaluation of diversity of submerged and emergent flora of lake Szeląg Wielki as threatened by a pesticide tomb. Polish Journal of Natural Sciences. 25(4):154–172.
- Grzybowski M, Szarek J, Skibniewska K, Sawicka-Kapusta K, Guziur J, Endler Z. 2005. The characteristics of plants in the littoral zone of lake Szeląg Wielki in the Iława Lake District threatened by pesticide tomb. Fresenius Environmental Bulletin. 14(5):357–362.
- Hakanson L, Boulion VV. 2002. Empirical and dynamical models to predict the cover, biomass and production of macrophytes in lakes. Ecological Modelling. 151:213–243.
- Hillbricht-Ilkowska A. 1993. Phosphorus loading to lakes of Suwałki Landscape Park (north-eastern Poland) and its relation to lake trophy indices. Ekologia Polska. 41:221–235.
- Hillbricht-Ilkowska A, Wiśniewski RJ. 1993. Trophic differentiation of lakes of the Suwałki Landscape Park (north-eastern Poland) and its buffer zone – present state, changes over years, position in trophic classification of lakes. Ekologia Polska. 41:195–219.
- Hosper SH. 1998. Stable states, buffers and switches: an ecosystem approach to the restoration and management of shallow lakes in the Netherlands. Water Science and Technology. 37:151–164.
- Hotelling H, 1933. Analysis of a complex of statistical variables into principal components. Journal of Educational Psychology. 24:417–441.
- Hoyer MV, Canfield DE Jr. 1996. Largemouth bass abundance and aquatic vegetation in Florida lakes: an empirical analysis. Journal of Aquatic Plant Management. 34:23–32.
- Jeffries MJ. 1998. Pond macrophyte assemblages, biodisparity and spatial distribution of ponds in the Northumberland Coastal Plain, UK. Aquatic Conservation: Marine and Freshwater Ecosystem. 8:657–667.
- Jeppesen E, Søndergaard M, Søndergaard M, Christoffersen K. 1998. The structuring role of submerged macrophytes in lakes. New York: Springer.
- Karr JR. 1991. Biological integrity: a long-neglected aspect of water resource management. Ecological Applications. 1:66–84.
- KCS. 2007. Multivariate statistical package (MVSP) ver. 3.1. Pentraeth, Wales (England): Kovach Computing Services.
- Kolada A. 2009. The evaluation of biocenotic typology of Polish lakes using macrophyte community structure. Verhandlungen Internationale Vereinigung für Theoretische und Angewandte Limnologie. 30/5:775–778.
- Kolada A. 2010. The use of aquatic vegetation in lake assessment: testing the sensitivity of macrophyte metrics to anthropogenic pressures and water quality. Hydrobiologia. 656:133–147.
- Kowalczewska-Madura K, Gołdyn R. 2012. Spatial and seasonal variability of pore water phosphorus concentration in shallow Lake Swarzêdzkie, Poland. Environmental Monitoring and Assessment. 184(3):1509–1516.
- Kufel L. 1998. Chlorophyll–nutrients–Secchi disc relationships in the Great Masurian Lakes (north-eastern Poland). Polish Journal of Ecology. 46:327–337.
- Kufel L. 1999. Dimictic versus polymictic Masurian lakes: similarities and differences in chlorophyll–nutrients–SD relationships. Hydrobiologia. 408/409 (Dev. Hydrobiol. 143):389–394.
- Kufel L. 2001. Uncoupling of chlorophyll and nutrients in lakes – possible reasons, expected consequences. Hydrobiologia. 443:59–67.
- Kufel L, Kufel I. 2002. Chara beds acting as nutrient sinks in shallow lakes – a review. Aquatic Botany. 72:249–260.
- Lacoul P, Freedman B. 2006. Environmental influences on aquatic plants in freshwater ecosystems. Environmental Reviews. 14:89–136.
- Lepš J, Šmilauer P. 2007. Multivariate analysis of ecological data using CANOCO. Cambridge (England): Cambridge University Press.
- Lind OT. 1986. The effect of non-algal turbidity on the relationship of Secchi depth to chlorophyll a. Hydrobiologia. 140:27–35.
- Linton S, Goulder R. 2000. Botanical conservation value related to origin and management of ponds. Aquatic Conservation: Marine and Freshwater Ecosystem. 10:77–91.
- Magurran A. 1988. Ecological diversity and its measurement. Princeton (NJ): Princeton University Press.
- Matuszkiewicz W. 2002. A guide book to identification plant communities in Poland. Warsaw: PWN. Polish.
- McCauley E, Downing JA, Watson S. 1989. Sigmoid relationships between nutrients and chlorophyll among lakes. Canadian Journal of Fisheries and Aquatic Sciences. 46:1171–1175.
- McClanahan T, Polunin N, Done T. 2002. Ecological states and the resilience of coral reefs. Conservation Ecology. 6:18.
- Megard RO, Settles JC, Boyer HA, Combs WS Jr. 1980. Light, Secchi disks and trophic states. Limnology and Oceanography. 25:373–377.
- Møller TR, Rørdam CP. 1985. Species numbers of vascular plants in relation to area, isolation and age of ponds in Denmark. Oikos. 45:8–16.
- Murphy KJ. 2002. Plant communities and plant diversity in softwater lakes of northern Europe. Aquatic Botany. 73:287–324.
- Nixdorf B, Daneke R. 1997. Why ‘very shallow’ lakes are more successful opposing reduced nutrient loads. Hydrobiologia. 342/343:269–284.
- Oertli B, Joey DA, Castella E, Juge R, Cambin D, Lachavanne JB. 2002. Does size matter? The relationship between pond area and biodiversity. Biological Conservation. 104:59–70.
- Otsuki A, Wetzel MM. 1974. Calcium and total alkalnity budget and calcium carbonate precipitation of a small hardwater lake. Archiv Für Hydrobiologie. 73:14–30.
- Penning E, Dudley B, Mjelde M, Hellsten S, Hanganu J, Kolada A, Van Den Berg M, Poikane S, Phillips G, Willby N, et al. 2008a. Using aquatic macrophyte community indices to define the ecological status of European lakes. Aquatic Ecology. 42:253–264.
- Penning E, Mjelde M, Dudley B, Hellsten S, Hanganu J, Kolada A, Van Den Berg M, Mäemets H, Poikane S, Phillips G, et al. 2008b. Classifying aquatic macrophytes as indicators of eutrophication in European lakes. Aquatic Ecology. 42:237–251.
- Pridmore RD, Hewitt JE, Cooper AB. 1989. Does the chlorophyll a content of phytoplankton vary with trophic status in lakes on the New Zealand central volcanic plateau?. Journal of Plankton Research. 11:583–593.
- Rørslett B. 1991. Principal determinants of aquatic macrophyte richness in northern European lakes. Aquatic Botany. 39:173–193.
- Rounsefell GA. 1946. Fish production in lakes as a guide for estimating production in proposed reservoirs. Copeia. 1:29–40.
- Sakamoto M. 1966. Primary production by phytoplankton community in some Japanese lakes and its dependence on lake depth. Archiv Für Hydrobiologie. 62:1–28.
- Scheffer M. 1998. Ecology of shallow lakes. London (England): Chapman & Hall.
- Scheffer M, Carpenter S, Foley JA, Folke C, Walker B. 2001. Catastrophic shifts in ecosystems. Nature. 413:591–596.
- Scheffer M, Hosper SH, Meijer ML, Moss B, Jeppesen E. 1993. Alternative equilibria in shallow lakes. Trends in Ecology & Evolution. 8:275–279.
- Scheffer M, van Nes EH. 2007. Shallow lakes theory revisited: various alternative regimes driven by climate, nutrients, depth and lake size. Hydrobiologia. 584:455–466.
- Søndergaard M, Jeppesen E, Jensen JP, Amsinck SL. 2005. Water Framework Directive: ecological classification of Danish lakes. Journal of Applied Ecology. 42:616–629.
- Søndergaard M, Johansson L, Lauridsen T, Jorgensen T, Liboriussen L, Jeppesen E. 2010. Submerged macrophytes as indicators of the ecological quality of lakes. Freshwater Biology. 55:893–908.
- Spence DHN. 1967. Factors controlling the distribution of freshwater macrophytes with particular reference to Scottish Lochs. Journal of Ecology. 55:147–170.
- SDF (Standard Data Form) PLH280016 Ostoja Borecka [Internet]. 2008. [ cited 2013 May 17]. Available from: http://natura2000.eea.europa.eu/natura2000/SDF.aspx?site=PLH280016
- Standard Methods for Examination of Water and Wastewater. 1999. American Public Health Association, American Water Works Association, Water Environment Federation, New York.
- StatSoft, Inc. 2011. STATISTICA (data analysis software system), version 10. Available from: http://www.statsoft.com
- Szmeja J. 2000. Tendencies of changes in the flora and vegetation structure of Pomeranian lakes under the influence of humic substances. In: Jackowiak B, Żukowski W, editors. Mechanisms of anthropogenic changes of the plant cover. Poznań (Poland): Bogucki Wydawnictwo Naukowe. p. 85–98.
- Ter Braak CJF, Šmilauer P. 2002. CANOCO reference manual and CanoDraw for windows user's guide: software for canonical community ordination (version 4.5). New York: Microcomputer Power.
- Tilzer MM, Serruya C. 1990. Large lakes, ecological structure and function. Heidelberg (Germany): Springer Verlag.
- Toivonen H, Huttunen P. 1995. Aquatic macrophytes and ecological gradients in 57 small lakes in southern Finland. Aquatic Botany. 51:197–221.
- Weber HE, Moravec J, Theurillat J-P. 2000. International code of phytosociological nomenclature. Journal of Vegetation Science (3rd Ed.). 1:739–768.
- Wetzel RG. 2001. Limnology: lake and river ecosystems. San Diego: Academic Press.
- Wilkinson KJ, Negre JC. 1997. Coagulation of colloidal material in surface waters: the role of natural organic mater. Journal of Contaminant Hydrology. 26:229–243.
- Wright JF. 1995. Development and use of a system for predicting macroinvertebrates in flowing waters. Australian Journal of Ecology. 20:181–197.
- Wright JF, Sutcliffe DW, Furse MT. 2000. Assessing the biological quality of freshwaters: RIVPACS and similar techniques. Ambleside (England): Freshwater Biological Association.
- Van den Berg MS. 1999. Charophyte colonization in shallow lakes: processes, ecological effects and implications for lake management [PhD thesis]. [Amsterdam (The Netherlands)]: Free University.
- Van den Berg MS, Coops H, Meijer ML, Scheffer M, Simons J. 1998. Clear water associated with a dense Chara vegetation in the shallow and turbid Lake Veluwemeer, The Netherlands. In: Jeppesen EM, Søndergaard M, Søndergaard M, Christoffersen K, editors. The structuring role of submerged macrophytes in lakes. New York: Springer. p. 339–352.
- Van den Berg MS, Joosse W, Coops H. 2003. A statistical model predicting the occurrence and dynamics of submerged macrophytes in shallow lakes in the Netherlands. Hydrobiologia. 506–509:611–623.
- Van den Wollenberg AL. 1977. Redundancy analysis – an alternative for canonical correlation analysis. Psychometrika. 42:207–219.
- Zdanowski B. 1982. Variability of nitrogen and phosphorus contents and lake eutrophication. Polskie Archiwum Hydrobiologii. 29(3–4):541–597.